Understanding the Critical Role of Beta NADPH
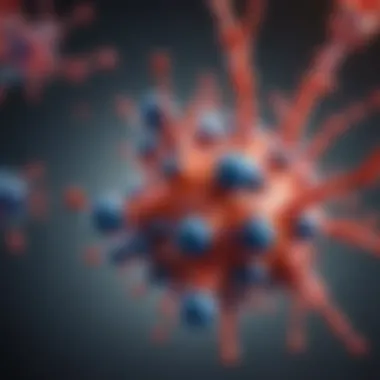
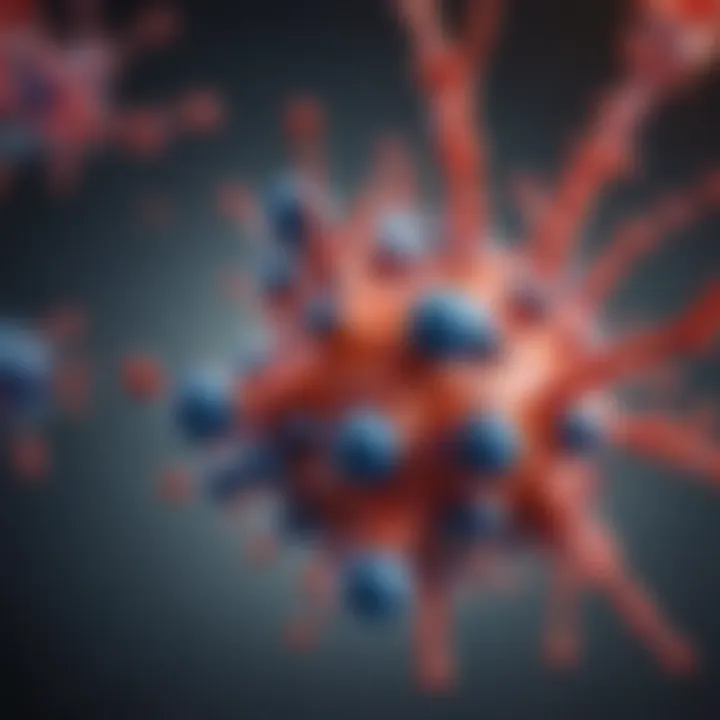
Intro
Beta NADPH is an essential coenzyme found widely in biological systems. It plays a pivotal role in numerous biochemical reactions, primarily those related to redox processes and biosynthetic pathways. Understanding its functionality and significance is crucial for several scientific disciplines, ranging from biochemistry to biotechnology.
The primary focus of this section is to highlight the key points surrounding beta NADPH, exploring its biochemical importance, metabolic roles, implications in health and disease, and potential applications in various fields, including medicine and environmental science. As we delve into this exploration, it is vital to recognize how beta NADPH acts not just as a mere cofactor but as a critical player in sustaining life at the cellular level.
Research Highlights
Overview of Key Findings
Beta NADPH is heavily involved in:
- Redox Reactions: It functions as a reducing agent in various biochemical pathways, helping maintain the balance of oxidative stress within cells.
- Biosynthetic Pathways: NADPH is vital for synthesizing fatty acids and cholesterol, playing an integral role in cellular metabolism.
- Antioxidant Defense: It aids in the regeneration of antioxidants, thereby promoting cellular health and longevity.
Significance of the Research
Research surrounding beta NADPH is key for several reasons:
- Understanding its role could lead to improved therapeutic strategies for diseases where oxidative stress is a major factor, such as cancer and cardiovascular diseases.
- Insights into its metabolic pathways may enable the development of biotechnological applications, including the engineering of microbial systems for enhanced production of biofuels or pharmaceuticals.
Original Research Articles
Summary of the Article
The exploration of beta NADPH demonstrates its multifaceted roles in living organisms. From supporting metabolic processes to its influence on human health, the research conducted offers valuable insights into its biochemical significance.
