Applications of Fluorescence Microscopy in Research
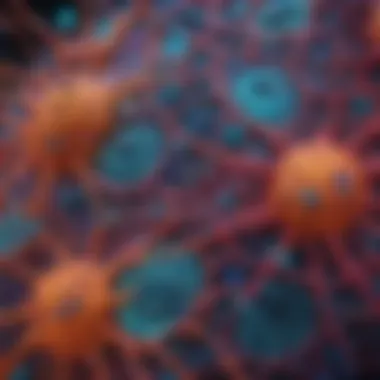
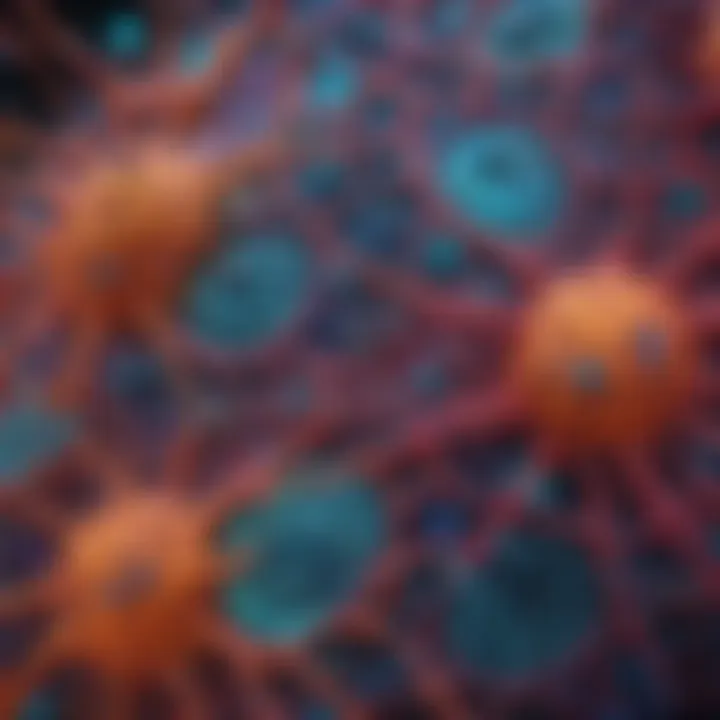
Intro
In the following sections, we will explore how fluorescence microscopy is used in different domains such as cellular biology, neuroscience, immunology, and material sciences. Each application reveals the strengths of this imaging technique and its impact on research.
Research Highlights
Overview of Key Findings
Fluorescence microscopy has been instrumental in revealing critical insights into cell dynamics, protein interactions, and disease mechanisms. Key findings include:
- Cellular Interactions: Researchers have utilized fluorescence to observe live cell interactions in real time, providing insights into processes such as cell signaling and migration.
- Neural Pathways: In neuroscience, fluorescence microscopy has illuminated how neurons connect and communicate, helping to map neural circuits with great precision.
- Disease Research: In medical research, fluorescence techniques have been applied to study the pathogenesis of diseases like cancer and Alzheimer's, identifying the roles of specific proteins in disease progression.
Significance of the Research
The significance of fluorescence microscopy extends beyond mere observation. It has revolutionized our understanding of complex biological phenomena. Recent advancements enable researchers to achieve greater specificity and resolution. Techniques like super-resolution microscopy push the boundaries of traditional light microscopy, allowing for the visualization of structures previously thought to be too small to observe.
"Fluorescence microscopy is not just a tool; it is a gateway to understanding life at a cellular level."
Original Research Articles
Summary of the Article
Recent research articles have demonstrated the versatility of fluorescence microscopy in various experimental settings. For example, studies on live cell imaging have shown the effective tracking of cellular components in their natural environment. Another notable contribution is the application of fluorescence in drug discovery, aiding in assessing drug efficacy and cellular responses.
Author Contributions
Contributions from multiple authors have enriched the field. For instance, researchers have developed new fluorescent probes and improved imaging protocols. Others have focused on data analysis techniques, helping to interpret complex fluorescent signal patterns. Such collaborative efforts enhance the overall understanding of biological processes.
This exploratory discussion on fluorescence microscopy sets the stage for the deeper investigation into its applications in diverse scientific fields, which follows in later sections.
Preamble to Fluorescence Microscopy
Fluorescence microscopy plays a crucial role in modern scientific research. As a powerful imaging technique, it allows for the visualization of various biological and material samples at a cellular level. Researchers can observe phenomena in real-time, leading to deeper insights into cellular processes and material behaviors. The necessity for clear imaging techniques becomes compelling in disciplines like cellular biology, neuroscience, and immunology. The efficacy of fluorescence microscopy lies in its ability to label specific targets, allowing scientists to study complex interactions within the cell or material being examined.
Understanding the foundational elements of fluorescence is important. This technique relies on the principle of fluorescence, where certain compounds absorb light at specific wavelengths and then re-emit it at longer wavelengths. This property is particularly advantageous in distinguishing different structures within a sample. Moreover, the incorporation of fluorescent dyes and proteins, such as green fluorescent protein (GFP), has revolutionized the capacity to mark biological structures.
