Energy Dispersive X-ray Spectroscopy: Principles and Applications
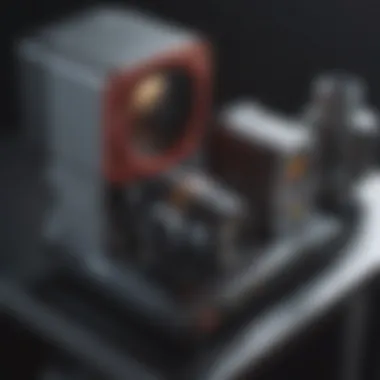
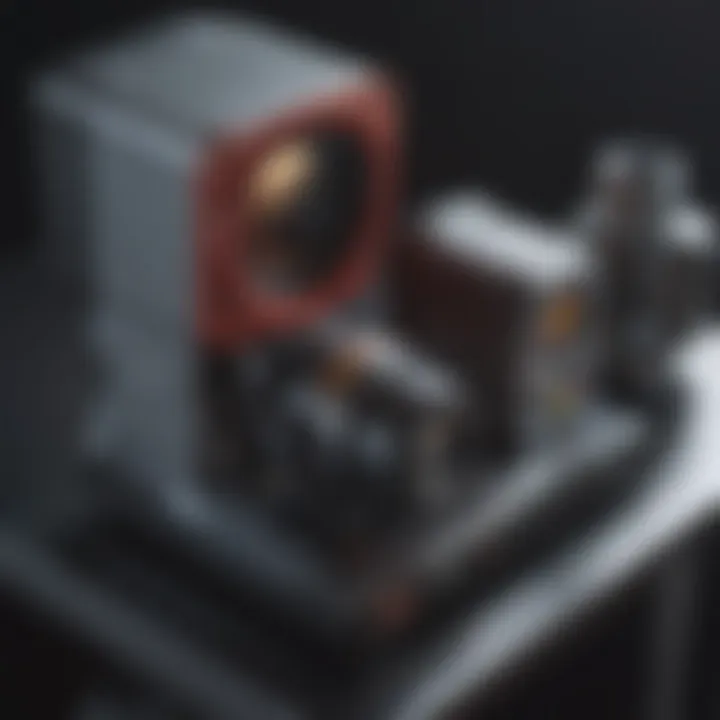
Intro
Energy Dispersive X-ray Spectroscopy (EDX) is an essential tool used in numerous fields like materials science, geology, and biochemistry. This technique stands out due to its ability to provide elemental composition data in a quick and precise manner. By employing X-ray emission as a medium for analysis, EDX enables researchers to understand the material properties at both the micro and nanoscale. The relevance of EDX cannot be understated; it bridges the gap between qualitative and quantitative analysis. Researchers increasingly depend on EDX not just for identifying materials but also for advancing material innovation.
The core of this article is to unpack the principles and applications of EDX while also examining its instrumentation. It outlines how EDX operates and elaborates on the advantages and limitations that it presents. As we dive into various applications of EDX across distinct scientific disciplines, a focus will be on current technological advancements. We will also touch on the future possibilities and challenges that lie ahead for EDX in research and practice.
In the sections that follow, we will delve deeper into the intricacies of this versatile technique, revealing its significance in contemporary research. Let us begin by examining the key findings surrounding EDX.
Prologue to Energy Dispersive X-ray Spectroscopy
Energy Dispersive X-ray Spectroscopy (EDX) is a critical method for analyzing the elemental composition of materials. It offers unique insights into materials at the micro and nano levels, which is particularly important in research and industry. As scientific inquiries progress into smaller scales, the importance of precise analytical techniques like EDX increases. This article will delve into the essential principles, applications, and advancements surrounding EDX, aiming to enhance understanding among students, researchers, and professionals.
Definition and Purpose
Energy Dispersive X-ray Spectroscopy is a technique that identifies elements in a sample by quantifying the X-ray emission resultant from material excitation. When samples are bombarded with high-energy electrons, they emit characteristic X-rays unique to each element present. The primary purpose of EDX is to determine and analyze the elemental composition of materials, which aids in understanding their structure and properties. Especially in material science, EDX helps provide not just qualitative but also quantitative data essential for characterizing diverse substances.
