Understanding Electricity Generation in Power Plants
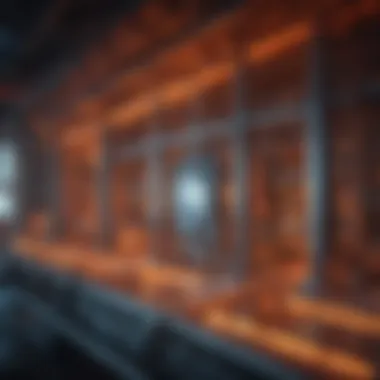
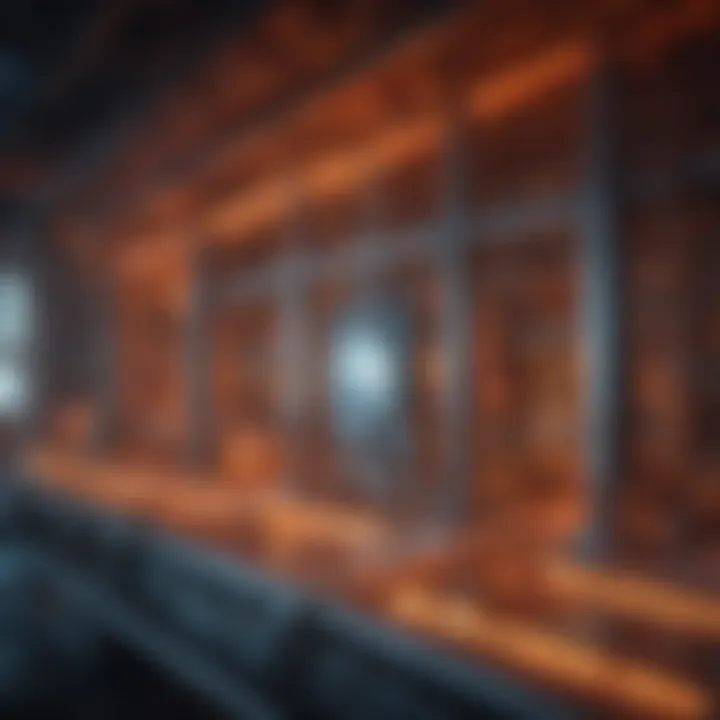
Intro
Electricity has woven itself into the very fabric of modern life, powering our homes, industries, and increasingly our methods of transportation. Understanding how power plants generate electricity is not just an academic pursuit; it’s crucial for grasping today's complexities in energy production and consumption.
The job of a power plant is to convert raw energy into a form that can be easily distributed and used—this process is anything but simple. Each generation method fares differently when it comes to efficiency, cost, and environmental ramifications.
It’s essential to peel back the layers on how thermal, hydroelectric, nuclear, and renewable electricity generation operate, especially when the conversation about sustainable energy is becoming ever more urgent. Let’s break down how these systems work and what implications they hold for our future.
Research Highlights
Overview of Key Findings
As we dive into the various electricity generation methods, several themes emerge:
- Energy Conversion Efficiency: The efficiency of each method varies widely. For instance, thermal power plants can achieve efficiencies closer to 45%, while hydroelectric systems may exceed 90%.
- Environmental Impact: From emissions associated with fossil fuels to the ecological impacts of damming rivers for hydroelectric projects, the environmental footprint of electricity generation is significant.
- Technological Innovations: Technologies such as carbon capture in thermal plants or advancements in photovoltaic systems for solar power are influencing future efficiency and sustainability.
Significance of the Research
This exploration is of utmost importance as it sheds light on the options we, as a society, have in transitioning toward more sustainable models of energy generation.
In a world where climate change is looming large, understanding the mechanisms that drive electricity production helps lay the groundwork for informed decisions and policies that can lead to greener alternatives.
"Electricity generation is more than just supply; it shapes how societies progress and respond to environmental challenges."
Original Research Articles
Summary of the Article
In this analysis, we will meticulously detail the inner workings of various electricity generation methods. Each section aims to dissect specific technical principles while also considering historical developments and future trends.
Author Contributions
In crafting this comprehensive guide, team contributions include research into technical specifications, case studies of operational power plants, and peer-reviewed literature to substantiate our findings.
By assembling a trove of resources, we hope to provide a nuanced overview that captures the critical aspects of power generation processes.
Intro to Power Generation
Electricity generation is a cornerstone of modern civilization, underpinning nearly every facet of daily life. Understanding the mechanisms of this process is more than an academic exercise; it is about grasping how our world functions and evolves. Without the synergy of technology and nature, we wouldn't have the means to power our homes, businesses, and cities.