Although fluorescence microscopy provides unparalleled insights, it is not without challenges. Researchers must consider issues related to photobleaching, where prolonged exposure to light causes fluorescent compounds to lose their ability to emit light. Furthermore, achieving a high signal-to-noise ratio is essential for obtaining clear images. Addressing these considerations can significantly enhance the quality of data collected during experiments.
Overview of Fluorescence Principles
Fluorescence microscopy relies on the fundamental principles of fluorescence. When a fluorophore absorbs light energy, it gets excited to a higher energy state. Subsequently, as the fluorophore returns to its ground state, it emits light at a longer wavelength. This process allows researchers to observe specific molecules within cells or materials based on their unique fluorescent signatures.
The effectiveness of fluorescence microscopy comes from the various types of fluorophores available, each with distinct absorption and emission characteristics. Selection of the right fluorophore is critical for optimizing imaging outcomes. Additionally, advanced filters and detection systems are implemented to enhance the quality of captured images, minimizing background noise and ensuring clarity in visualization.
Historical Context and Development
The development of fluorescence microscopy spans several decades. Early experimentation with fluorescence concentrated on basic principles without advanced imaging capabilities. With technological advancements, fluorescence microscopy evolved significantly. The introduction of specific fluorescent dyes enabled more precise imaging of cellular components.
In the 20th century, major improvements began to emerge, particularly with the development of confocal and multiphoton microscopy. These techniques offered enhanced resolution and depth of field. Sophisticated image processing software further transformed the landscape of fluorescence microscopy, allowing for the reconstruction of three-dimensional images from multiple two-dimensional slices.
"The advancement in imaging technologies has propelled fluorescence microscopy into a new era, providing unprecedented details of biological systems."
The interplay of chemistry and optics facilitated major breakthroughs, making fluorescence microscopy a standard tool in laboratories worldwide. Today, it remains an essential approach for life sciences research, paving the way for groundbreaking discoveries in various scientific fields.
Fundamental Techniques in Fluorescence Microscopy
Basic Setup and Configuration
The setup of a fluorescence microscope fundamentally influences its performance and image quality. A typical system contains a light source, filters, and an imaging system such as a camera or eyepiece. The light source is often a high-intensity lamp or laser, providing the necessary excitation for fluorescent dyes. Accurate filtration of light is crucial; excitation filters allow only specific wavelengths to illuminate the sample, while emission filters ensure that only light emitted by the fluorophores reaches the detector. Adjusting these components influences resolution and contrast. Depending on the study's objectives, researchers may need to customize the configuration to obtain the best results.
Types of Fluorescence Microscopes
Different types of fluorescence microscopes accommodate varying research needs, each with distinctive advantages and disadvantages.
Wide-field Microscopy
Wide-field microscopy is among the simplest forms of fluorescence microscopy. Its primary advantage is the ability to quickly capture images of a sample across a large area in one shot. This is particularly beneficial for applications that demand a broad view of cellular structures. The key characteristic is its use of wide-field illumination, which results in images of significant brightness. However, this technique can suffer from issues like out-of-focus light contamination, limiting resolution in thicker samples. Despite this limitation, wide-field microscopy remains a popular choice due to its simplicity and ease of use.
Confocal Microscopy
Confocal microscopy brings a more refined approach, allowing for higher resolution and improved depth of field. One critical feature is its ability to collect images from a single plane at a time, effectively rejecting out-of-focus light. This results in clearer images of samples, especially thicker specimens. The use of lasers as the light source enhances the ability to focus tightly on specific areas, making it a favored method in many research contexts. The strength of this method lies in its detailed imaging capability, although it requires more extensive setup and can be time-consuming.
Multiphoton Microscopy
Multiphoton microscopy is a more advanced technique that further enhances imaging depth. It utilizes two or more photons to excite fluorophores, allowing for deeper tissue penetration without damaging the sample. A notable feature is its reduced phototoxicity, making it highly suitable for imaging live cells over extended periods. The main advantage is the ability to visualize samples in their natural state, providing insights into dynamic cellular processes. However, this method can be expensive and may require specialized training for optimal usage.