Historical Context
The development of EDX was a significant phase in analytical chemistry and has roots predating the modern form. The technique gained early momentum in the mid-20th century, coinciding with advancements in electron microscopy. Researchers began to recognize the potential of combining electron beams with X-ray analysis. This synergy paved the way for modern EDX systems, refining detection capabilities.
As technology evolved, EDX emerged as a versatile tool, transcending its initial scope. From its initial use in materials science to applications within biology and environmental studies, EDX has become indispensable in fields requiring detailed material characterization. Understanding this historical context is crucial to appreciate its evolution and current position in scientific research.
"Energy Dispersive X-ray Spectroscopy has transformed our understanding of material composition and behavior, offering pivotal insights that were previously unattainable."
The journey of EDX represents a cumulative effort of individuals and research communities over decades, contributing to the tool we recognize today. With the ongoing technological advancements, the potential for EDX continues to grow, making it a topic worth discussing in-depth.
Underlying Principles of EDX
Understanding the underlying principles of Energy Dispersive X-ray Spectroscopy (EDX) is crucial for grasping the full scope of this analytical technique. EDX plays a direct role in material analysis by revealing the elemental composition of samples, which is vital in various scientific applications.
X-ray Generation and Interaction
The generation of X-rays is a fundamental aspect of EDX. In EDX systems, X-rays are produced through the interaction of high-energy electrons with a target material, often metallic. When the electron beam strikes the sample, it causes the ejection of inner-shell electrons in the atoms of the target material. This process results in the formation of vacancies in the inner shells, which are typically filled by electrons from higher energy levels. The transition of these electrons between energy levels releases energy in the form of X-rays.
The energy of these X-rays corresponds to specific elements present in the sample. Therefore, each element emits characteristic X-rays that can be detected and analyzed. This interaction not only allows for qualitative analysis but also for quantitative assessment when calibrated properly. The fine control over conditions in EDX setups enhances the ability to analyze diverse materials, from metals to organic compounds.
Detection Mechanisms
Detection mechanisms are a pivotal component in EDX functionality. Upon emission, the characteristic X-rays travel to a detector, where they are converted into electrical signals for analysis. Common types of detectors include Silicon Drift Detectors (SDDs) and Energy Dispersive X-ray Detectors (EDX). These devices are designed to measure the energy of incoming X-rays.
When an incident X-ray is absorbed within the detector, it produces electron-hole pairs. The number of these pairs is directly related to the energy of the X-ray photon. The detectors analyze this information and generate a spectrum that displays peaks corresponding to the different elements within the sample. Each peak's intensity is proportional to the amount of the element present, allowing for quantitative analysis.
"Accuracy in elemental detection through EDX is significantly influenced by the choice of detector and sample preparation."
Both X-ray generation and detection mechanisms highlight the combination of physics and technology in EDX. Proper understanding of these principles is essential for researchers and professionals involved in materials science, nanotechnology, and environmental studies. Through the effective use of EDX, one can gain insights into the intricacies of a sample's composition and improve the outcomes of various industrial and scientific processes.
Instrumentation and Setup
The instrumentation and setup of Energy Dispersive X-ray Spectroscopy (EDX) are crucial foundations for obtaining accurate and reliable data. Understanding the specifications of the instruments and the proper setup ensures effective elemental analysis. This section delves into the essential elements of EDX systems and sample preparation techniques that are pivotal for high-quality results.