The importance of power generation lies not just in the act of creating electricity but in the various systems and methods employed to harness energy effectively. Each form of power generation comes with its unique set of benefits, environmental impacts, and technological considerations. As energy demands escalate, the way we produce and use electricity must adapt to become more efficient and sustainable.
Key elements include:
- Diverse Energy Sources: Ranging from fossil fuels to renewables, understanding these sources allows for better energy mix strategies.
- Technological Innovations: Advances in technology not only improve efficiency but also pave the way for cleaner energy options.
- Economic Factors: The costing of electricity generation impacts everything from utility prices to international energy policies.
To fully appreciate the role of power generation in today’s society, one must consider how it has evolved and the challenges it faces in the quest for sustainable energy solutions. The confluence of energy needs and environmental consciousness leads us to a crucial understanding of how we generate power, ultimately shaping the landscape of global energy use.
Defining Electricity and Energy
Electricity, in its simplest form, is the flow of electric charge. Created by the movement of electrons, it is a versatile and widely used form of energy. On the other hand, energy itself is the capacity to do work, encompassing various forms including thermal, kinetic, potential, and electrical.
In practical terms, understanding the distinction between electricity and energy helps clarify the processes involved in generation. For instance, while electricity is a product generated from energy transformation, it is energy in its own right, just in a different form. This inherent relationship defines much of the discourse surrounding power plants and their operation.
The Role of Power Plants
Power plants serve as the linchpins of electricity generation systems. These facilities convert various forms of energy into electrical energy using distinct technologies based on the source of energy harnessed. The role of power plants extends beyond mere generation; they also manage transmission and ensure that electricity is distributed efficiently across grids.
There are multiple types of power plants, each playing a crucial role in meeting energy demands:
- Thermal Power Plants: Utilize fossil fuels or biomass to produce steam, which in turn drives turbines that generate electricity.
- Hydroelectric Power Plants: Use flowing water to turn turbines directly, leveraging gravitational potential energy.
- Nuclear Power Plants: Rely on nuclear reactions to produce heat, generating steam that powers turbines.
- Renewable Energy Plants: Harness natural processes like sunlight or wind to create electricity sustainably.
By understanding the operations and contributions of various types of power plants, we can better appreciate their importance in maintaining societal functions, ranging from the mundane—like keeping our lights on—to the monumental task of navigating a transition to more sustainable energy practices.
Types of Power Plants
The types of power plants play a critical role in the overall discussion of electricity generation. Understanding these various types helps clarify how different methods of energy conversion contribute to our electrical grid. Each type comes with its unique set of advantages, drawbacks, and operational considerations. Identifying the characteristics of these power plants also aids in comprehending the broader implications for energy policy, sustainability, and environmental impacts.
Thermal Power Plants
Thermal power plants, often the backbone of electricity generation, harness heat energy primarily from the combustion of fossil fuels like coal, natural gas, and oil. The basic principle involves burning fuel to create steam, which turns turbines connected to generators. This conversion of heat to mechanical energy is fundamental in linking thermal processes to electrical output.
One of the significant benefits of thermal plants is their ability to generate large amounts of electricity relatively quickly, meeting sudden increases in demand. However, they come with various considerations. For example, the emissions from burning fossil fuels raise environmental concerns, making it crucial to explore cleaner alternatives.
A critical aspect of thermal plants is their thermal efficiency, which varies based on the technology used, with modern combined cycle plants often achieving higher efficiencies due to advanced heat recovery systems.
Hydroelectric Power Plants
Hydroelectric power plants represent another important segment of the electricity generation landscape. These plants use flowing water, typically from rivers or dams, to drive turbines. The fundamental mechanics here are straightforward: as water flows, it has kinetic energy, which is transformed into mechanical energy to generate electricity.