Applications in Cellular Biology
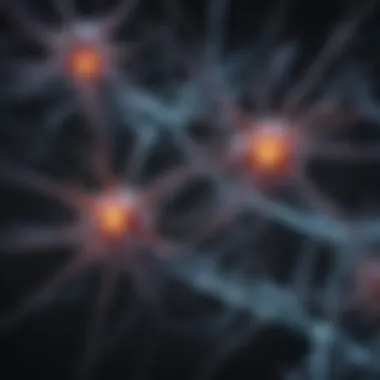
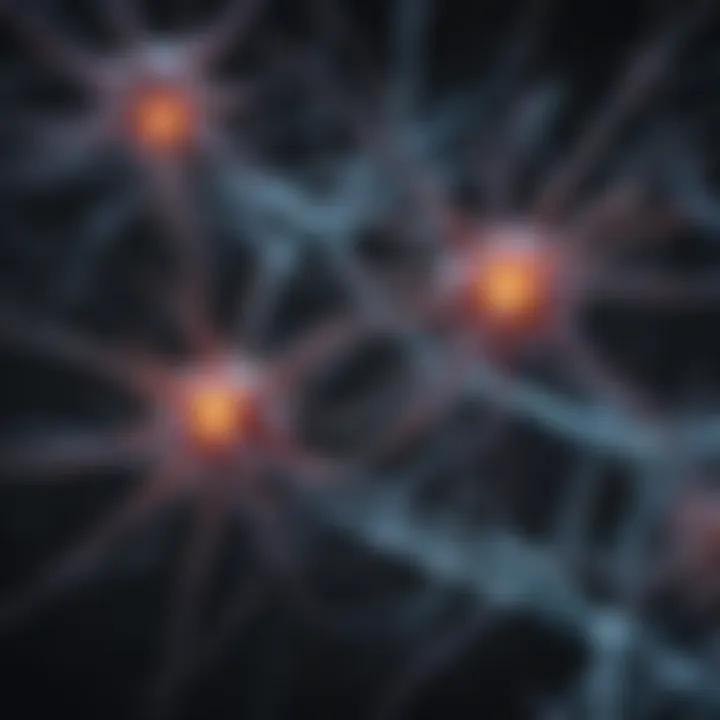
Fluorescence microscopy plays a crucial role in cellular biology. It allows scientists to visualize and analyze complex cellular structures and processes in real time. The technique offers unique advantages that contribute significantly to our understanding of cellular dynamics. By employing fluorescent labels, researchers can specifically tag various components within cells. This capability enhances the specificity of observations, leading to better insights into cell behavior and function. Moreover, understanding cellular processes is essential not only in basic research but also in medical and environmental sciences.
Cell Imaging and Analysis
Cell imaging and analysis using fluorescence microscopy has revolutionized how we observe biological samples. With this technique, high-resolution images can be captured, revealing detailed structures within cells. This is important for identifying various organelles, such as mitochondria and nuclei, as well as understanding their functions.
Key benefits of cell imaging include:
- Real-time observation: Researchers can monitor cellular changes as they occur, providing insights into dynamic processes like cell division or migration.
- Specificity: By using different fluorescent labels, researchers can target specific proteins or structures, making it easier to study their roles and interactions within the cell.
- Quantitative analysis: Advanced imaging allows for detailed quantification of cellular components, contributing to a deeper understanding of cellular biology.
However, challenges such as photobleaching and signal overlap must be addressed. These issues can hinder accurate analysis and observation, making careful experimental design critical.
Tracing Cellular Processes
Tracing cellular processes is another vital application of fluorescence microscopy. This is particularly important for tracking interactions between cellular components and observing functional processes.
Fluorescence microscopy allows for:
- Live-cell imaging: This method enables scientists to watch processes like protein trafficking or apoptosis in living cells. Understanding these processes is key for insights into cell health, development, and disease.
- Dynamic studies: Researchers can examine how cells respond to external stimuli, such as drugs or environmental changes. This helps in identifying pathways involved in disease states and treatments.
Furthermore, the ability to perform time-lapse imaging adds a temporal component that enhances the understanding of cellular processes over time. For example, observing how a population of cells migrates in response to a wound can inform on healing mechanisms.
Fluorescence Microscopy in Neuroscience
Fluorescence microscopy has significantly advanced the field of neuroscience by providing detailed insights into the workings of the nervous system. The ability to visualize live neurons and their interactions in real-time is crucial for understanding various neurological processes. Techniques like this allow scientists to monitor both neuronal structure and function under physiological conditions. This understanding can lead to improvements in treating neurological disorders and enhancing our knowledge of brain health.
Neuronal Activity Mapping
Neuronal activity mapping is an essential application of fluorescence microscopy in neuroscience. This technique focuses on tracking the activity of neurons in response to stimuli or during specific tasks. By using genetically encoded calcium indicators, researchers can visualize calcium ion concentration changes in neurons. This provides a direct way to infer neuronal activity.
For example, the GCaMP family of genetically encoded calcium indicators can be introduced into neurons via viral vectors. When neurons fire, calcium ions influx, causing the GCaMP molecules to fluoresce. This allows real-time recording of neuronal activity. The data gathered from these experiments offers a precise map of how neurons interact and synchronize with one another during different states of activity.
Key points regarding neuronal activity mapping include:
- Temporal Resolution: The ability to capture fast changes in neuronal activity.
- Quantitative Analysis: Providing numerical data about neuron firing rates, allowing for a thorough understanding of neuronal behavior.
- Non-invasiveness: Enabling observations in living animals, contributing to more naturalistic data collection.
Neuronal activity mapping is not just beneficial for basic research; it is also critical for developing new therapeutic strategies in brain disorders.