Author Contributions
Contributions to this field of study come from a variety of researchers and educators who specialize in biochemistry, molecular biology, and clinical research. Their collaborative efforts have enriched our understanding of beta NADPH, providing a clearer picture of its impact on cellular functionality and overall health.
Prelims to Beta NADPH
Beta NADPH, a pivotal coenzyme, plays an essential role in various biological systems. Its significance extends beyond mere participation in metabolic reactions; it is a fundamental component that drives cellular processes. Understanding beta NADPH can shed light on its relevance in phenomena such as cellular respiration, photosynthesis, and biosynthetic pathways. The intricate balance of its reduction and oxidation reactions is vital for maintaining cellular health and facilitating growth.
Defining NADPH and its Isoforms
NADPH stands for Nicotinamide Adenine Dinucleotide Phosphate. It is closely related to NADH (Nicotinamide Adenine Dinucleotide). The presence of an additional phosphate group distinguishes NADPH from NADH. Two major isoforms of NADPH exist: the β-form and the α-form, with the beta variant being more common in biological systems. This difference in structure not only influences their roles but also their reactivity and interaction with other biomolecules. By promoting various redox reactions, beta NADPH serves as an electron donor in anabolic reactions, contributing significantly to biosynthesis.
Importance in Cellular Metabolism
Beta NADPH is a crucial player in cellular metabolism. It serves primarily as a reducing agent, which is fundamental for the synthesis of lipids, nucleotides, and amino acids. This reducing power is also pivotal for the regeneration of antioxidants, helping the cell to combat oxidative stress.
Moreover, NADPH is essential in the Calvin cycle, wherein it assists in the conversion of carbon dioxide into glucose through a series of light-independent reactions. This demonstrates its importance in energy transfer and carbon fixation processes.
"Beta NADPH is not just a coenzyme; it is the lifeline of metabolic pathways that are crucial for growth and maintenance of cellular functions."
In summary, understanding beta NADPH and its biochemical roles allows for deeper insights into its function within various metabolic pathways. Researchers, educators, and students benefit greatly from grasping the intricacies of this coenzyme, enhancing their comprehension of metabolic functions and broader biological systems.
Biochemical Properties of Beta NADPH
The biochemical properties of Beta NADPH are essential to understanding its functions in various biological systems. This section will discuss its molecular structure and how its functional groups confer specific reactivity. Insights into these properties deepen our awareness of Beta NADPH’s role as an electron donor in metabolic reactions and its significance in maintaining cellular health.
Molecular Structure Analysis
Beta NADPH consists of two nucleotides joined through their phosphate groups. Each nucleotide includes a nitrogenous base, ribose sugar, and a phosphate group. The structure of Beta NADPH is critical because it defines how this coenzyme interacts with enzymes and substrates during biochemical reactions.