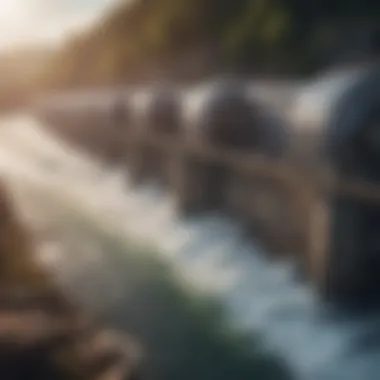
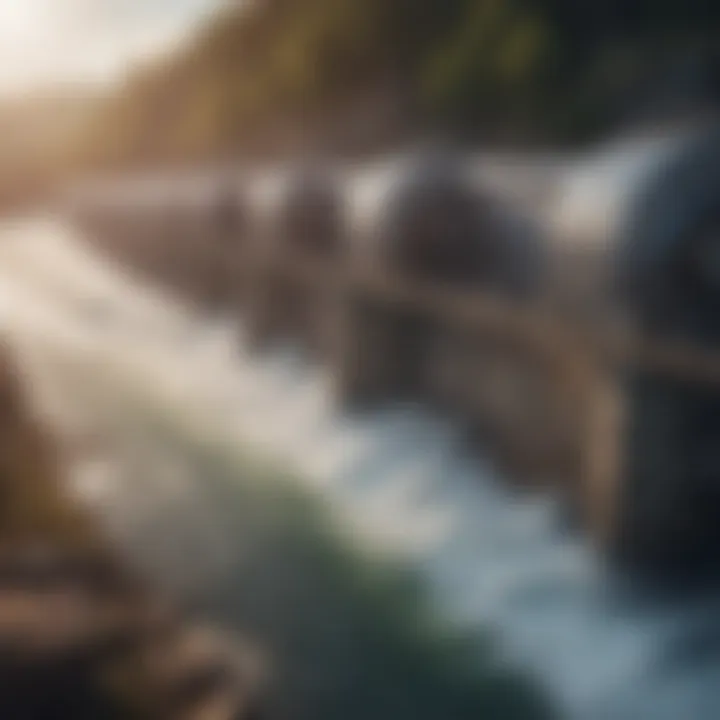
The advantages of hydroelectric power include its reliability and low operational costs once the infrastructure is in place. Additionally, hydroelectric power is renewable and has a much lower environmental impact compared to fossil fuels. Yet, the initial construction of dams can disrupt local ecosystems and communities.
Nuclear Power Plants
Nuclear power plants take a different approach to electricity generation by harnessing the energy released during nuclear fission. In this process, the nuclei of heavy elements, typically uranium or plutonium, are split to release substantial amounts of heat. This heat is then used to produce steam that turns turbines.
Nuclear energy has the advantage of being incredibly efficient, often producing large-scale energy with minimal greenhouse gas emissions. However, concerns about radioactive waste, the potential for accidents, and the high costs of building and maintaining nuclear facilities cannot be overlooked. Furthermore, the long-term management of nuclear waste presents a considerable challenge.
Renewable Energy Plants
Renewable energy plants encompass a wide range of technologies, including solar, wind, and geothermal. These plants capitalize on endless energy resources, hence supporting long-term sustainability.
Solar energy plants typically utilize photovoltaic cells to convert sunlight directly into electricity, while wind energy harnesses the power of moving air through large turbines. Each renewable source has its own merits—solar is highly scalable and can be integrated into residential areas, while wind energy boasts incredible efficiency in areas with strong winds.
Despite their advantages, renewable energy plants face challenges such as intermittent energy supply and high initial installation costs. However, advancements in technology and energy storage systems, like batteries, are helping to mitigate these challenges, making renewables a promising avenue for the future of power generation.
Energy Conversion Mechanisms
Understanding energy conversion mechanisms is pivotal in grasping how electricity is generated in power plants. This section will explore different processes that transform various forms of energy into electrical energy. Each method has its own unique characteristics, benefits, and challenges. This is essential for developing a holistic view of energy generation and optimizing efficiency across the board.
From Fuel to Energy
Combustion Processes
Combustion processes are fundamental in converting fossil fuels into energy. This method primarily relies on igniting fuels like coal, natural gas, or oil, triggering a chemical reaction that releases heat. A crucial aspect of combustion is that it’s relatively straightforward to implement and has been a reliable choice for many decades. Additionally, the high energy output makes it an attractive option for large-scale electricity generation, typically found in thermal power plants.
However, the combustion method does come with certain drawbacks. A significant con is that it produces greenhouse gases, notably carbon dioxide, which contributes to climate change. Moreover, the dependence on non-renewable resources raises sustainability concerns in a world increasingly pushing for cleaner energy alternatives.
Heat Exchange
Heat exchange processes play an equally vital role in the energy conversion equation. They facilitate the transfer of thermal energy from one medium to another, usually from the hot exhaust gases in thermal power plants to water, which gets heated to produce steam. This steam drives turbines to generate electricity. Key features of heat exchange processes include their efficiency in maximizing the energy derived from fuel combustion.
However, an essential factor to keep in mind is that these systems require careful regulation and maintenance. One unique feature of heat exchangers is their potential to reclaim waste heat, which can enhance overall efficiency. On the flip side, they can be expensive to install and maintain, necessitating upfront investment that can deter some plant operators.