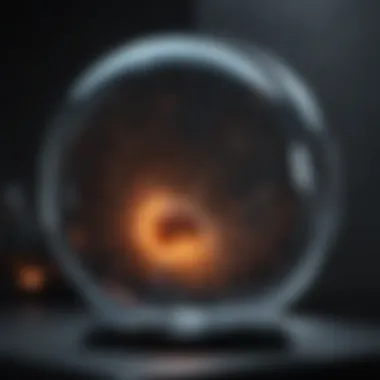
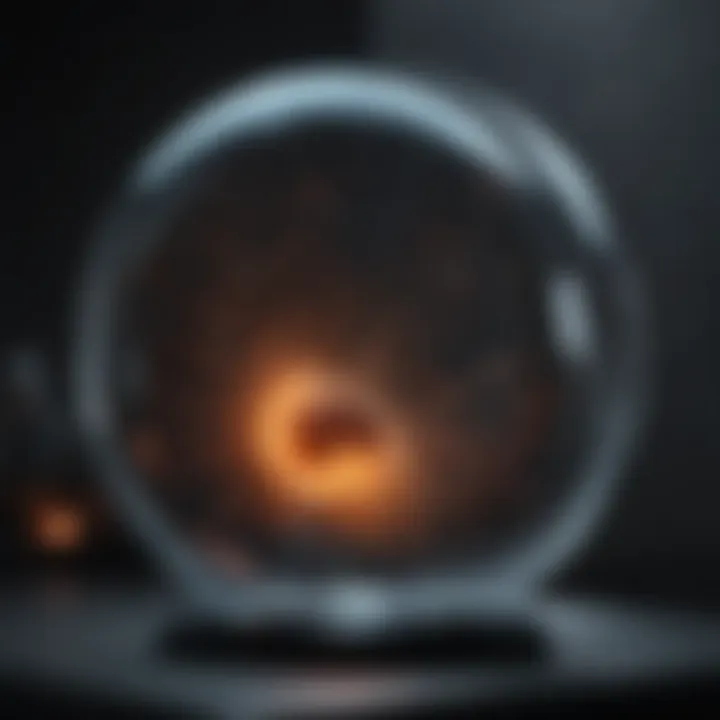
EDX Systems Overview
EDX systems primarily consist of an X-ray source, a sample holder, and a detector. The X-ray source often uses an electron beam, typically produced by a scanning electron microscope (SEM) or a transmission electron microscope (TEM). This beam interacts with the sample, generating X-rays that are specific to the elements within the material.
Since EDX relies on the detection of these characteristic X-rays, the detector plays a vital role. Various types of detectors are available, with silicon drift detectors (SDD) being widely used due to their high resolution and rapid analysis capabilities. The versatility of EDX systems allows for analysis in different modes, such as point analysis, mapping, or line scans, offering flexibility depending on the research objectives.
Setting up these instruments involves careful calibration to accurately correlate the detected energy peaks with specific elements. Consideration must be given to the sampleβs surface characteristics, as topography can influence the emission and detection of X-rays. Overall, a well-configured EDX system is fundamental for achieving reliable quantitative analysis in research and industry.
An EDX system requires proper calibration and knowledge of the sample's physical properties for optimal functionality.
Sample Preparation Techniques
Sample preparation is another critical step in EDX analysis. The primary goal is to obtain a surface that is smooth and free from contaminants. Techniques may vary considerably depending on the nature of the material being analyzed.
For solid samples, polishing is often essential. This can involve grinding the surface using abrasives and polishing compounds to achieve the desired finish. Sometimes, this process is followed by etching to reveal surface features and composition information.
For powders, the samples generally need to be compacted into pellets to ensure a uniform layer. This minimizes issues related to particle size and density that could affect the X-ray interaction.
Biological samples require even more careful handling. Often, they undergo fixation and dehydration before being embedded in a resin. This process preserves the sample while allowing for effective EDX analysis.
Quantitative Analysis in EDX
Quantitative analysis is a core aspect of Energy Dispersive X-ray Spectroscopy (EDX), crucial for determining the elemental composition of materials. This method does not merely identify the presence of elements, but also quantifies their respective concentrations, allowing for a detailed understanding of material properties. The precision and efficiency of quantitative analysis in EDX significantly contribute to its application in various fields, such as materials science, archaeology, and environmental science.
A key benefit of quantitative analysis using EDX is its ability to deliver timely results in a non-destructive manner. This is especially beneficial when analyzing precious or unique samples where sample integrity is paramount. Additionally, the straightforward nature of EDX instrumentation allows rapid analysis, which is essential for high-throughput studies or quality control processes in manufacturing.
However, there are specific considerations associated with accurate quantification. The calibration methods used to standardize the results are essential. These methods play a vital role in ensuring that the detected signals correlate accurately to the actual elemental concentrations. Therefore, robust calibration processes must be established and periodically revisited to maintain the reliability of the analysis.
