Dynamic Light Scattering Systems: Principles and Applications
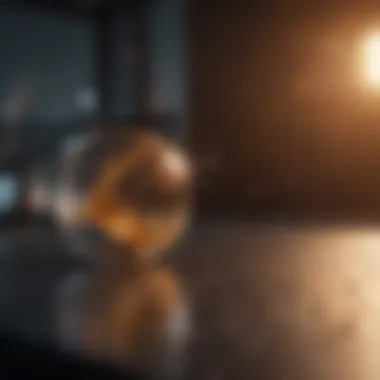
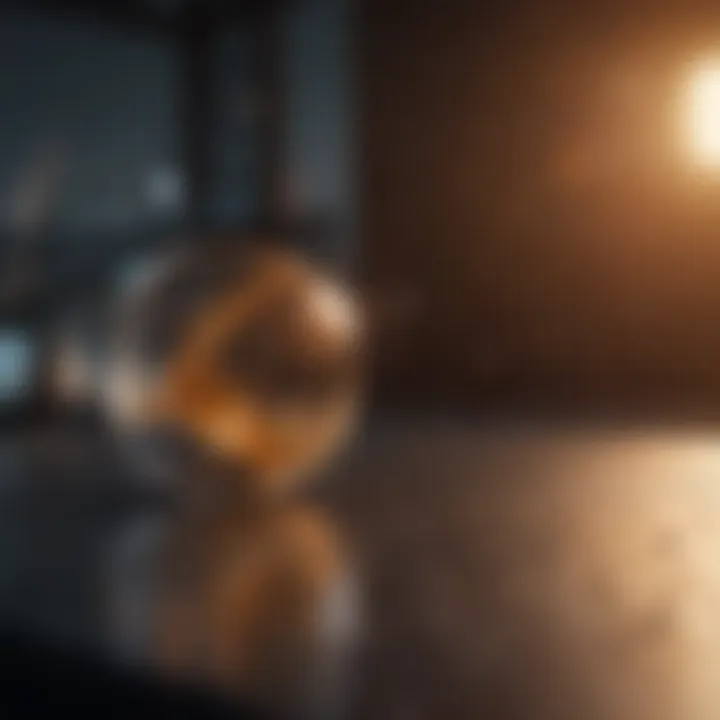
Intro
Dynamic light scattering (DLS) systems have emerged as a cornerstone in the world of particle characterization. This technique provides critical insights into the size and distribution of small particles in a variety of contexts. As we delve into the realms of scientific research and industrial applications, the significance of DLS becomes undeniably clear. From pharmaceuticals to nanotechnology, the ability to accurately analyze particles offers a deeper understanding of material properties and behaviors.
Yet, what makes DLS stand out among other characterization techniques? To put it simply, it’s a game changer. Unlike methods that require extensive sample preparation or can only analyze larger particles, DLS excels at assessing nanometer-sized particles with minimal interruption, allowing researchers to focus on the fundamental properties that often govern material performance.
In this article, we will traverse the intricate landscape of dynamic light scattering. We aim to clarify its foundational principles, explore its versatile applications across various fields, highlight cutting-edge innovations, and dissect the nuances of data interpretation. By the end of this journey, readers will appreciate not just the how's but also the why's of DLS in modern science.
Fundamentals of Dynamic Light Scattering
Dynamic Light Scattering (DLS) stands as one of the keystones in particle size analysis, especially in colloid science and nanotechnology. Understanding the fundamentals of DLS proves crucial for researchers and professionals aiming to harness this method for various applications—from drug formulation to material science. These principles lay the groundwork for interpreting complex interactions between light and microscopic particles.
By grasping these foundational concepts, users can make informed decisions about sample preparation, instrument settings, and data interpretation, leading to more reliable outcomes in experiments and studies.
Basic Principles
At its core, dynamic light scattering relies on the elastic scattering of light by particles suspended in a medium. The fundamental principle involves illuminating a sample with a coherent light source, typically a laser. When light encounters small particles, it scatters in various directions. The key aspect of DLS is analyzing how these scattered light patterns vary with time. These variations correlate to the movement of the particles in the solution, predominantly influenced by their size and the viscosity of the medium.
The intensity of the scattered light fluctuates due to the Brownian motion of the particles. Larger particles move more slowly, while smaller ones scatter light more dynamically. This time-dependent fluctuation captures the essence of the DLS technique. By employing sophisticated algorithms, researchers can extract particle size distributions from the changing light patterns.
Light and Particle Interaction
The interaction between light and particles is not merely a mechanical process but also one rooted in physical principles. The size of the particles relative to the wavelength of light plays a pivotal role. If the particles are small enough (typically less than one-tenth the wavelength of the incident light), they scatter light at lower angles. In contrast, larger particles produce more significant scattering effects and may scatter light across broader angles.