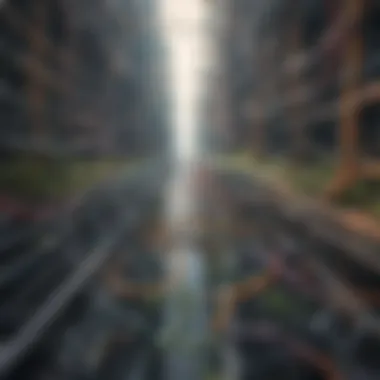
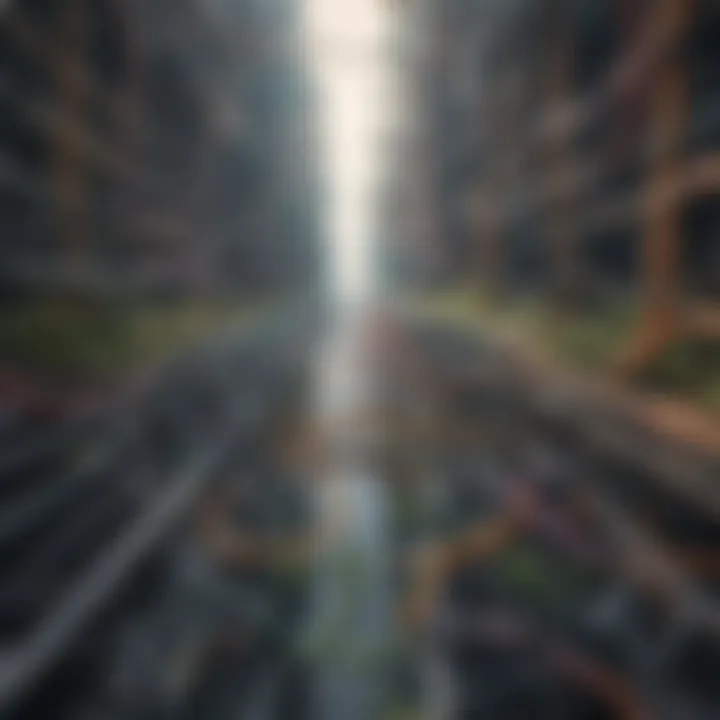
The ribose components of NADPH enable it to serve as a versatile electron carrier. This aspect is crucial in the synthesis of various biomolecules. In Beta NADPH, the presence of the phosphoryl group at the 2-position of the ribose enhances its stability and affinity for certain enzymes. The geometric configuration allows for easy protomerization, facilitating efficient interactions with different substrates in metabolic pathways.
Functional Groups and Reactivity
The functional groups in Beta NADPH include hydroxyl (–OH) and phosphate (–PO4) groups, which play significant roles in its reactivity. These functional groups influence the molecular behaviour in redox reactions. The ability to donate electrons makes Beta NADPH a vital player in reductive biosynthesis, such as fatty acid production and the Calvin cycle in photosynthesis.
Due to the presence of the 2′-phosphate group, Beta NADPH has distinct reactivity compared to its oxidized counterpart, NADP+. This difference is particularly evident in cellular conditions where reduction processes are required. The hydroxyl groups stabilize the carbanion intermediate, allowing Beta NADPH to participate in nucleophilic attacks effectively.
In practice, the specific configuration of the phosphate and hydroxyl groups leads to a versatile coenzyme capable of participating in various enzymatic reactions. These reactivity characteristics make Beta NADPH indispensable in maintaining redox balance and supporting anabolic processes in cells.
"Understanding the biochemical properties of Beta NADPH is fundamental for explaining its role in essential metabolic pathways."
In summary, the molecular structure and functional groups of Beta NADPH define its biochemical identity. Their influence on reactivity has far-reaching implications across numerous cellular processes. Recognizing these properties provides valuable insights into why Beta NADPH is revered in biochemistry.
Synthesis of Beta NADPH
The synthesis of Beta NADPH is fundamentally important for understanding its role in cellular metabolism. This coenzyme acts as a critical electron donor, influencing various biochemical reactions. Understanding how Beta NADPH is synthesized allows researchers to appreciate its versatility in supporting numerous biological functions. This section will analyze the pathways involved in NADPH generation and the specific enzymatic reactions that facilitate its formation.
Pathways for NADPH Generation
There are several key pathways through which NADPH is generated within cells. Two particularly prominent pathways are the pentose phosphate pathway and the malic enzyme pathway.
- Pentose Phosphate Pathway (PPP): This is one of the primary routes for NADPH synthesis. The oxidative phase of the PPP generates NADPH as glucose-6-phosphate is converted into ribulose-5-phosphate. This process is crucial not only for NADPH production but also for the generation of ribose-5-phosphate, a precursor for nucleotide synthesis.
- Malic Enzyme Pathway: This pathway provides another source of NADPH. In this pathway, malate is converted to pyruvate, resulting in the production of NADPH. This pathway becomes especially significant in tissues with high metabolic demands, such as liver and adipose tissues.