Studying Synaptic Dynamics
Studying synaptic dynamics is another critical application of fluorescence microscopy in neuroscience. Synapses play an indispensable role in communication between neurons. By observing how synapses form, change, and destabilize during different conditions, researchers gain insights into both healthy and diseased states of the brain.
Fluorescence microscopy can be particularly effective for studying synaptic dynamics by employing techniques such as photo-activated localization microscopy (PALM) or stimulated emission depletion microscopy (STED). These methods achieve high resolution, enabling researchers to visualize synapse activity at the molecular level.
Research has shown that certain neurodegenerative diseases, such as Alzheimer’s, involve synaptic dysfunction. By using fluorescence microscopy to study these changes, scientists can observe the early stages of synaptic failure. This understanding allows for the identification of potential targets for therapeutic interventions.
Important aspects of studying synaptic dynamics include:
- Molecular Imaging: Use of fluorescent markers to visualize specific proteins involved in synaptic transmission.
- Dynamic Monitoring: Tracking real-time changes in synaptic structure and function as neuronal activity fluctuates.
- Impact on Learning and Memory: Assessing how synaptic changes correlate with behavioral outcomes, linking molecular events to cognitive functions.
Role in Immunology Research
Fluorescence microscopy plays a pivotal role in immunology research, significantly enhancing our understanding of immune responses and disease mechanisms. This technique allows for high-resolution imaging of cells and tissues, providing insights into the complex interactions of immune cells. Utilizing fluorescent markers can easily visualize various components within the immune system. This leads to better diagnostics and innovative therapeutic approaches.
Understanding Immune Responses
The immune system is intricate and dynamic, and observing it in real-time can be quite challenging. Fluorescence microscopy enables researchers to track immune cells’ movements and behaviors. For instance, researchers can label T cells with specific fluorescent dyes to visualize their activation and interaction with antigen-presenting cells. This real-time tracking of immune responses is essential for understanding infections, autoimmune diseases, and cancer immunology.
Studies have shown how fluorescence microscopy can reveal how dendritic cells activate T cells. Not only does it help in discovering the cellular mechanisms but also enhances the understanding of how these processes alter in disease states. Here, specific applications include:
- Tracking the migration patterns of immune cells in tissues during inflammatory responses.
- Studying the activation and signaling pathways of immune cells at a molecular level.
- Investigating interactions between different cell types, such as helper T cells and B cells.
"Fluorescence microscopy paves the way for more precise interventions in immunological disorders through visualizing real-time cellular interactions."
Antibody Labeling Techniques
Antibody labeling techniques are integral to the effectiveness of fluorescence microscopy in immunology. By conjugating fluorescent dyes to antibodies, researchers can specifically target proteins and other molecules of interest. This allows for clear visualization of antigens within complex samples.
Popular techniques include:
- Immunofluorescence: This method uses antibodies labeled with fluorescent dyes to stain specific proteins within cells or tissues. It is valuable in localizing antigens in fixed tissues or live cells.
- Flow Cytometry: Although not traditional microscopy, this technique applies fluorescent labeling to analyze the characteristics of cells in a fluid stream. It enables simultaneous detection of multiple markers on individual cells.
- Fluorescent in situ hybridization (FISH): This technique combines fluorescence microscopy with genetic studies to allow imaging of specific DNA or RNA sequences.
Each of these techniques provides unique insights into cellular behaviors and interactions within the immune system. Therefore, the role of fluorescence microscopy in immunology cannot be overstated. The advancements in antibody labeling and imaging techniques have opened new pathways for research, deepening our understanding of immune responses and their implications in health and disease.
Advancements in Imaging Technology
The field of fluorescence microscopy has witnessed tremendous advancements in imaging technology. These developments have significantly enhanced the ability to visualize biological structures and processes. This section details key innovations such as super-resolution microscopy and time-lapse imaging, highlighting their impact and relevance in modern scientific research.