As particles oscillate in the solution, they create a dynamic environment where the light's phase and amplitude change continually. The relationship between scattering angle and the size of the particles is essential in determining the particle size distribution. Hence, understanding how light interacts with particles is fundamental for both interpreting DLS results and troubleshooting experimental setups.
Mathematical Foundations
The mathematical underpinnings of DLS are equally critical, involving various equations to describe the light scattering phenomenon and particle motion. At a basic level, one employs the Stokes-Einstein equation to relate the diffusion coefficient of a particle to its size:
[ D = \frackT6\pi \eta r ]
where:
- D is the diffusion coefficient
- k is Boltzmann's constant
- T is the absolute temperature
- η is the viscosity of the solvent
- r is the radius of the particle
By manipulating this equation, researchers can derive the hydrodynamic radius of particles, helping determine their behavior in various formulations and environments.
Additionally, autocorrelation functions play a fundamental role in DLS data processing by providing a powerful method to analyze the temporal fluctuations of scattered light intensity. The average time interval over which scattered light rearrangement occurs leads directly to insights about particle size and distribution.
"Unlocking the mathematical characteristics of DLS is akin to decoding a language of particle dynamics—empowering researchers to extract valuable insights from their scattered light studies."
The integration of these mathematical models with experimental data allows for a comprehensive understanding of particle behavior, lending credence to the significance of DLS in scientific research and industry applications.
Instrumentation in DLS Systems
Instrumentation is the backbone of dynamic light scattering (DLS) systems, facilitating the measurement and analysis of particles in suspension or solution. Understanding how these instruments work and their key components is crucial for anyone involved in research or practical applications of DLS. The efficiency, accuracy, and quality of results depend largely on the rigors of instrumentation.
Essential Components
Every DLS system is composed of several essential components, each playing a vital role in the functioning of the apparatus. These components ensure precise data collection and analysis, which leads to reliable results in various applications.
- Laser Source: The heart of any DLS system is its laser. A coherent light source provides the illumination necessary for scattering experiments. The choice of laser's wavelength can significantly affect the results obtained, often leading to different scattering patterns.
- Detector: After the laser light hits the particles, it gets scattered. The detector picks up this scattered light. Technologies like photomultiplier tubes or avalanche photodiodes are commonly used. High sensitivity and speed in detecting variations are crucial,
- Sample Holder: This is where the sample is contained during measurement. The design of this component can influence how well the light interacts with the particles. Good sample holders are often designed to minimize background noise and optimize the scattering events.
- Data Processing Unit: Finally, the data collected by the detector is sent to a processing unit. This unit analyzes the information and converts it into useful data regarding particle size and distribution.
Calibration and Setup
Calibration is essential for achieving accuracy and reliability in DLS measurements. Proper setup ensures that the system is ready to deliver correct insights.
- Initial Calibration: The DLS system must be calibrated with known standard materials, helping to establish a baseline for measurements.
- Alignment of Components: Components like lasers and detectors must be carefully aligned. Misalignment can lead to erroneous data, potentially skewing results.
- Temperature Control: Since particle movement can be affected by temperature, maintaining a constant temperature during measurements is necessary. This often involves integrating temperature controls into the sample holder.
Measurement Techniques
In practice, various measurement techniques can be employed within DLS systems. Although the core principle remains constant, the method of data acquisition can vary significantly:
- Exponential Decay Methods: In this technique, intensity fluctuations of scattered light are analyzed over time to derive particle size.
- Multi-Angle Scattering: This involves measuring the scattering at various angles, allowing for a more generalized analysis of particle distributions rather than a single, fixed angle.
In the realm of particle sizing, the precision of measurements is of utmost importance; even minor fluctuations can greatly influence outcomes.
- Digital Signal Processing: Advanced systems may use digital signal processing algorithms to extract meaningful data from the intensity fluctuations, enhancing signal clarity and measurement accuracy.
Thus, the instrumentation in DLS systems not only encompasses multiple complex parts but also requires careful calibration and the application of various measurement techniques to yield valuable scientific insights.