Other notable sources include the activity of isocitrate dehydrogenase and the action of glutamate dehydrogenase, both of which can also contribute to the cellular pool of NADPH.
Generating NADPH through these pathways has several benefits. It supports anabolic reactions, protects against oxidative stress, and plays a role in maintaining the overall redox balance within the cell.
Enzymatic Reactions Involved
The synthesis of Beta NADPH is facilitated by various key enzymatic reactions. These enzymatic actions are crucial for effectively generating and regulating NADPH levels within biological systems.
- Glucose-6-Phosphate Dehydrogenase (G6PD): This enzyme catalyzes the conversion of glucose-6-phosphate to 6-phosphoglucono-δ-lactone, producing NADPH in the process. The activity of G6PD is tightly regulated, making it a key control point in the PPP.
- Isocitrate Dehydrogenase: This enzyme also contributes to NADPH synthesis by catalyzing the decarboxylation of isocitrate to α-ketoglutarate, during which NADPH is produced. Its activity provides an important link between the citric acid cycle and NADPH generation.
- Malic Enzyme: As mentioned earlier, the conversion of malate to pyruvate leads to NADPH generation. The malic enzyme plays a significant role in tissues with a high rate of fatty acid biosynthesis.
The interplay of these reactions highlights the complexity and efficiency of NADPH synthesis. The ability to generate Beta NADPH through these various enzymatic pathways showcases its importance in cellular functions, particularly in metabolism and biosynthetic pathways.
Understanding the mechanisms behind Beta NADPH synthesis provides essential insights into its biological significance and its role in health and disease.
Role in Metabolic Pathways
Beta NADPH plays a vital role in many metabolic pathways within biological systems. Its primary function as a reducing agent significantly impacts various biochemical processes. This section highlights its specific contributions, emphasizing both its critical functions and broader implications.
NADPH in the Calvin Cycle
In photosynthetic organisms, the Calvin cycle is essential for carbon fixation, where carbon dioxide is converted into glucose. Beta NADPH is a crucial player in this process, providing the necessary electrons for the reduction of 3-phosphoglycerate into glyceraldehyde-3-phosphate. During this conversion, NADPH donates electrons, which helps in the formation of stable sugars.
Additionally, NADPH provides the reducing power needed to drive other reactions in the cycle. Without sufficient NADPH, the efficiency of glucose synthesis declines. Studies have shown that fluctuations in NADPH levels directly affect the rate of photosynthesis. This indicates a clear relationship between the availability of NADPH and the efficacy of the Calvin cycle.
Contribution to Fatty Acid Synthesis
Beta NADPH also serves an important function in fatty acid biosynthesis. The process involves the acyl carrier protein (ACP) and a series of enzymes that elongate carbon chains. NADPH contributes to the reduction of acyl intermediates, transforming them into fatty acids.
The synthesis of fatty acids is an energy-intensive process that requires a large amount of reducing power. Through the action of enzymes like fatty acid synthase, NADPH is consumed to help convert acetyl-CoA into palmitate, the precursor of many fatty acids. The rate of fatty acid production is, therefore, closely linked to the availability of NADPH. Insufficient NADPH can lead to metabolic blockages and altered lipid profiles.
Detoxification Mechanisms
One of the lesser-known roles of Beta NADPH is in cellular detoxification. It plays a key role in the function of cytochrome P450 enzymes, which are involved in the metabolism of drugs and xenobiotics. In this context, NADPH provides the reducing equivalents needed to convert potentially harmful substances into less toxic forms, facilitating their excretion from the body.