Hydraulic Energy Transformation
Turbine Mechanics
When it comes to hydraulic energy, turbine mechanics are at the forefront. Turbines convert the kinetic energy from moving water into rotational energy, which then generates electricity. The simplicity and effectiveness of this approach make turbines a mainstay in hydroelectric power plants. A notable aspect is that turbines can be designed in various ways, such as Francis or Kaplan types, to suit different water flow conditions.
While turbine mechanics bring many advantages, they are not without their issues. One downside is that their efficiency can be influenced by variable water levels, sometimes leading to reduced output. Also, environmental concerns regarding water flow alterations and ecological balance can pose challenges.
Water Flow Dynamics
Water flow dynamics focus on how water moves within a hydroelectric system, critical in determining energy production. The rate and direction of water flow directly impact how effectively turbines can harness energy. A vital feature here is that optimal flow conditions can greatly enhance electricity generation.
However, conditions fluctuate due to weather, seasonal changes, or human activity, causing uncertainties in energy output. An important point to consider is the balance between maximizing energy generation and ensuring ecological sustainability, as alterations in flow can affect local ecosystems.
Nuclear Fission Process
Chain Reactions
Nuclear fission involves the splitting of heavy atomic nuclei—such as uranium or plutonium—during a chain reaction. This process releases a tremendous amount of energy. A defining characteristic of chain reactions is that they can maintain a steady flow of energy once initiated, making them highly efficient in producing electricity.
Still, nuclear energy carries significant challenges. The primary concern is the handling of radioactive waste, which poses long-term storage and safety issues. Additionally, the potential for catastrophic failures, as seen in historical accidents, demands rigorous safeguards and regulations, which add to operational costs.
Heat Generation
Heat generation from nuclear fission is another crucial element in electricity production. The intense heat produced from fission is used to convert water into steam, driving turbines for electricity generation. A notable aspect of this method is its ability to offer a continuous power supply, often termed base load power, crucial for grid stability.
However, a significant drawback is the high financial investment required up front for plant construction and safety measures. Moreover, public perception and regulatory hurdles can be considerable, restricting new projects even as established plants continue to function.
Harnessing Wind and Solar Energy
Photovoltaic Cells
Photovoltaic cells are becoming increasingly popular in converting sunlight directly into electricity. This method relies on the photovoltaic effect, generating direct current when exposed to sunlight. A key characteristic here is their potential for scalability, from small residential setups to large solar farms.
Despite their advantages, photovoltaic cells have limitations. The main concern is their dependence on sunlight, necessitating energy storage solutions for nighttime or cloudy days, which can complicate energy planning.
Wind Turbine Operation
Wind turbine operation relies on converting kinetic energy from wind into mechanical energy. These turbines can operate in various wind conditions, making them a versatile energy solution. A crucial advantage is that wind energy is renewable and produces no emissions during operation.
Nevertheless, some challenges exist, such as noise generation and the impact on local wildlife, particularly birds. The variability of wind means that energy generation can be unpredictable, necessitating a balanced approach alongside other energy sources.
Electrical Generation Techniques
Electrical generation techniques are at the core of how we harness energy from various resources. Understanding this segment is essential as it lays the foundation for comprehending the numerous methods used to convert energy into electricity. These techniques not only influence the efficiency of power generation but also have profound impacts on the environment, infrastructure, and economic factors surrounding energy production. Unpacking these techniques allows readers to appreciate different technologies and the factors influencing their deployment in today’s power landscape.