Particle Size Analysis
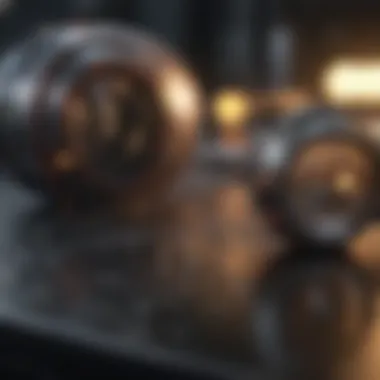
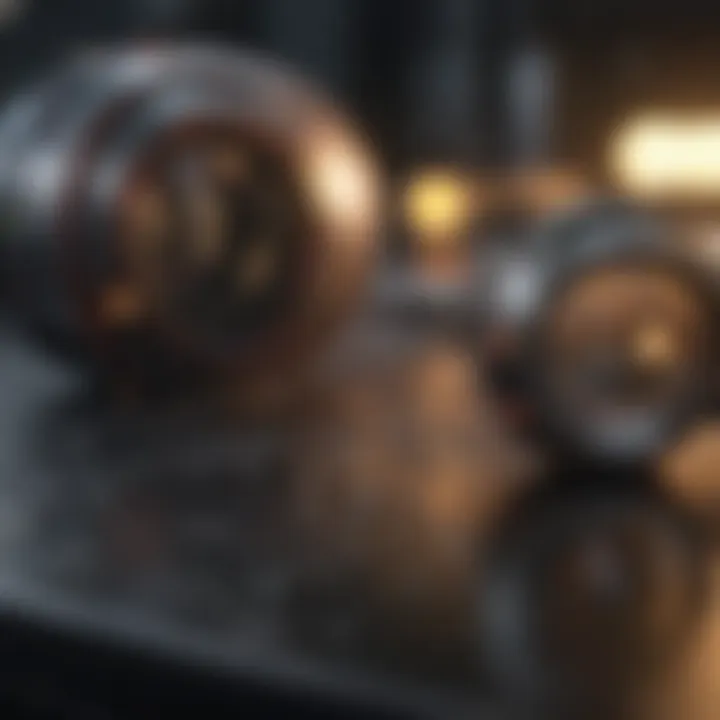
In the realm of dynamic light scattering (DLS), particle size analysis stands as a crucial component, serving as a gateway to understanding the behavior of colloidal systems. This significance is not merely academic; rather, it resonates deeply across various industries, influencing everything from pharmaceutical formulations to nanotechnology advancements. By accurately measuring particle sizes and distributions, researchers and practitioners can tailor their approaches to enhance product performance and quality.
The focus on particle size analysis brings with it a multitude of benefits. First and foremost, it helps in characterizing the physical and chemical properties of materials. By determining sizes, one can predict how particles will interact with each other and their surrounding environments. Another critical element is the impact on product stability. In suspensions, larger agglomerates may settle out over time, potentially leading to issues in product efficacy. Hence, businesses cannot overlook this aspect when developing consistent production methods.
Let's break down some specific elements that underscore the importance of particle size analysis in DLS:
- Quality Control: Ensuring that particle sizes fall within designated thresholds is vital for maintaining product quality.
- Customization of Formulations: Different applications require distinct particle sizes. For instance, in pharmaceuticals, the bioavailability of a drug can often hinge on the size of its active particles.
- Regulatory Compliance: Many industries are governed by strict standards. Demonstrating controlled size distributions can aid in satisfying these regulations.
Size Distribution Profiles
Size distribution profiles offer a visual representation of particle size within a sample. Understanding these profiles is essential because they reveal not only the average particle size but also the spread and uniformity of sizes. In practical terms, they help identify any predominance of certain sizes, which can be indicative of underlying issues during the production process or changes within a sample.
A common approach for generating size distribution profiles is through histogram plots, which can effectively communicate information about particle populations.
When interpreting these distributions, keep in mind:
- Symmetry: A symmetrical distribution may hint at a controlled production process, whereas asymmetrical ones could suggest agglomeration or variability.
- Range: A wide distribution may affect performance, particularly in sensitive applications like drug delivery or material science.
Polydispersity Index
The Polydispersity Index (PDI) functions as a pivotal measure of the breadth of size distribution in a sample. Conceptually, it indicates the uniformity of particle sizes: a lower PDI denotes a narrow size distribution, while a higher PDI suggests more diversity.
For example, an index value close to 1 implies a predominantly uniform sample, which is often desirable in applications requiring homogeneity. Conversely, a PDI much greater than 1 could signal a problem, such as poor formulation control, or it may highlight a purposeful design for certain applications.
Applications in Nanotechnology
Nanotechnology thrives on precise control of particle sizes and distributions, making particle size analysis through DLS a game-changer for this field. Applications are numerous, spanning the development of novel materials, drug delivery systems, and even environmental remediation technologies. With nanoparticles often exhibiting unique properties due to their size, understanding their behavior in various contexts is paramount.
Some specific applications in nanotechnology include:
- Drug Delivery: Optimizing a drug's size can enhance its absorption and targeted delivery.
- Nanocomposites: Particle size impacts the mechanical properties and strength of these composites, crucial in materials science.
- Environmental Cleanup: Analyzing size distributions of nanoparticles used to adsorb pollutants can inform strategies on effectiveness.