Another significant aspect is the influence of matrix effects, which can impact signal intensity. The presence of other elements in the sample can absorb or enhance the X-ray signals emitted, thus altering the apparent concentration of elements being analyzed. Proper corrections and adjustments must be made to account for these effects to ensure accurate results.
"Quantitative analysis in EDX is not just about identifying elements, but understanding the intricate balance of their quantities, which informs material properties and functionality."
Applications of EDX in Various Fields
Energy Dispersive X-ray Spectroscopy (EDX) serves as a pivotal analytical tool across numerous scientific disciplines. Its role in elemental analysis provides significant insights into material composition, making it invaluable for both research and applied science. EDX aids in understanding materials at a fundamental level, influencing how they are used in various industries. The following sections detail the specific applications that showcase the versatility and reliability of EDX technology.
Material Science
In the domain of material science, EDX plays an essential role in characterizing and analyzing materials. This includes metals, ceramics, polymers, and composites. Through EDX, researchers can identify the elemental composition of materials, which is critical for understanding the properties and behaviors of these materials under different conditions.
For instance, EDX can be employed to inspect the integrity of materials used in construction or aerospace. The determination of trace elements can also inform decisions related to material selection and quality control in manufacturing processes. Moreover, EDX facilitates comparative studies, enabling scientists to assess the impact of different compositions on the performance of materials.
Nanotechnology
Nanotechnology has emerged as a significant field driven by the unique properties of nanomaterials. EDX is crucial here, as it allows for the detailed analysis of these materials at the nanoscale. The ability to characterize the elemental composition and distribution provides insights that are necessary for ensuring the effectiveness and safety of nanomaterials in applications like drug delivery, sensors, and renewable energy solutions.
EDX helps researchers to evaluate how different elements contribute to the overall functionality of a nanomaterial. Through mapping techniques, scientists can visualize how different components interact, guiding the design of innovative nanostructures. The integration of EDX in this field not only enhances the understanding of fundamental processes but also supports the development of cutting-edge technologies.
Environmental Studies
In environmental studies, EDX is a powerful tool for assessing pollution levels and understanding the elemental composition of various environmental samples. This includes soil, water, and air samples. By utilizing EDX, researchers can identify contaminants at trace levels, which is vital for environmental remediation efforts.
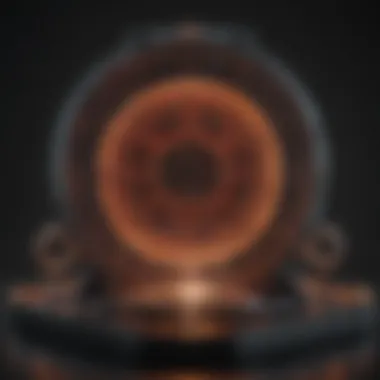
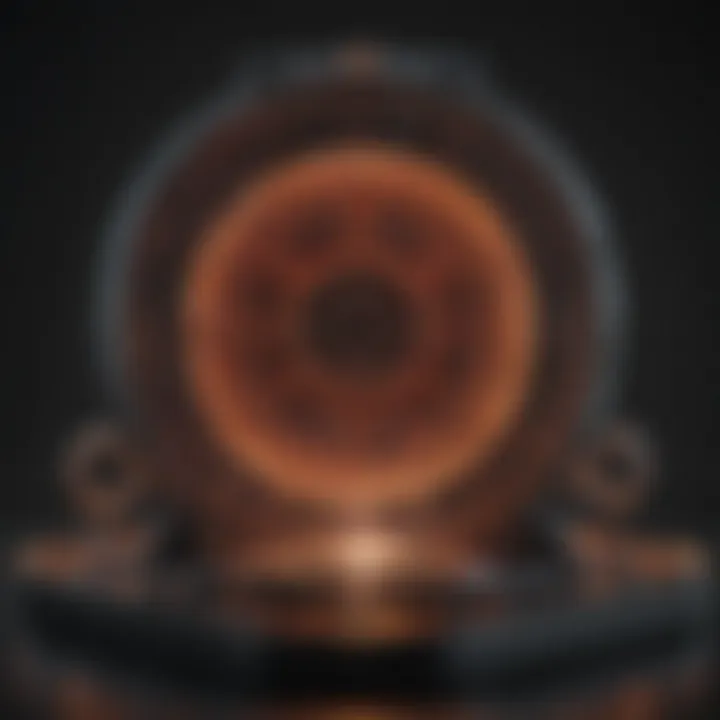
"EDX allows for the precise identification of pollutants, enabling targeted interventions for environmental cleanup."