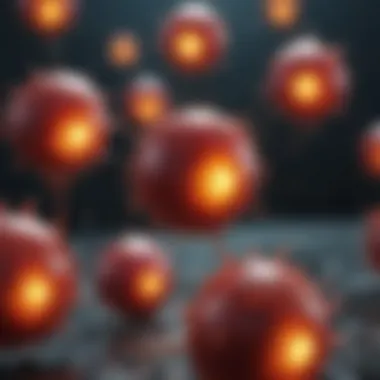
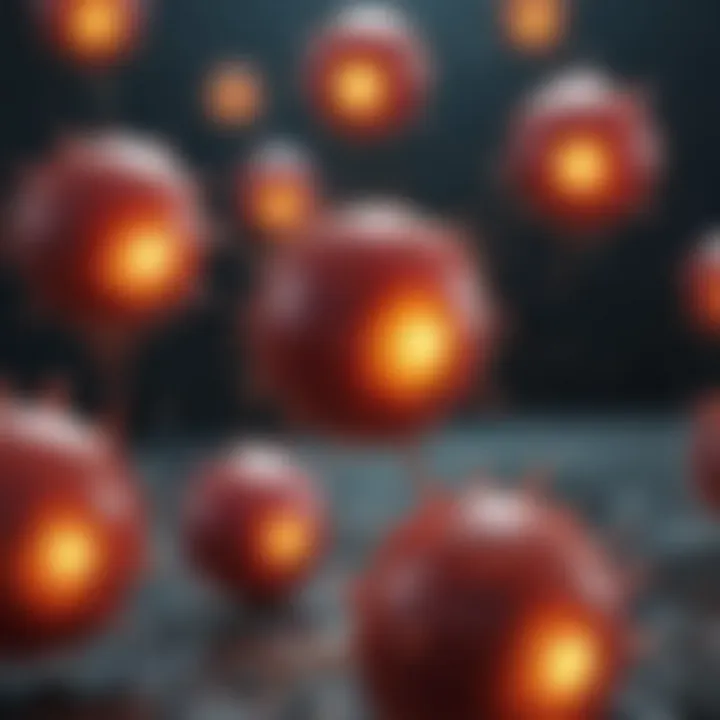
Additionally, NADPH is involved in the production of reduced glutathione, a major antioxidant in cells. This molecule helps to neutralize free radicals and protect cellular components from oxidative damage. The balance between NADPH levels and reactive oxygen species is critical in maintaining cellular homeostasis. A disruption in this balance can have dire consequences, contributing to various diseases.
"Beta NADPH’s role in detoxification mechanisms illustrates its importance not only in metabolic pathways but also in protecting cells against damage."
In summary, Beta NADPH is integral to metabolic pathways, influencing processes from carbon fixation to fatty acid synthesis and detoxification. Understanding these roles highlights its significant impact on overall cellular health and functioning.
Beta NADPH and Cellular Signaling
Beta NADPH plays a significant role in cellular signaling, affecting various biological processes through its involvement in redox reactions. The ability of NADPH to donate electrons makes it essential in maintaining redox homeostasis, which is vital for cell survival and function. Furthermore, Beta NADPH acts as a cofactor for many enzymes, thus influencing cellular pathways involved in signaling.
Impact on Redox Homeostasis
Redox homeostasis refers to the balance between oxidants and reductants within the cell. Beta NADPH is crucial in this context as it functions both as a reducing agent and a source of reducing equivalents. By providing necessary electrons, it helps regenerate glutathione, an important antioxidant in cells. The oxidation-reduction reactions driven by Beta NADPH directly influence the cellular response to oxidative stress. This stress can lead to cellular damage if not managed properly. Therefore, maintaining proper levels of Beta NADPH is essential to facilitate effective cellular responses.
Role in Antioxidant Defense
In terms of antioxidant defense, Beta NADPH supports the regeneration of antioxidants involved in various protective mechanisms. One of its key roles is in the reduction of oxidized glutathione back to its reduced form. Glutathione, being a potent antioxidant, neutralizes reactive oxygen species and mitigates damage to cellular components like DNA, proteins, and lipids.