In summary, particle size analysis in dynamic light scattering is not just an analytical step; it plays a critical role in product development, quality assurance, and advancing scientific knowledge across various disciplines. Its implications are broad and impactful, setting the stage for innovative advancements in technology and industry.
Applications of DLS in Various Fields
Dynamic Light Scattering (DLS) systems play a pivotal role in various scientific domains, facilitating a deeper understanding of particle behavior in diverse environments. The versatility of DLS allows it to serve as an essential tool in addressing complex challenges faced by researchers across numerous fields. Its ability to provide information on particle size, distribution, and movement aids in advancing both theoretical studies and practical applications. Below, we explore how DLS finds relevance in biological sciences, material science, the pharmaceutical industry, and environmental monitoring.
Biological Sciences
In the biological realm, DLS is employed to elucidate the properties of macromolecules, such as proteins and nucleic acids, which are largely significant in understanding cellular functions. Researchers utilize DLS to obtain size distributions of these biomolecules in solution, providing insights into their conformation and interactions. This is particularly crucial when studying complex biological systems, where the scattering profiles can indicate aggregation or conformational changes.
One compelling aspect of using DLS in biological sciences is its non-invasive nature, allowing for measurements under physiological conditions. It is often employed in the development and characterization of nanoparticles used for drug delivery systems. By analyzing the size and stability of these nanoparticles, researchers can assess their effectiveness in transporting therapeutic agents within the body.
Material Science
Material science leverages DLS to investigate various particulate systems, from emulsions to colloidal dispersions. The ability of DLS to measure particle size on the nanoscale helps scientists understand fundamental properties that dictate material performance and behavior. For instance, in the development of new materials like nanocomposites, knowing size distribution and polydispersity is crucial for tailoring properties such as strength, conductivity, and thermal stability.
Furthermore, DLS aids in real-time monitoring of processes such as polymerization, where particle size changes during reactions can significantly influence the final product's qualities. This responsiveness makes DLS a valuable asset in production quality control—ensuring uniformity and reliability in material characteristics.
Pharmaceutical Industry
Dynamic Light Scattering is indispensable in the pharmaceutical sector, where understanding particle size contributes directly to the efficacy of drug formulations. Many drugs are administered as nanoparticles or colloidal systems, and their therapeutic effect is closely related to their size and distribution. In this framework, DLS assists in characterizing active pharmaceutical ingredients (APIs) and excipients, ultimately impacting drug absorption and biodistribution.
Moreover, during formulation development, DLS can monitor stability and aggregation of suspensions and emulsions. The insights gained guide researchers in optimizing formulations before clinical testing. When quality concerns arise, DLS serves as a rapid diagnostic tool, facilitating timely corrections in production processes.
Environmental Monitoring
Environmental applications of DLS are gaining traction, particularly in assessing particulate matter in air or water bodies. Monitoring pollutants and understanding their size and behavior is crucial for environmental health. With its ability to measure small particles, DLS helps researchers gather data on the dispersion of contaminants, crucial for regulatory compliance and public safety.
Using DLS, scientists can quickly analyze sediment in aquatic environments or track changes in aerosol concentrations in the atmosphere. This information informs environmental policies and remediation strategies, showcasing DLS's role as an essential instrument not just in research but in practical applications aimed at protecting our ecosystem.
Challenges in Dynamic Light Scattering
The examination of dynamic light scattering (DLS) offers numerous insights into particle characterization, but it does not come without its hurdles. Recognizing these challenges is crucial for both novice and experienced practitioners in this field. Addressing these difficulties can greatly enhance the reliability and accuracy of results. Specific elements such as understanding the refractive index variations, dealing with sample preparation issues, and mitigating the influence of environmental factors encapsulate the essence of challenges faced in DLS.
Refractive Index Variances
One of the paramount challenges in DLS arises from variances in the refractive index between the particles and the surrounding medium. Any mismatch can skew the scattering intensity and consequently lead to inaccurate particle size measurements. This issue is particularly pronounced when dealing with mixtures of particles having diverse refractive indices.
- The scattering function is highly sensitive to these variances, changing expectations in size distributions.
- Misinterpretations could find root in optical misalignments or incorrectly assumed values of the refractive index, especially in aqueous solutions.
- Researchers should pay careful attention to the material’s properties, conducting comparative studies where necessary.
Ultimately, establishing a calibrated model that accurately reflects the system's refractive indices is vital for reliable data.