Moreover, it supports the monitoring of heavy metals and other hazardous substances, contributing to public health and safety. Assessing soils for specific elemental content is also crucial in agriculture, ensuring the sustainability and productivity of land use.
Biological Applications
The application of EDX in biological sciences is growing, particularly in understanding cellular and tissue composition. By analyzing biological samples, researchers can uncover important details about the presence and distribution of elements essential for life, such as calcium, phosphorus, and trace metals.
In histology, for example, EDX can provide insight into the mineralization of tissues, leading to a better understanding of certain diseases. Additionally, studying the elemental composition of biological samples leads to advancements in biotechnology and medicine, influencing areas such as diagnostics and therapeutic development.
In summary, the diverse applications of EDX across multiple fields illustrate its significance in advancing knowledge and technology. From material science to environmental studies and biology, EDX continues to provide invaluable insights that drive innovation and improvement.
Advantages of EDX
Energy Dispersive X-ray Spectroscopy (EDX) offers several advantages that significantly enhance its utility in various scientific fields. Understanding these benefits is crucial for anyone engaged in elemental analysis, material characterization, or related research. The following explores the specific advantages that EDX presents.
Speed and Efficiency
One of the primary strengths of EDX is its speed. The technique provides rapid results, often in just a few minutes. This capability is essential in high-throughput environments, such as industrial quality control and materials research. Researchers can quickly analyze samples without extensive delay, which promotes productivity.
EDX systems typically operate with minimal sample preparation. This factor not only saves time but also reduces the risk of contamination, which can compromise results. The efficiency of this method allows scientists to conduct multiple analyses within a short time, optimizing the overall workflow. In practical applications, this means that both routine inspections and more detailed research can be accomplished swiftly.
Non-Destructive Analysis
Another significant advantage of EDX is its non-destructive nature. The technique allows for the analysis of materials without altering their composition or structure. This characteristic is particularly important in fields where sample integrity is vital, such as archaeology or art conservation, where artifacts must not be damaged during study.
Non-destructive analysis enables repeated measurements on the same sample. Researchers can explore changes over time or compare different techniques without the fear of losing sample material. Given these aspects, EDX stands out in its ability to facilitate both comprehensive studies and preservation efforts.
"The non-destructive property of EDX opens pathways in research fields where conservation is paramount, matching modern analytical needs with essential preservation ethics."
In summary, the speed and efficiency of EDX, along with its non-destructive analysis capabilities, make it a highly valuable tool for scientists and engineers. These advantages allow for quick data acquisition and the preservation of vital specimens, translating to broad applications across disciplines.
Limitations and Challenges
Understanding the limitations and challenges of Energy Dispersive X-ray Spectroscopy (EDX) is crucial for properly utilizing this technique. While EDX offers many advantages, such as rapid analysis and non-destructive testing, several factors can hinder its performance. Awareness of these limitations can help researchers and practitioners mitigate issues and enhance the quality of their results.
Elemental Detection Limits
One significant limitation of EDX is its elemental detection limits. Each element has a specific threshold concentration below which it becomes challenging to detect accurately. Generally, heavier elements are detected more easily than lighter ones. For instance, elements like lead or gold can be identified at low concentrations, while lighter elements like lithium or beryllium require substantially higher amounts for reliable detection. This discrepancy can limit the effectiveness of EDX in characterizing materials that contain lighter elements in trace quantities.
Moreover, the matrix of the sample can affect detection limits. A complex matrix can potentially obscure the spectral lines of the element being analyzed. Thus, in heterogeneous samples or those with significant background noise, interpretation becomes even more complicated. Researchers must take careful considerations during planning to optimize sample preparation, making sure appropriate standards are in place.