Super-Resolution Microscopy
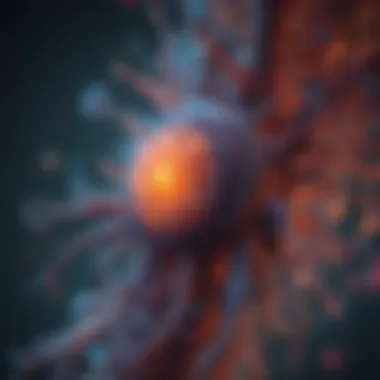
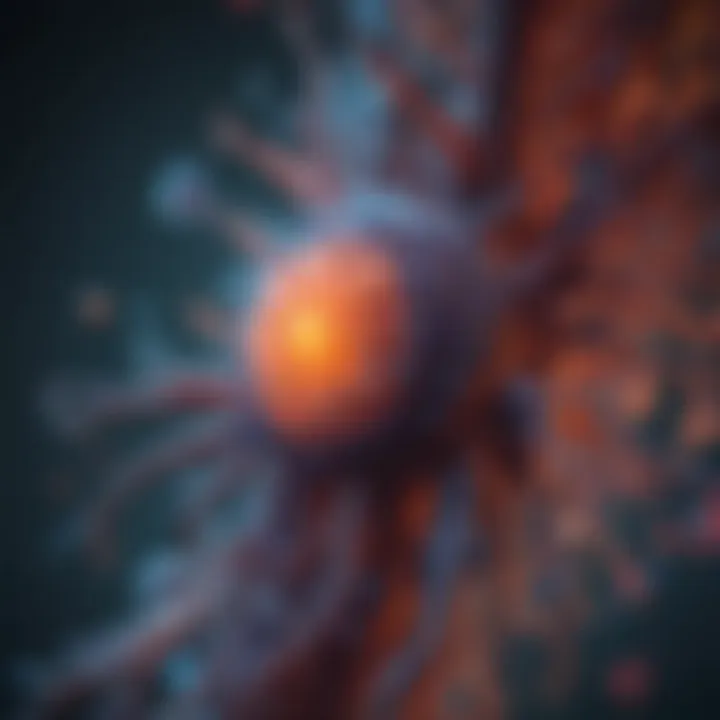
Super-resolution microscopy represents a leap forward in the resolution limit of light microscopy. Traditional fluorescence microscopy is often restricted to a resolution of about 200 nanometers due to the diffraction limit of light. Super-resolution techniques, such as STED (Stimulated Emission Depletion) microscopy and PALM (Photo-Activated Localization Microscopy), allow researchers to surpass this limit, achieving resolutions in the range of tens of nanometers.
This increased resolution has far-reaching implications. For instance, in cellular biology, researchers can observe the arrangement of proteins within small cellular compartments, providing insights into fundamental biological processes. It allows scientists to explore molecular interactions with unprecedented clarity, shedding light on intricate cellular mechanisms. The potential for super-resolution imaging is not just limited to fundamental research; it also plays a crucial role in drug discovery, as enhanced detailed imaging aids in identifying therapeutic targets.
Moreover, as these techniques evolve, they also improve accessibility and usability. Newer systems integrate super-resolution modalities with existing fluorescence microscopy setups. This integration allows broader adoption across various research environments, expanding the versatility of fluorescence microscopy in scientific exploration.
Time-Lapse and Live-Cell Imaging
Time-lapse and live-cell imaging techniques are essential for studying dynamic biological processes in real-time. Fluorescence microscopy has become a key tool for observing live cells under various conditions, enabling researchers to track cellular changes over time.
Time-lapse imaging involves capturing a series of images at set intervals, allowing for the observation of processes such as cell division, migration, or morphology changes. This method helps parse complex biological interactions in situ, giving invaluable insight into cellular behavior. For example, researchers can visualize the movement of vesicles inside a cell, providing evidence for cellular communication pathways or the cellular response to external stimuli.
Live-cell imaging, a subset of time-lapse techniques, focuses specifically on observing living cells without compromising their viability. Using advanced fluorescent tags and careful imaging protocols, it allows for the monitoring of live cellular events while preserving the functionality of the cells. This approach is particularly valuable in drug response studies, where knowing a compound's effect on live tissue can lead to more informed hypotheses about efficacy and mechanisms of action.
Fluorescence Microscopy in Material Sciences
Fluorescence microscopy plays a crucial role in material sciences due to its ability to provide detailed insights into the structure and properties of various materials. By leveraging fluorescence, researchers can visualize components at the nanoscale, analyze properties such as morphology and distribution, and monitor interactions in real-time. The unique capabilities of fluorescence microscopy not only enhance understanding but also facilitate innovation in material development. Its applications span across nanomaterials characterization and polymer studies.
Characterization of Nanomaterials
Nanomaterials possess distinctive properties that arise from their small size and high surface area. Fluorescence microscopy has become invaluable for characterizing these nanomaterials. It allows for the visualization of nanoparticles, providing crucial information regarding size, shape, and surface modifications. This technique can help assess the distribution of nanoparticles in different environments, which is essential in fields like electronics and medicine.
Using fluorescence, scientists can perform imaging of nanomaterials without the need for extensive sample preparation. For instance, Quantum dots and other fluorescent nanoparticles can be tracked within biological systems, ensuring that their interaction and movement can be monitored with precision. Continuous observation during applications, such as drug delivery, gives insights into how these materials function in practical scenarios.
"Fluorescence microscopy enables a high-resolution view of nanomaterials, bridging gaps between their theoretical predictions and practical applications."
Fluorescence in Polymer Studies
In polymer studies, fluorescence microscopy assists in understanding the behavior and properties of complex polymer systems. Polymers can exhibit heterogeneous properties, and fluorescence techniques help visualize these differences at the microscopic level. For example, the distribution of additives and fillers within a polymer matrix can directly influence its mechanical properties. By using fluorescent labels, researchers can evaluate how well these components are mixed and whether they lead to intended material characteristics.