Sample Preparation Issues
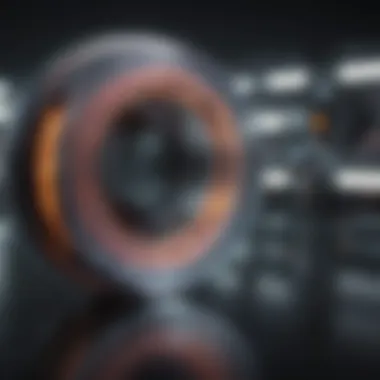
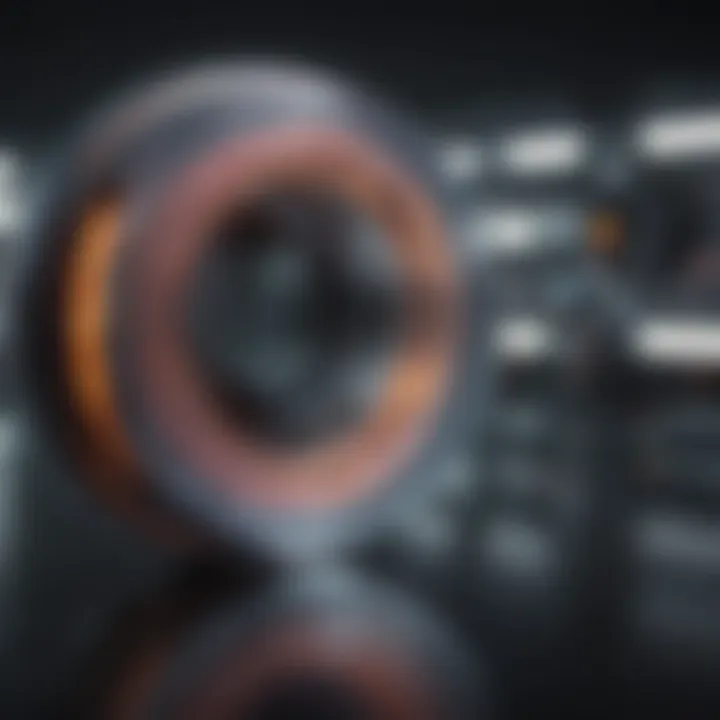
Sample preparation is another critical aspect that often presents challenges in DLS applications. The way samples are handled before measurement can directly impact the scatter data obtained. Particularly, pollutants or excluded components can lead to inconsistencies. Here are some common issues observed:
- Aggregation: Sample components might tend to cluster together, which can misrepresent size distributions.
- Concentration Levels: An ideal concentration range is essential for effective measurements. Overly diluted or concentrated samples can further complicate the interpretation.
- Solvent Effects: The choice of solvent can also influence particle behavior and scattering characteristics.
An effective strategy to overcome these issues would involve methodical sample preparation protocols, such as using ultrafiltration techniques to remove larger aggregates and ensure homogenous solutions.
Environmental Factors
Environmental conditions play a significant role in the performance of DLS techniques. Suboptimal conditions can lead to fluctuations in measurements. Factors to keep in mind include:
- Temperature Fluctuations: Variations can alter the dynamics of particles and affect scattering outcomes.
- Vibrations: External vibrations from surrounding equipment can introduce noise into measurements, resulting in less reliable data.
- Humidity Levels: Particularly when working with hygroscopic materials, the moisture content in the air can change the effective size of particles.
To guard against these external influences, an isolated and controlled environment for measurements becomes paramount. Implementing rigorous quality control measures helps ensure that environmental variations do not compromise data integrity.
"Challenges in DLS can often become pivotal learning opportunities that refine techniques and enhance data accuracy."
Recent Innovations in DLS Technology
Dynamic Light Scattering (DLS) has undergone remarkable changes in recent years, driven by the need for more precise measurements and deeper insights into particle characterization. This section focuses on the advancements that have reshaped the landscape of DLS technology, highlighting how they contribute to improved data quality and broadened applicability across various fields.
Advancements in Detection Systems
One of the most significant leaps in DLS technology has been in detection methods. Traditional DLS systems often relied on basic photodetectors, which could limit sensitivity and dynamic range. Nowadays, new detection systems utilizing advanced photon counting techniques have come to the forefront. These developments allow for the observation of lower concentrations of particles and enhance the system's capacity to measure smaller particles with greater accuracy.
Moreover, the two-dimensional detection systems are emerging as a game-changer. They provide richer data by measuring the scattering pattern of light in multiple dimensions, facilitating better resolution of particle size and shape. Such innovations not only elevate measurement precision but also speed up the data acquisition process, making high-throughput studies more feasible. This is particularly advantageous in fields like pharmaceuticals where time is critically important.
Integration with Other Characterization Techniques
The trend of integrating DLS with complementary techniques is another noteworthy advancement. When combined with methods such as Electron Microscopy (EM) or Nuclear Magnetic Resonance (NMR), DLS results can now be cross-verified, furnishing a more holistic understanding of particle properties. For example, pairing DLS with EM helps confirm dimensions obtained through light scattering by providing visual confirmation.