Interpretation of Complex Spectra
Interpreting complex spectra remains a challenge in EDX analysis. The resulting spectra can exhibit multiple overlapping peaks, particularly when various elements are present in a sample. These overlaps complicate the identification and quantification of specific elements. In some cases, the presence of compounds or phases can further obscure the spectral signals, making analysis even more challenging.
To improve the accuracy of interpretation, advanced software tools are often employed. These tools can help deconvolute overlapping peaks and provide more reliable analysis. However, dependence on software does not eliminate the need for skilled operators. Well-trained personnel are essential, as they can apply contextual knowledge when assessing complex scenarios.
While EDX is a powerful tool, addressing its limitations is essential for maximizing its potential. By being aware of detection limits and having the ability to interpret complex spectra, users can ensure they derive the most accurate and helpful information from their analyses.
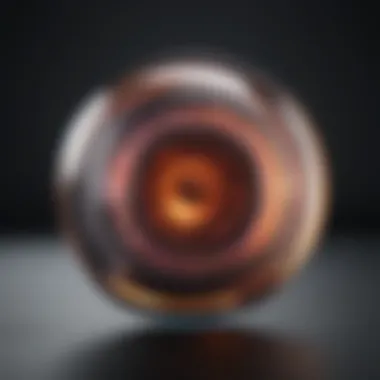
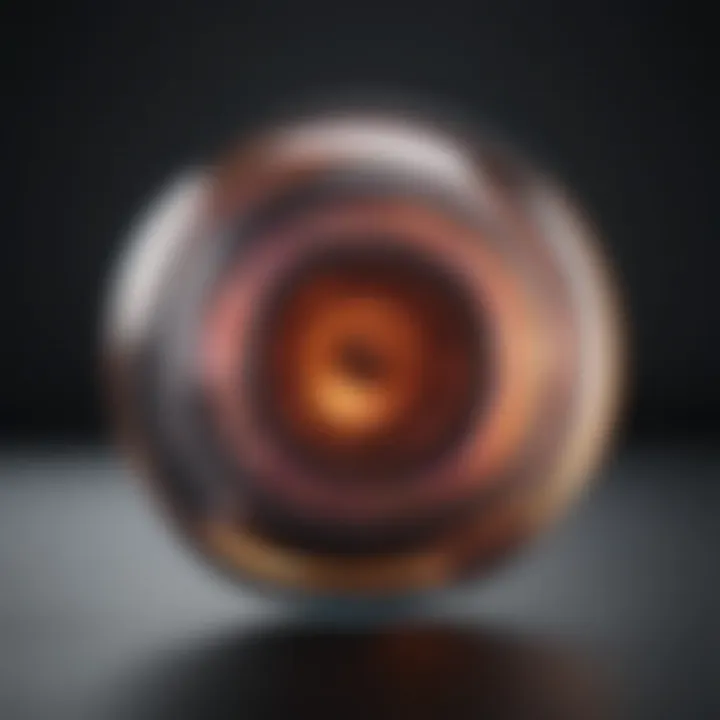
The success of EDX lies in understanding both its capabilities and its inherent limitations.
Recent Advancements in EDX Technology
Recent advancements in Energy Dispersive X-ray Spectroscopy (EDX) significantly enhance the capabilities and applications of this analytical technique. As the demand for precise elemental analysis grows across various fields, these innovations are crucial for maintaining EDX's relevance and efficacy. Improvements in technology not only allow for better detection limits but also improve the overall application scope, showing promise for future research.
Improvements in Detector Technology
One of the most noteworthy advancements in EDX technology involves detector improvements. Newer detector designs, such as silicon drift detectors (SDD), provide substantial benefits over traditional detectors. SDDs offer higher energy resolution and faster counting rates. This means that subtle variations in elemental composition can be discerned more effectively. Higher energy resolution minimizes overlap between spectral peaks, thus enhancing accuracy in identifying elements.
In addition, modern detectors exhibit increased sensitivity to low-energy X-rays. This sensitivity is vital when analyzing materials where lighter elements are of interest. Using these advanced detectors, researchers can obtain a more comprehensive understanding of materials at micro and nanoscales, contributing to better material characterization.