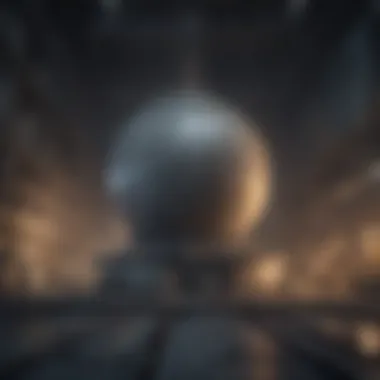
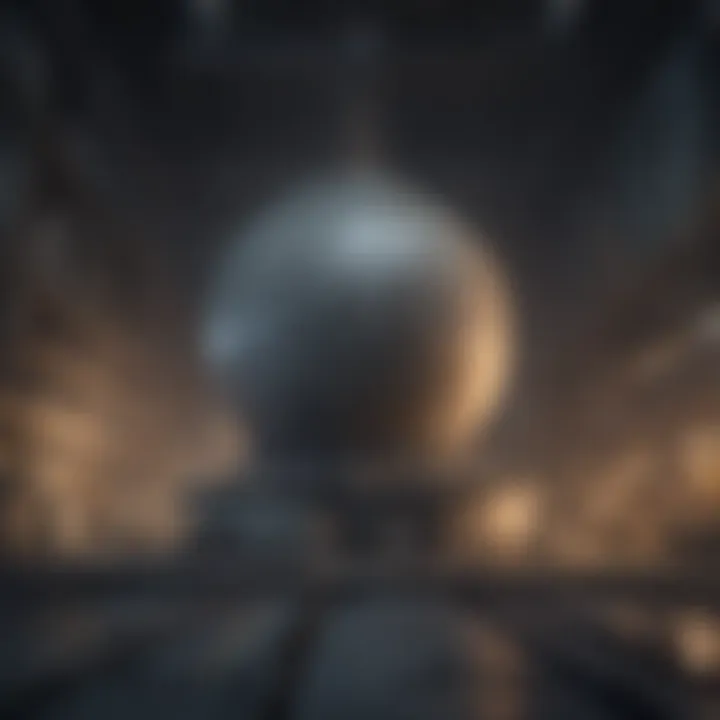
Electromagnetic Induction
Principles of Generation
At the heart of most electricity generation systems lies the principle of electromagnetic induction. This concept was pioneered by Michael Faraday and essentially states that a changing magnetic field can induce an electric current in a conductor. One compelling aspect of this principle is its versatility; it can be applied in various settings, from traditional thermal and hydroelectric plants to modern wind generators. The key characteristic of this principle is its high adaptability, making it a preferred choice in electricity generation.
The induction process itself is pretty straightforward yet quite powerful. As conductors—such as copper wires—move through a magnetic field or when the magnetic field around them alters, an electric current is produced. This not only saves time in resource utilization but also optimizes the output.
However, it does have its disadvantages, primarily concerning efficiency. Factors like resistance losses in the conductors can affect overall performance, requiring advancements such as better materials or hybrid systems to maximize output. Still, the principle’s fundamental role in voltage generation is irrefutable.
Role of Generators
Generators play an integral part in the broader context of electrical generation. Primarily, they convert mechanical energy into electrical energy based on the principle of electromagnetic induction. A remarkable feature of generators is their scalability. They can range from small portable units to massive installations that supply power to cities.
The versatility of generators is summed up in their ability to work alongside various power generation methods. Whether driven by steam, water, or wind, these devices transform energy into forms usable for daily consumption. Plus, they contribute significantly to the efficiency of the electrical generation process.
Nevertheless, they are not without their drawbacks. For one, maintenance costs can pile up, especially for large generators, where wear and tear can become a costly affair. Furthermore, the initial investment for setting up high-capacity generators may deter smaller producers. Nonetheless, their unique capability to convert energy for widespread use makes them indispensable within our energy infrastructure.
Role of Turbines
Types of Turbines
Turbines serve as vital components in the electricity generation matrix, transforming kinetic energy into mechanical energy, which is then converted to electrical energy. They come in various designs, including steam, gas, and water turbines. A significant highlight of turbines is their efficiency and adaptability across different energy forms, making them effective for generating electricity from different resources.
Each type of turbine has its unique operational characteristics and is chosen based on several factors including energy source, operational scale, and specific energy needs. For instance, steam turbines might be the go-to choice in thermal power plants, where high pressure and temperature maximize efficiency. Conversely, water turbines shine in hydroelectric power stations due to their exceptional capacity to leverage flowing water.
On the downside, the complexity of turbine design can make individual units expensive to manufacture and install. In addition, they can also be quite sensitive to operational changes, necessitating regular maintenance to prevent outages.
Energy Extraction Efficiency
Energy extraction efficiency is a crucial component in assessing the performance of turbines and their overall contribution to power generation. It encompasses how effectively a turbine converts the available energy in motion to deliver usable electrical output. This efficiency varies significantly across different turbine types and designs.
One major characteristic influencing energy extraction efficiency is blade design and aerodynamics. Advances in technology have led to the development of more sophisticated blades that maximize energy capture, even under suboptimal conditions. The unique feature of these high-efficiency turbines is their ability to operate across a broad range of conditions—something that is increasingly important as energy resource availability changes.
However, achieving high energy extraction efficiency comes with challenges, often involving higher initial costs or complex designs that require specialized maintenance. Despite this, the benefits are clear; better efficiency translates to less waste and more reliable energy production, making it a worthy investment for any energy producer.
Grid Integration and Distribution
Grid integration and distribution is a critical area of focus in electricity generation. It serves as the backbone of modern energy systems, acting as the bridge between power plants and end users. The effectiveness of this integration directly impacts reliability, efficiency, and sustainability of energy supply.