Additionally, the integration with techniques like Atomic Force Microscopy (AFM) allows researchers to analyze not only size but also surface properties. This multi-faceted approach enhances the capability to characterize complex nanoparticles and aggregates, which is pivotal in materials science and biological applications. The comprehensive data produced by these integrated systems helps in a deeper understanding of particle interactions and dynamics, proving essential for researchers.
Software Developments for Data Analysis
As DLS technology evolves, so too does the software that supports data analysis. Modern DLS systems are now equipped with sophisticated software tools that employ machine learning algorithms to enhance data interpretation. These tools can process vast amounts of scattering data, offering insights that were previously unattainable through conventional statistical methods.
Additionally, visualization capabilities have significantly improved; users can now generate advanced graphical representations of particle distributions and dynamics. The implementation of user-friendly interfaces allows researchers, even those less experienced in data analysis, to extract valuable conclusions from their experiments.
The advent of cloud-based data analysis platforms facilitates collaboration among scientists from different locations, providing them with the ability to share findings and interpretations seamlessly. This trend not only supports enhanced productivity but also fosters innovation through collective problem-solving in the scientific community.
The advancements in DLS technology are not just about better equipment; they also pave the way for transformative research that could redefine our understanding of particle interactions in various fields.
Data Interpretation in DLS
Data interpretation in Dynamic Light Scattering (DLS) is a critical aspect that can’t be overlooked. Understanding how to analyze the data produced by DLS systems enhances the ability to make inferences about particle sizing and distribution. With the vast amount of information generated, moving beyond raw data to meaningful interpretations is fundamental in both research and industrial applications.
In simple terms, data interpretation helps researchers and professionals make informed decisions based on the characteristics of the particles under scrutiny. The significance of this area lies in the ability to accurately determine concentrations, track changes in particle size over time, and ascertain the stability of formulations. This is especially pertinent in fields like pharmaceuticals and nanotechnology, where precision is key to product performance and safety.
Correlation Functions
Correlation functions are a bedrock of DLS data analysis. They provide insights into the dynamics of scattered light, which reflects the movement of particles in suspension. Essentially, these functions analyze how the intensity of scattered light fluctuates with time. The underlying idea is to capture the temporal changes and relate them to particle motion.
Effective interpretation of correlation functions allows scientists to extract crucial parameters such as diffusion coefficients, which are essential for determining particle sizes. To perform these analyses, multiple mathematical approaches, including exponential decay fitting, are employed. This adds a layer of complexity to the interpretation process but exponentially increases the accuracy of the size information extracted.
"The correlation functions serve as a bridge between the physical behavior of particles and the quantitative data needed for analysis."
Analysis Models and Algorithms
The next step in DLS data interpretation involves applying various models and algorithms to extract meaningful size distributions from collected data. Commonly used models include the Stokes-Einstein equation and more sophisticated algorithms like Non-Negative Least Squares (NNLS). Each model has specifications regarding the assumptions made, including particle shape and distribution, which can impact outcomes.
Consideration of these factors is vital, as they dictate how representative the size estimates are. For instance, a model that assumes perfectly spherical particles may not yield accurate results for elongated or irregularly shaped ones. Moreover, advancements in machine learning have opened new avenues for creating predictive models that can analyze data with greater agility and context.
Quality Control Metrics
Quality control in DLS is paramount. Ensuring that the interpreted data accurately reflects the true characteristics of the sample is essential for reproducibility and reliability of results. Various metrics are employed to assess the quality of the measurements, including:
- Auto-correlation Function Quality: This determines the stability and repeatability of the light intensity fluctuations.
- Signal-to-Noise Ratio (SNR): A high SNR indicates reliable data, as it minimizes noise and enhances clarity in particle motion interpretation.
- Particle Concentration Limits: Understanding how concentration affects measurements helps in designing experiments that yield the most accurate results without overwhelming the system.
Through diligent microscopic scrutiny of these quality control metrics, researchers can make more resilient interpretations that stand up to peer scrutiny.
Data interpretation in DLS isn’t merely about crunching numbers; it acts as a linchpin that connects theory to practical applications. The importance of nuanced analysis can never be overstated, especially in fast-evolving scientific fields.