The use of photostable fluorescent dyes allows for prolonged observation, making it easier to study dynamic processes such as polymer degradation or phase separation. Specific applications include analyzing polymer blends or composites under varying thermal or chemical conditions.
Applications in Pharmaceutical Research
Fluorescence microscopy plays a crucial role in pharmaceutical research by providing detailed insights into drug interactions, efficacy, and safety. Understanding the behavior of drugs at the cellular level is essential for both development and testing phases. The applications of this microscopy technique encompass various aspects of drug discovery and toxicity assessment, allowing researchers to visualize and analyze biological processes with high sensitivity and resolution.
Drug Development and Testing
In the realm of drug development, fluorescence microscopy offers significant benefits. Researchers can observe cellular responses to new compounds in real-time. This real-time observation aids in identifying how drugs interact with target cells, such as cancer cells or pathogens. Here are some key points regarding its application in drug development:
- High Sensitivity: Fluorescent markers allow for the detection of drugs at lower concentrations than traditional methods.
- Spatial Resolution: This technique enables scientists to pinpoint the location of drugs within cellular structures. This is vital for understanding distribution and accumulation.
- Temporal Resolution: Monitoring dynamic processes over time provides insights into the pharmacodynamics of a drug.
For instance, employing fluorescently labeled compounds can illustrate how a drug enters a cell and subsequently localizes within specific organelles. This information can inform adjustments to chemical structures for improved efficacy and reduced side effects.
Toxicology Studies
Toxicology is another area where fluorescence microscopy shines. It enables researchers to assess the safety of new compounds effectively. Understanding the potential toxic effects of drugs before they reach clinical trials is crucial. Here’s how fluorescence microscopy facilitates toxicological assessments:
- Cell Viability: By using fluorescent stains, scientists can quickly assess whether cells survive after drug administration. Live/Dead assays are commonly implemented to distinguish between viable and non-viable cells.
- Mechanism of Toxicity: The technique allows for detailed observation of cellular events like apoptosis or necrosis. By studying these processes, researchers gain insights into the molecular pathways involved in toxicity.
- Biomarker Visualization: Certain fluorescent probes can help visualize specific biomarkers related to toxicity, offering an understanding of how a compound affects cellular health.
"Fluorescence microscopy enables a nuanced view of drug interactions and cellular responses, crucial for ensuring safety and efficacy in drug development."
Challenges and Limitations
The application of fluorescence microscopy, while impactful, comes with its set of challenges and limitations that researchers must navigate. Understanding these issues is critical for improving imaging techniques and obtaining reliable results. Addressing challenges such as photobleaching and the signal-to-noise ratio directly influences the quality of the data produced in various scientific studies. This section will delve into these problems, outlining their implications for research and suggesting strategies to mitigate their effects.
Photobleaching Issues
Photobleaching refers to the irreversible loss of fluorescence from a fluorophore after exposure to light. This phenomenon poses a significant challenge in fluorescence microscopy, particularly during long imaging sessions or time-lapse experiments. When photobleaching occurs, the signals can become weaker, leading to underrepresentation of important cellular processes.
The extent of photobleaching can vary based on several factors:
- Type of Fluorophore: Some fluorophores are inherently more stable than others.
- Excitation Intensity: Higher light intensities can lead to increased rates of photobleaching.
- Environmental Conditions: Temperature and the presence of oxygen can affect photostability.
To manage photobleaching, researchers can employ several strategies. One common approach is using antifade reagents, which can help reduce the impact of photobleaching. Another technique involves using lower excitation intensities and optimizing imaging protocols to minimize exposure time. In some cases, selecting fluorophores with higher photostability can also improve outcomes.
Signal-to-Noise Ratio
The signal-to-noise ratio (SNR) is a crucial metric in fluorescence microscopy. It describes the level of desired signal compared to the level of background noise. A high SNR is essential for obtaining clear images and reliable data. Poor SNR can lead to misinterpretation of results, as noise can obscure real signals or create false positives.
Several factors can influence SNR in fluorescence microscopy:
- Fluorophore Concentration: Higher concentrations can enhance signal strength but also increase background noise.
- Environmental Conditions: Factors like scattering and fluorescence from the sample itself can affect SNR.
- Detection System Quality: Superior optical components and detectors can significantly improve SNR.
To improve the signal-to-noise ratio, researchers can use several techniques. One effective method is to optimize the imaging setup, adjusting settings to enhance signal capture while reducing noise. Additionally, ensuring proper sample preparation and employing advanced signal processing algorithms can help improve SNR.
The challenges of photobleaching and low signal-to-noise ratio are critical concerns that should not be overlooked. Addressing these issues enhances the reliability and precision of fluorescence microscopy results.
By recognizing and addressing these challenges, researchers can enhance the efficacy of fluorescence microscopy, enabling better insights in cellular biology, neuroscience, and beyond.