In essence, the integration of generated power into a grid allows for widespread distribution across different regions. It helps ensure balanced load management, reducing the risk of outages during peak demand times. More importantly, modern grids can accommodate various types of energy sources, including renewables, thereby promoting a cleaner energy profile.
Power Transmission Systems
Transmission Lines
Transmission lines are the highways for electricity, carrying high-voltage power from generation sites to substations. The key characteristic of these lines is their ability to transmit electricity over long distances with minimal losses. This aspect becomes particularly relevant as more remote locations harness energy generation methods, such as wind and solar, which aren't always near urban centers.
A unique feature of transmission lines is their capability to operate at high voltages, which reduce the current flowing through them. This is beneficial because lower currents minimize energy losses due to heat. However, higher voltages can also pose challenges, including the need for more robust infrastructure and greater safety measures owing to the risks associated with electrocution.
Substation Functions
Substations play a pivotal role in transforming high-voltage electricity from transmission lines to lower voltages suitable for distribution. The key characteristic of substations lies in their function as control centers within the power grid, managing voltage levels and routing power effectively. With substations, voltage regulation becomes possible, ensuring stability in supply.
One unique feature of substations is the integration of various systems, including transformers, circuit breakers, and monitoring equipment. This integration allows for real-time adjustments in response to demand fluctuations. However, substations can incur high upkeep costs and require careful siting due to space and noise considerations in residential areas.
Load Balancing
Importance of Balance
Load balancing is integral to a stable power supply; it ensures that electricity generation meets consumption. The key characteristic of effective load balancing is its real-time responsiveness to fluctuations in energy demand, which helps maintain system stability. Without proper balance, the grid risks disruption, leading to blackouts or equipment damage.
The unique feature here is the ability to forecast demand accurately using historical data and demand-side management techniques. This allows grid operators to tweak generation outputs timely. However, achieving perfect load balance is challenging due to unpredictability in energy consumption patterns, requiring ongoing adjustments and an adaptive strategy.
Demand Response Strategies
Demand response strategies are proactive measures to adjust consumer demand for electricity in response to supply conditions. They are vital for maintaining power balance, particularly during peak times or extraordinary events. The key characteristic of these strategies is flexibility; they enable consumers to reduce or shift their energy usage based on signals from the grid operators.
A unique feature of demand response is its ability to harness consumer behaviors and preferences. This helps alleviate stress on the grid and can lead to cost savings for consumers and reduced need for additional power generation. Nevertheless, implementing these strategies can be complex and requires technological support for real-time communications and consumer engagement.
In summary, understanding grid integration and distribution, along with power transmission systems and load balancing, is crucial for appreciating how electricity generation connects to everyday life. This knowledge aids in recognizing the complexities of our energy landscape.
Efficiency and Performance Factors
In the realm of power generation, efficiency and performance stand as critical pillars that shape not just the effectiveness of energy production but also its sustainability and cost-effectiveness. Understanding how electricity is generated in power plants necessitates a close look at specific elements such as thermal efficiency, environmental impact, and advancements in technology. Efficiency metrics provide essential insights that inform decision-making for upgrades, development strategies, and regulatory compliance.
Thermal Efficiency Calculations
Thermal efficiency is a fundamental concept in evaluating how well a power plant converts fuel into usable energy. At its core, thermal efficiency calculations are about comparing the energy output of a power plant to the energy input in fuel form. This relationship is pivotal, as it determines how much of the fuel consumed is being effectively used to generate electricity.