Comparison with Other Particle Sizing Techniques
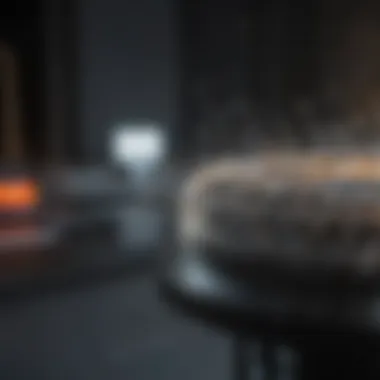
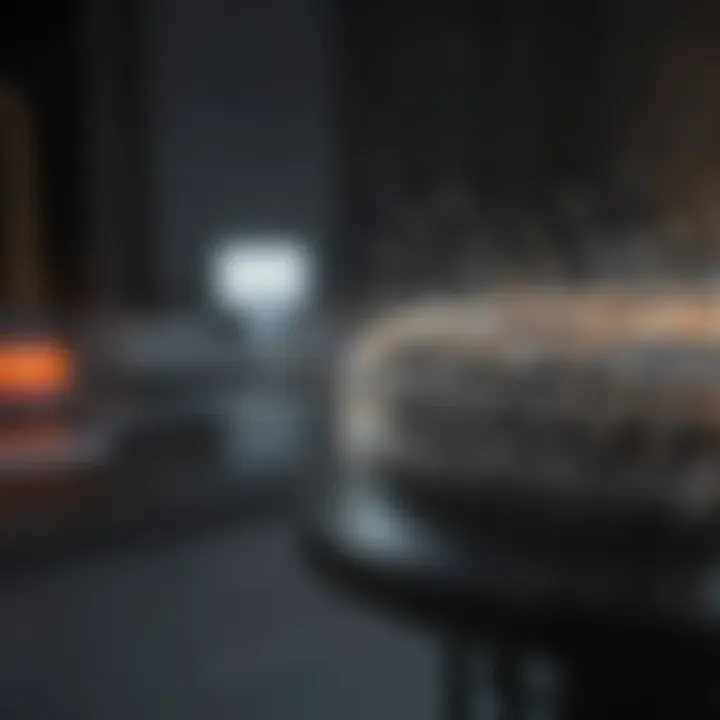
Understanding how dynamic light scattering (DLS) compares to other particle sizing methods is crucial for a well-rounded view of particle characterization. Different techniques can provide unique insights, and choosing the appropriate one can ultimately depend on the specifics of a study. Here, we'll delve into the nuances of DLS alongside other prominent methods, highlighting the elements that make each technique distinctive.
Dynamic vs Static Light Scattering
Dynamic and static light scattering (SLS) are both widely utilized methods; however, they serve different purposes. Dynamic light scattering measures the fluctuation in light scattered by particles in motion, aiming to determine the size and distribution of particles in suspension. This is particularly useful when identifying nanoparticles because it can capture rapid temporal changes. On the other hand, static light scattering focuses on the intensity of scattered light, revealing insights into the particle's molecular weight and structure rather than just size.
One key point of consideration is sensitivity. DLS often detects smaller particles than SLS, particularly in cases of low concentration, making DLS more suitable for applications involving nanoparticles or biologically relevant samples.
DLS vs. Laser Diffraction
Dynamic light scattering and laser diffraction both aim to measure particle size but employ different methodologies. Laser diffraction analyzes the angle of light scattered from a laser beam passing through a particle suspension. The idea is that larger particles scatter light at shallower angles, while smaller particles scatter at wider angles. This differentiation can make laser diffraction useful for larger particles, especially in dry powders or emulsions.
However, DLS shines in the world of tiny particles, particularly those in the nanometer range. While laser diffraction might struggle with truly fine particles due to diffraction limitations, DLS can effectively measure them. DLS also excels in scenarios where particle polydispersity is high and knowledge of the size distribution is crucial for application, such as in drug formulations.
Use Cases for Each Technique
When considering which sizing method to employ, context and requirements of the study must be front and center. Below are some illustrative use cases:
- DLS is often favored in:
- Static light scattering is typically used for:
- Laser diffraction cases include:
- Characterizing nanoparticles in drug delivery systems
- Analyzing protein aggregates or other biomolecules
- Investigating dispersions in food science
- Analyzing large polymer systems
- Determining the molecular weight of macromolecules
- Studying gel formation processes
- Analyzing aggregates in a cement or mortar mixture
- Measuring the size distribution of various industrial powders
- Evaluating emulsions or suspensions where larger particles are dominant
In sum, while DLS is a powerful tool particularly well-suited for analyzing small particles, static light scattering and laser diffraction each have their own strengths in different contexts. The choice of particle sizing technique should align closely with specific project requirements, ensuring accurate and meaningful data in scientific and industrial applications.
"Choosing the right particle sizing method is not just about size; it's about insight. Different techniques offer diverse perspectives on the same problem."
Ultimately, the comparison of these methods underscores the importance of contextuality and application in particle analysis.
Future Directions in DLS Research
The landscape of dynamic light scattering (DLS) is ever-changing, with a wave of advancements promising to open new doors in various fields. This section focuses on the future of DLS technology, shedding light on how emerging trends are poised to enhance sensitivity, broaden applications, and introduce innovative industrial uses.