This relationship underscores the importance of Beta NADPH not just in metabolic pathways but also in safeguarding cellular integrity. It allows cells to endure sometimes detrimental conditions while promoting longevity and functionality.
"Beta NADPH's role in cellular signaling cannot be underestimated, as it ensures that cellular environments remain conducive to optimal functioning."
Additionally, research suggests that disturbances in Beta NADPH levels can lead to a breakdown in the antioxidant defense system. Such disturbances can be linked to various diseases, underscoring why ongoing studies continue to explore its signaling capabilities in more depth.
In summary, the importance of Beta NADPH in cellular signaling is multifaceted. It acts as a critical player in sustaining redox homeostasis and forms an integral part of the antioxidant defense mechanisms. Understanding these roles further elucidates the complexity of biological systems and the essential functions of coenzymes like Beta NADPH.
Implications for Health and Disease
Beta NADPH plays a significant role in maintaining cellular health and influencing various disease processes. Its involvement in redox reactions is crucial for cellular function. In particular, it serves as a reducing agent in many biochemical pathways. Understanding the implications of beta NADPH on health and disease can provide insights into metabolic disorders and cancer biology.
Association with Metabolic Disorders
Metabolic disorders are conditions that arise from abnormal biochemical reactions in the body. Evidence indicates that disruptions in NADPH levels can contribute to various metabolic issues such as obesity, diabetes, and cardiovascular diseases.
- NADPH is vital for fatty acid synthesis and cholesterol metabolism. In conditions where NADPH production is compromised, there can be an increase in fat accumulation and altered lipid profiles.
- Diabetes, for example, is associated with oxidative stress, which is partly controlled by NADPH. Insufficient NADPH levels can lead to impaired detoxification of reactive oxygen species, exacerbating insulin resistance and leading to further metabolic dysregulation.
- Studies have shown that patients with type 2 diabetes often exhibit altered NADPH oxidase activity, leading to increased oxidative stress and inflammation in tissues.
Notably, therapies aimed at enhancing NADPH generation or improving its utilization are being explored as potential strategies to combat the effects of metabolic disorders.
NADPH in Cancer Biology
The relationship between beta NADPH and cancer biology is complex yet crucial. Cancer cells often exhibit altered metabolism, which supports their rapid growth and proliferation.
- Increased levels of NADPH in cancer cells facilitate biosynthetic processes such as nucleotide synthesis and lipid adaptation. This enhanced ability to generate NADPH allows cancer cells to thrive under conditions that would otherwise impede normal cell function.
- Additionally, beta NADPH is necessary for maintaining glutathione levels, a powerful antioxidant. Cancer cells utilize this to counteract oxidative stress and evade programmed cell death.
- Recent research also highlights that manipulating NADPH levels can influence tumor progression. Inhibitors targeting pathways linked to NADPH production are under investigation as potential cancer therapies.
Understanding the dual role of beta NADPH—supporting cell survival under stress while also contributing to disease progression—is essential for developing effective treatment strategies.
Beta NADPH in Biotechnology
Beta NADPH plays an important role in biotechnology, where it serves as a pivotal cofactor in various processes that extend across molecular biology and industrial applications. Its functions are not limited to biological reactions within organisms but also encompass engineered systems that leverage biological processes for scientific and practical advancements. Understanding its applications can empower researchers to develop better methodologies and products.
Applications in Synthetic Biology
In the field of synthetic biology, Beta NADPH is critically important. It provides reducing power required for biosynthetic reactions. This aspect is essential for creating novel pathways in microorganisms. Using genetic engineering, scientists manipulate these organisms to produce high-value compounds, ranging from pharmaceuticals to biofuels.