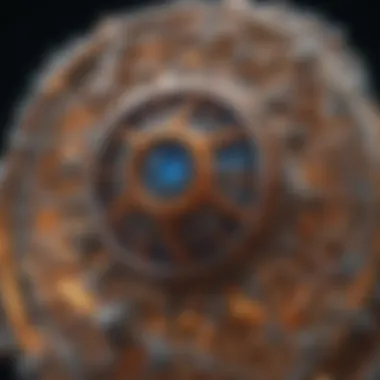
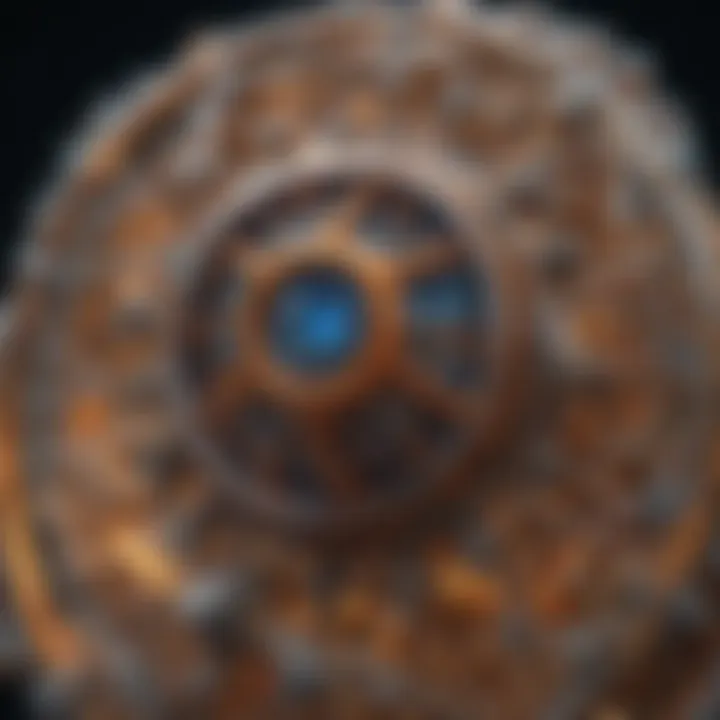
Ethical Considerations in Research
Ethical considerations in research using fluorescence microscopy are critical. As this technique becomes more integrated within various scientific fields, it is essential to recognize the implications of its application. Researchers face numerous ethical dilemmas, particularly regarding the use and disposal of fluorescent dyes. Understanding these dilemmas is crucial to maintain scientific integrity and public trust.
Responsible Use of Fluorescent Dyes
The responsible use of fluorescent dyes is paramount to ensure both effective research and environmental safety. These dyes can be toxic to cells and may have harmful side effects. Thus, researchers must carefully select the right fluorescent dye. This choice often dictates the outcome of the experiment.
When choosing fluorescent dyes, it is essential to follow established guidelines to minimize potential adverse effects. Here are some important considerations:
- Selection of Non-Toxic Dyes: Favor dyes that have lower toxicity profiles. For example, dyes like GFP (Green Fluorescent Protein) are often preferred for cellular studies.
- Dosage: Only use the minimum required concentration. This approach limits exposure risk for living organisms.
- Disposal Procedures: Proper disposal of used dyes is another critical aspect. Always follow institutional protocols to prevent contamination.
Overall, ethical use of fluorescent dyes serves not only to enhance research outcomes but also to protect biological systems from unnecessary harm.
Data Transparency and Reproducibility
Data transparency and reproducibility are essential in all scientific research, and fluorescence microscopy is no exception. Providing access to data fosters trust between researchers and the community at large. Access to well-documented methodologies enables other scientists to repeat experiments and verify results.
Important aspects include:
- Clear Documentation: Researchers should document all procedures, including the specifics of fluorescent dye usage, imaging settings, and analysis methods. This detailed record allows others to replicate studies accurately.
- Open Data Practices: Where feasible, sharing data sets publicly can enhance the reproducibility of findings. Utilizing platforms such as figshare or the Open Science Framework is encouraged.
- Peer Review and Collaboration: Engaging in peer review and collaborative efforts can also improve the quality of research, as external insights can lead to higher standards of transparency.
Future Directions in Fluorescence Microscopy
Fluorescence microscopy continues to evolve, markedly improving its function and potential applications in various fields of scientific inquiry. The future of this technology is promising, driven by innovation and cross-disciplinary collaboration. As we advance into this new era, it is essential to recognize how these future directions can significantly enhance research capabilities and outcomes.
Integration with Other Technologies
One of the central elements shaping the future of fluorescence microscopy is the integration with emerging technologies. Combining fluorescence microscopy with techniques such as cryo-electron microscopy (cryo-EM) can yield superior imaging of biological samples. This pairing allows scientists to grasp both the structural and functional characteristics of cellular environments, which paves the way for more complete biological understanding.
Moreover, the adoption of artificial intelligence in image analysis can optimize data processing in fluorescence microscopy. AI can aid in identifying patterns, quantifying fluorescence intensity, and classifying outcomes—tasks that can be tremendously laborious for researchers. As machine learning tools improve, the efficiency and accuracy of analyses will likely see substantial enhancement.