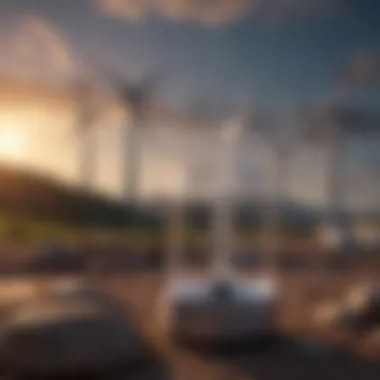
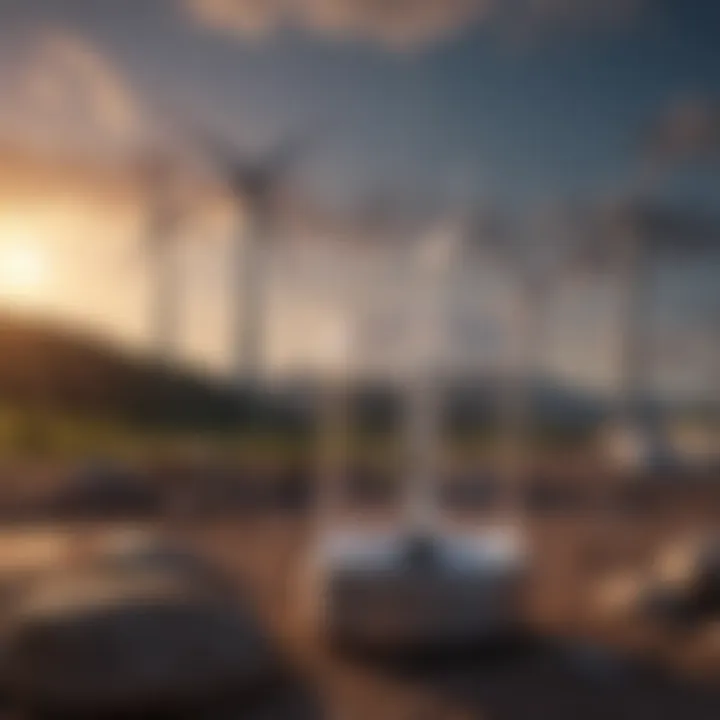
Input-Output Analysis
Input-output analysis focuses specifically on measuring the energy inputs against the outputs over a given period. It effectively shines a light on the operational performance of a power plant. By charting how much energy is consumed versus how much electricity is produced, one can identify inefficiencies and areas for improvement. A standout characteristic here is its clear, quantitative approach, making complex energy dynamics digestible and actionable.
The unique merit of input-output analysis lies in its tangible performance metrics which can be directly correlated to energy costs. This aids in comprehensively assessing operational flaws or anomalies within the system, leading to informed decisions about equipment upgrades or process enhancements. However, this method also faces limitations such as simplifications that might overlook certain variables like energy losses in transmission.
Heat Rate Evaluation
Another crucial tool in evaluating thermal efficiency is the heat rate evaluation. The heat rate indicates the amount of fuel energy needed to produce a specific amount of electricity, often expressed in British thermal units (BTUs) per kilowatt-hour (kWh). This is significant because it allows for comparison not just across different plants but also helps in tracking performance improvements over time.
One particular aspect that makes heat rate evaluation popular is its direct link between operational practices and fuel cost management. Operators can strategically use this data to optimize their processes, reducing operational expenses. However, like many assessments, it has its downsides; variations may occur based on changing operational conditions or fuel types.
Environmental Considerations
With increasing focus on sustainability, environmental considerations have become more paramount in electricity generation. The impact power generation has on the environment cannot be ignored, especially in the context of climate change and ecological preservation. As regulations evolve, embracing environmental metrics becomes a necessary step for power plants to maintain compliance and public support.
Emissions Standards
Emissions standards serve as benchmarks for the allowable discharge of pollutants created during the power generation process. These standards not only impact the design and operation of a power plant but they also dictate the kinds of technologies utilized for pollution control. Regulatory bodies enforce these standards to curb environmental degradation and protect public health.
The significant characteristic of emissions standards lies in how they guide industries towards cleaner technologies. By understanding and complying with these standards, operators can improve their strategies for emissions reduction. However, the challenge remains that achieving compliance often requires significant investments in technology and process redesign, which may be cost-prohibitive for some facilities.
Impact on Ecosystems
Finally, one must consider the impact on ecosystems when discussing electricity generation. Power plants, especially those that rely on water or land resources, can affect local wildlife and vegetation. The conclusion drawn here is that the ecological ramifications of energy production are far-reaching, necessitating comprehensive assessments that factor in all components of ecosystems involved.
Understanding this impact is vital for any power generation endeavor. A strong characteristic of evaluating ecological effects is its interdisciplinary nature, allowing collaboration across ecological, technological, and social domains. Unique to this approach is the need to balance energy needs with conservation efforts, which can lead to innovative solutions but also presents challenges when priorities conflict.
"Efficiency is doing things right; effectiveness is doing the right things." - Peter Drucker
In summary, delving into the efficiency and performance factors related to electricity generation reveals a complex but crucial narrative. From thermal efficiency calculations to robust environmental considerations, every aspect offers a unique lens through which one can appreciate the intricacies of power plants and their broader implications for society.
Future of Power Generation
The future of power generation is not just a trend; it’s an essential evolution in how we think about and utilize energy. With global demands rising and environmental challenges becoming more pressing, it's important to stay tuned to the developments shaping this landscape. The mention of technology advancements and efficiency gains that can be achieved through new methods are at the forefront of this discussion. Moreover, taking stock of these emerging trends leads us to consider both the opportunities they present and the challenges they pose.