Some common applications include:
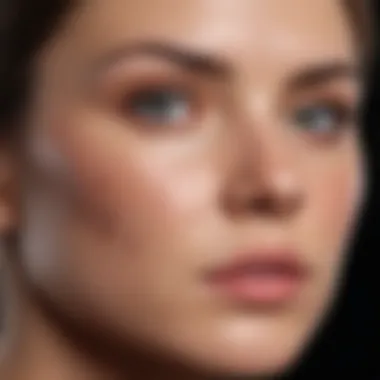
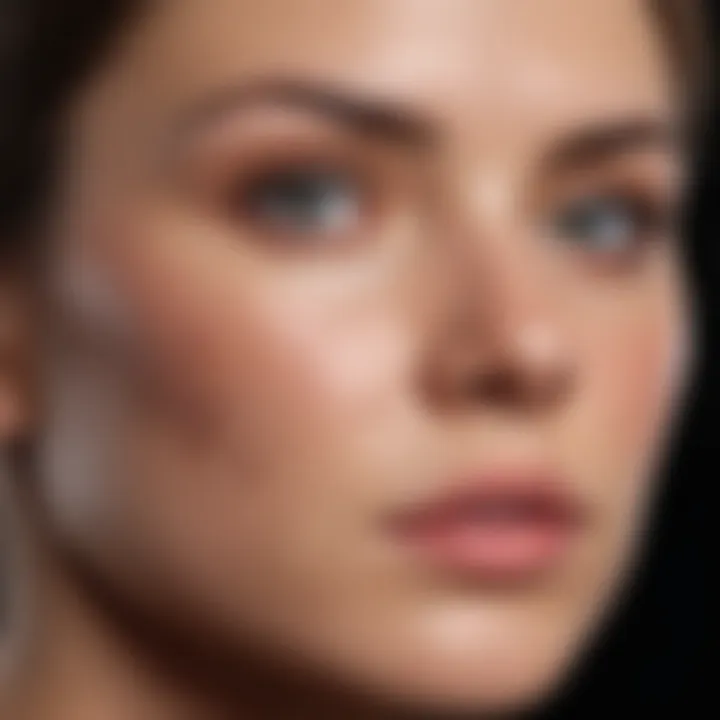
- Production of Biofuels: Microbial strains can be engineered to enhance the production of fatty acids, which are precursors for biodiesel. The presence of Beta NADPH ensures optimal conditions for these biochemical pathways.
- Pharmaceuticals Development: Many drugs require complex organic molecules. Beta NADPH is often a key player in synthetic pathways leading to the production of these molecules.
- Metabolite Overproduction: Scientists utilize Beta NADPH to amplify the yield of important metabolites. This is achieved through metabolic engineering techniques that redirect the flow of metabolic intermediates.
Use in Pharmaceutical Development
Beta NADPH's impact on pharmaceutical development cannot be understated. It is involved in several key enzymatic reactions that synthesize drugs. Understanding these processes can lead to more efficient development of medications.
Considerations in using Beta NADPH in drug discovery and development include:
- Biocatalysis: Enzymes that utilize NADPH are crucial in converting precursors to therapeutic compounds. These biocatalytic processes often have higher specificity and lower environmental impact compared to traditional chemical methods.
- Drug Metabolism: Beta NADPH plays a vital role in the metabolism of xenobiotics. This knowledge is applicable in pharmacokinetics and helps predict how drugs are processed in the body.
- Target Identification: Research on NADPH-utilizing pathways can lead to new targets for drug action. Understanding these interactions results in more innovative therapeutic strategies.
"The integration of Beta NADPH in biotechnology not only enhances efficiency but also opens new doors for innovations across multiple biological applications."
Future Directions in NADPH Research
The study of Beta NADPH is evolving. Understanding its mechanisms and roles in biological systems can unlock new possibilities in various fields. Future research on Beta NADPH will heavily focus on its biochemical pathways and interactions. This research can lead to breakthroughs in medical science and biotechnology.
Emerging Technologies and Techniques
New technologies are reshaping how researchers study Beta NADPH. Next-generation sequencing and high-throughput screening are notable examples. These methods allow for larger datasets and more comprehensive analysis of NADPH-related pathways.