Another promising direction is the integration of fluorescence microscopy with multiplex imaging techniques. By examining multiple targets within a single sample through spectral imaging or spatial multiplexing, researchers can achieve a more holistic view of complex biological systems. This will be essential in understanding intricate cellular mechanisms and interactions in real-time.
Potential for Clinical Applications
Other applications are seen in imaging techniques for neurodegenerative diseases or cardiovascular disorders. The ability to visualize cellular changes in real-time can provide significant insights into disease mechanisms and progression. This understanding may enhance the development of targeted therapies that can slow or halt diseases.
Additionally, the field of pathology is experiencing a shift with fluorescence microscopy. Compared to traditional histopathology, fluorescence techniques allow for better visualization of cellular anomalies. By employing fluorescent-conjugated antibodies, pathologists can achieve more detailed assessments of tissue samples, leading to improved diagnoses and treatment plans.
"The integration of fluorescence microscopy into clinical practice represents a significant leap toward more effective diagnostics and treatments."
Finale
The conclusion of this article emphasizes the significance of fluorescence microscopy in contemporary scientific research. It underscores how this technique has fundamentally changed our approach to observing biological systems at a molecular level. The ability to visualize cellular components in real-time offers researchers and educators invaluable insights into complex biological processes.
Summarizing Key Findings
Fluorescence microscopy has numerous applications across different fields. Key findings from this discussion include:
- The capacity for high-resolution imaging, which allows for detailed analysis of cellular structures and dynamics.
- The role of advanced methodologies, such as super-resolution and live-cell imaging, in enhancing our understanding of cellular behaviors.
- Fluorescence microscopy's significance in neuroscience for mapping neuronal activity and studying synaptic functions, which can lead to better understanding neurological disorders.
- Its applications in immunology, pivotal for observing immune responses and tracking antibody interactions.
- The contribution of fluorescence techniques in material science for characterizing nanomaterials and polymers, extending our comprehension of materials at the nanoscale.
Understanding these applications provides a foundation for future research opportunities. It also highlights the importance of integrating fluorescence microscopy with emerging technologies.
The Importance of Ongoing Research
Continued research in fluorescence microscopy is critical for several reasons. First, the field is rapidly advancing, and new techniques are consistently being developed that enhance imaging capabilities. Keeping pace with these advancements is vital for researchers to utilize the most effective methods in their work.
Finally, there is a strong need for ethical consideration in evolving research methods. As fluorescence microscopy becomes increasingly integral to scientific discovery, ensuring responsible use of fluorescent dyes and maintaining data transparency will be paramount. This focus on ethics shapes not only scientific practices but also the integrity of research outcomes.
As fluorescence microscopy advances, it will pave the way for innovative applications that can address the challenges faced in biology, healthcare, and materials science.
Importance of References in Research
References are not merely a list of citations; they are vital for several reasons:
- Validation of Claims: Each assertion made in a research article must be supported by previous findings. This ensures that any conclusions drawn are credible and based on established knowledge.
- Historical Context: References provide a historical narrative. They give insight into how fluorescence microscopy has evolved over time, tracing its applications across different fields such as biology and materials science.
- Resource for Further Investigation: They serve as a portal for researchers to expand knowledge. By following references, researchers can delve deeper into related studies that inform or challenge their findings.
Considerations for Effective Referencing
When dealing with references, several key elements must be observed:
- Accurate Citation: Every reference must be accurately cited. This includes all necessary details such as authors, publication years, and journals. Inaccurate citations can lead to misinformation.
- Diverse Sources: A broad spectrum of sources strengthens the integrity of the research. Combining seminal papers with recent studies offers a balance of foundational knowledge and current advancements.
- Accessible Literature: Researchers should ensure that cited works are accessible. Providing links or DOIs enables others to easily locate and review the original texts.
The Role of References in Fluorescence Microscopy
In the realm of fluorescence microscopy, references are especially significant. They allow for:
- Cross-Disciplinary Insights: Studies from diverse fields can provide valuable perspectives and methodologies that enhance fluorescence microscopy practices. This is particularly relevant when innovations emerge that incorporate multiple disciplines, such as material sciences and biology.
- Establishing Best Practices: By referencing established protocols and methodologies, researchers can develop best practices that reduce variability and increase reproducibility in experiments.
- Facilitating Technological Advancement: References highlight pivotal studies that have led to technological advancements in fluorescence microscopy. Understanding these developments can inspire new research questions and applications.
"The credibility of scientific research hinges on the integrity of its references. They transform isolated studies into a cohesive narrative."
Summation of the Role of References
In closing, the sections discussing fluorescence microscopy's applications reveal the complexity and significance of the field. However, without meticulously curated references, the impact and legitimacy of this research diminish. Thus, the commitment to rigorous referencing should remain a priority for anyone engaging in scientific writing and exploration.