Advancements in Technology
Smart Grids
Smart grids represent a leap toward integrating technology with traditional power generation methods. At their core, smart grids utilize digital communication technology to identify and react to local changes in usage. This is one key charactesitic that sets them apart from traditional grid systems. They enable a two-way communication interface between the utility and the consumers, which can significantly enhance overall efficiency.
One unique feature of smart grids is their capacity to incorporate distributed energy resources. This capacity allows them to manage energy generated from small-scale renewables, such as solar panels on homes or wind turbines in rural areas. The benefits of this integration are profound, enhancing energy sustainability and resilience, while also reducing reliance on fossil fuels. However, adapting to these innovations can also present challenges in terms of infrastructure and costs for immediate implementation.
Battery Storage Innovations
Battery storage innovations serve as another pillar of future energy solutions. These systems store excess energy produced during peak generation periods, making it available during times of high demand or low generation. The hallmark of these technologies is their ability to level out energy supply and demand, thereby increasing reliability across the power grid.
One notable characteristic of battery storage is how it can support renewable energy integration, reducing intermittency issues often faced with solar and wind power. The unique ability to store energy on a large scale makes these innovations increasingly essential. However, there are concerns around the sourcing of materials and the environmental impact of battery production and disposal.
Challenges Ahead
Despite the promising advancements, challenges loom on the horizon that must be addressed to ensure a smooth transition to future power generation methods.
Resource Sustainability
Resource sustainability focuses on ensuring that our energy sources do not deplete or cause irreparable harm to our ecosystem. This is important not only for maintaining energy availability but also for protecting the environment. One key aspect that makes this topic essential is that sustainable practices can lead to long-term cost savings and energy independence.
Unique in this discussion is how resource sustainability intertwines with social responsibility, driving the conversation beyond just technical viability. However, achieving this balance often faces hurdles, including economic factors and legislative frameworks that may not yet favor such shifts in energy production methods.
Climate Change Impacts
The impacts of climate change pose a significant risk to power generation systems globally. Shifts in weather patterns can have a direct effect on energy production, especially for renewables like wind and solar, which depend heavily on specific environmental conditions. A crucial characteristic of understanding climate change impacts is that they compel the industry to adapt quickly.
The unique feature of focusing on this aspect lies in how it encourages power generation methods to incorporate resilience into their planning and operation. Overall, addressing these impacts can drive innovation; nonetheless, it often requires substantial investment and cooperation across sectors, which can be a heavy lift in the current climate.
Therefore, the future of power generation is a balancing act between embracing innovation while navigating the multifaceted challenges that lie ahead.
End
The examination of electricity generation mechanisms in power plants has taught us a plethora about the complex yet fascinating processes that transform various forms of energy into electrical power. This conclusion serves to summarize significant insights while underscoring the relevance of comprehending these mechanisms in today’s fast-paced and energy-dependent world.
Summary of Key Points
To begin with, it is essential to recapitulate the core concepts discussed throughout this article:
- Types of Power Plants: We explored thermal, hydroelectric, nuclear, and renewable energy plants, emphasizing each type's unique operational mechanisms.
- Energy Conversion Mechanisms: This highlighted how different energy forms, from fuel combustion to wind and solar radiation, undergo conversion into electric energy.
- Electrical Generation Techniques: The importance of electromagnetic induction and turbine functioning was elucidated, focusing on how they drive the generation of electricity.
- Grid Integration and Distribution: Understanding how power transmission systems work alongside effective load balancing was critical to grasping how generated electricity reaches consumers.
- Efficiency and Performance Factors: We took a peek at thermal efficiency and environmental considerations, pointing out the balance required between energy generation and ecological impacts.
- Future of Power Generation: Lastly, the discussion brought us to advancements in technology and the challenges facing the energy sector as we move closer to sustainable practices.
The Importance of Understanding Power Generation
Having a firm grasp on how power generation operates is of paramount importance for multiple stakeholders, including students, researchers, and professionals in the energy domain.
- Informed Decision-Making: For policymakers and scientists, understanding these mechanisms enables informed decisions about energy infrastructure and the development of cleaner technologies.
- Inspiring Innovation: Knowledge of current technologies and their efficiency helps inspire innovations that can significantly reduce environmental footprints in energy generation.
- Educational Value: For students and newcomers to the field, a clear perspective on electricity generation mechanisms lays a solid foundation for future studies or careers in energy and environmental sciences.
In summation, understanding the mechanisms behind electricity generation is not merely academic; it holds real-world implications for the future of energy, sustainability, and the planet. When we are well aware of how energy originates and how we can improve upon that process, we take critical steps towards a cleaner, more efficient future.