- CRISPR-Cas9: This gene-editing technology can help scientists manipulate genes involved in the synthesis of NADPH. By understanding these genes, researchers can explore their implications in health.
- Metabolomics: This technique measures metabolites in biological samples. It can give insights into how NADPH levels change in different conditions, such as disease states or under environmental stress.
- Proteomics: Studying protein interactions with NADPH can clarify its role in metabolic processes. This approach helps isolate and identify target proteins that use NADPH, shedding light on its function.
These technologies bring new dimensions to NADPH research, making it crucial for exploring - whether investigating basic metabolism or specific disease mechanisms.
Potential for Therapeutic Advances
The potential for therapeutic applications of Beta NADPH is notable. Its role in redox reactions is of interest in disease contexts, such as cancer and metabolic disorders. Understanding how Beta NADPH operates may allow for new treatment options.
Additionally, the modulation of NADPH levels could become a target for drug development. Some potential therapeutic strategies include:
- Antioxidant Therapies: Enhancing NADPH-dependent antioxidant pathways could protect against oxidative stress in various diseases.
- Cancer Treatments: Targeting NADPH levels in cancer cells may affect tumor growth by disrupting metabolic pathways.
- Metabolic Disorders: By managing NADPH synthesis, it may be possible to correct metabolic imbalances.
"Research into NADPH is not just about understanding biology; it is about opening new avenues for treatment."
Overall, the future of NADPH research is promising. It holds significance beyond academic exploration, influencing therapeutic strategies and our understanding of cellular function. The integration of new technologies will enhance knowledge, lead to potential breakthroughs, and significantly impact health outcomes.
End
In summarizing the significance of Beta NADPH, one must acknowledge its pivotal role within biological systems. This coenzyme is not merely a player in metabolic pathways; it is essential for sustaining life through its participation in redox reactions and various biosynthetic processes. Understanding the multifaceted functions of Beta NADPH enriches our comprehension of cellular metabolism and the intricate balance required for health.
Summary of Key Findings
Several key findings emerge from the exploration of Beta NADPH. Firstly, its biochemical properties underline a well-defined molecular structure that allows it to function effectively in organismal processes. It is primarily generated through pathways such as the pentose phosphate pathway and utilized in important reactions including fatty acid synthesis and the Calvin cycle. Furthermore, indicators of its critical roles in redox homeostasis and cellular signaling illuminate how organisms maintain their internal balance under varied conditions.
- Biochemical Roles:
- Health Implications:
- Biotechnological Applications:
- Crucial for biosynthetic pathways.
- Key player in detoxification processes.
- Linked to metabolic disorders and cancer.
- Potential marker for therapeutic strategies.
- Significant in synthetic biology.
- Integral for pharmaceutical developments.
Understanding these aspects not only provides insights into metabolic pathways but also emphasizes the therapeutic potential of targeting Beta NADPH in various diseases.
Significance in Understanding Biological Systems
The examination of Beta NADPH extends far beyond mere biochemical reactions. It serves as a cornerstone for understanding broader biological systems. Such knowledge is instrumental for researchers and practitioners in medicine, biotechnology, and related fields.
By recognizing how Beta NADPH facilitates critical metabolic functions, professionals can derive implications for health and disease management. For example, disorders associated with imbalances in NADPH can lead to conditions such as diabetes or cancer, emphasizing the need to monitor and potentially manipulate levels of this coenzyme in therapeutic contexts.
Moreover, advances in biotechnology highlighting Beta NADPH open new avenues for innovation. Recognizing its role in synthetic biology can lead to groundbreaking methods for sustainable production of essential compounds, thereby contributing to environmental efforts and health solutions.
In summary, the findings reveal that Beta NADPH plays an indispensable role in sustaining life, driving home the fact that understanding its functions is crucial for harnessing its potential in health and industry.