Enhanced Sensitivity and Resolution
One of the most critical trends in DLS technology is the continual striving for enhanced sensitivity and resolution. As researchers seek to characterize increasingly smaller and more complex particles, the demand for more precise measurements escalates. Improved sensitivity allows for the detection of subtle changes in particle size and distribution, which can be crucial in applications ranging from drug formulation to material science.
Consider the integration of advanced detection systems, such as high-sensitivity detectors combined with sophisticated light sources. These improvements enable the capture of faint signals scattered by tiny particles, thereby facilitating measurements that were previously unattainable. Moreover, the ongoing development of algorithms for data analysis plays a fundamental role in maximizing the utility of the information obtained. Enhanced sensitivity translates to better quality data, reducing the ambiguities in size distributions and aiding researchers in making more informed decisions.
Cross-disciplinary Applications
The versatility of DLS systems makes them a perfect candidate for cross-disciplinary applications. As the boundaries between scientific disciplines blur, DLS is finding a significant role in various fields like biology, materials science, and nanotechnology. By enabling researchers from diverse backgrounds to analyze particle properties, DLS fosters collaborative projects that yield innovative solutions.
For instance, in the realm of biochemistry, DLS is employed not only to understand drug delivery systems but also to probe protein interactions and interactions at the molecular level. Meanwhile, in environmental science, it helps in tracking pollutants at nano-scale dimensions. Such cross-disciplinary integration enriches the data pool and cultivates holistic perspectives on problem-solving.
A few examples of fields benefiting from DLS include:
- Pharmaceuticals: Tailoring drug delivery mechanisms.
- Material Sciences: Studying the mechanical properties of new materials.
- Environmental: Monitoring pollutants in real-time.
This interconnectedness emphasizes how future explorations in DLS can yield insights that are both profound and multi-faceted.
Innovative Applications in Industry
As industries evolve, so do their needs, which beckons for innovative applications of DLS. The future will likely see DLS being adapted for real-time analysis within manufacturing processes. Picture this: a DLS system integrated into a production line, continuously measuring particle sizes and distributions in a fluid medium. This would lead to immediate adjustments in the process, ensuring that product quality remains uncompromised.
Furthermore, with the increasing focus on sustainable practices, the ability to analyze nanomaterials for waste management or resource recovery is extremely valuable. DLS can provide insights into the behavior and distribution of nanoparticles in systems, allowing industries to optimize their processes for better environmental compliance.
"Innovations in DLS technology are bound to redefine analytical methods in several sectors, paving the way for sustainable industrial practices."
As the demand for precision and functionality grows, DLS stands on the threshold of exciting opportunities, making it a cornerstone for research and practical applications alike.
Closure
Dynamic light scattering (DLS) stands at the forefront of particle characterization techniques, offering unmatched insight into the behavior of small particles suspended in liquids. This article has taken a thorough look at the principles, applications, and innovations tied to DLS, serving as an essential resource for scholars, industry professionals, and researchers alike.
Summary of Key Points
DLS remains unequivocally valuable in multiple fields, from biological sciences to material analysis. Key points discussed include:
- Principles of Operation: We delved into how DLS leverages the fluctuations in scattered light intensity to estimate particle size based on Brownian motion.
- Instrumentation: The article highlighted the components necessary for effective DLS, such as lasers, detectors, and correlators.
- Applications: We explored a variety of applications across industries including pharmaceuticals and environmental monitoring, illustrating the versatility of DLS in real-world scenarios.
- Recent Innovations: The advancements in detection systems and software analytics shed light on how technology continually enhances data accuracy and interpretation.
- Continuous Research is Crucial: Emphasizing ongoing developments, we uncovered why staying at the cutting edge is imperative for maximizing DLS capabilities.
The Importance of Continuous Research
The field of dynamic light scattering is not stagnant; it evolves with every advancement in technology and methodology. Continuous research plays a critical role in several ways:
- Improvement of Sensitivity: Researchers strive to enhance the sensitivity of DLS equipment, allowing for the detection of even smaller particles and making it applicable to new areas of study.
- Cross-disciplinary Approach: By integrating insights from different domains, such as physics, chemistry, and biology, DLS can tackle complex research questions that were previously thought to be insurmountable.
- Staying Ahead of the Curve: Engaging in ongoing research ensures that both academic institutions and industry players do not lose their competitive edge. This is particularly relevant for businesses aiming to apply DLS for product innovation and quality control.
In summary, the significance of DLS and its applications will only grow as new technologies emerge and our understanding of particle dynamics expands. Investing in this research is not just a suggestion—it’s a necessity for contributing to advancements that could very well redefine entire fields.