Integration with Other Techniques
The integration of EDX with other analytical techniques is also a remarkable trend shaping the future of elemental analysis. For instance, coupling EDX with scanning electron microscopy (SEM) allows for precise spatial mapping of elemental distributions. SEM provides high-resolution images of material surfaces, while EDX analyzes elemental composition in those regions. This powerful combination allows researchers to draw deeper insights into material properties and behaviors.
Moreover, the integration of EDX with techniques like X-ray diffraction (XRD) facilitates the study of crystalline structures alongside elemental analysis. These interdisciplinary approaches enable a fuller understanding of the interactions and properties of various materials. Through effective collaboration among different analytical methods, the realms of characterization expand, yielding more robust data for researchers.
Future Directions in EDX Research
The field of Energy Dispersive X-ray Spectroscopy (EDX) is poised for significant growth and innovation. As technological advancements continue to reshape scientific research, EDX remains critical in various applications. Understanding future directions in EDX can provide insights into the potential benefits and considerations for scientists and researchers.
Emerging Applications
EDX has traditionally been used in materials science, but its applications are expanding rapidly. Emerging fields such as nanotechnology and biomedicine are beginning to exploit the unique capabilities of EDX.
- Nanomaterials Analysis: As nanotechnology evolves, the demand for precise analysis of nanoparticles is increasing. EDX can be employed to characterize the elemental composition of nanoparticles, aiding in the development of new materials with enhanced properties.
- Environmental Monitoring: Another promising application is in environmental studies. By assessing soil and water samples, EDX can help identify contaminant compositions. This is crucial for assessing pollution sources and developing remediation strategies.
Moreover, the integration of EDX with advanced imaging techniques, such as electron microscopy, presents new opportunities. Combining these techniques allows for a more comprehensive understanding of material interactions and properties at the nanoscale.
Interdisciplinary Collaborations
Interdisciplinary collaboration is vital for advancing EDX research. By working together, professionals from various fields can bring new perspectives and innovations.
- Materials Science and Chemistry: Chemists and material scientists can work jointly to develop new synthesis methods that enhance the capabilities of EDX. This collaboration can lead to the creation of novel materials that possess unique characteristics.
- Biology and Medicine: In the biomedical field, interdisciplinary approaches can improve the understanding of biomaterials and tissue engineering. EDX can identify elemental distributions in biological samples, furthering the study of how materials interact with biological systems.
These collaborations often lead to the development of new methods and applications, further enhancing the versatility of EDX in research.
The continual evolution of EDX technology positions it as an indispensable tool in modern scientific research, making it essential for scientists to stay informed about future trends and methodologies.
Finale
The conclusion of this article encapsulates the importance and relevance of Energy Dispersive X-ray Spectroscopy (EDX) within various fields of study. EDX is not merely an analytical tool; it is an essential technique that contributes to a deeper understanding of material properties and compositions. The discussion highlights several critical elements, including its efficiency in elemental characterization, the non-destructive nature of the analysis, and the ability to provide high spatial resolution information. These factors make EDX a preferred choice for researchers and professionals alike.
Summary of Key Points
In reviewing the previous sections, certain key points stand out about EDX:
- Principles and Mechanisms: Understanding the basic principles of X-ray generation, detection, and interactions aids in grasping how EDX functions.
- Applications: The versatility of EDX is demonstrated across disciplines such as material science, nanotechnology, and environmental studies, showing its vital role in research and development.
- Advantages over Other Techniques: The swift analysis time, coupled with the non-destructive method of sample examination, positions EDX as a valuable asset in laboratories.
- Limitations: Awareness of its limitations, such as elemental detection limits and complexity in data interpretation, is equally crucial for effective application.
- Future Directions: Emerging applications and collaborations indicate that EDX will evolve as technology progresses, leading to enhanced capabilities and broader usage.
The Role of EDX in Scientific Research
Energy Dispersive X-ray Spectroscopy plays a pivotal role in scientific research. It enables researchers to perform precise elemental analyses on a micro and nano scale, significantly advancing teaching, experimentation, and product development. The ability to combine EDX with other techniques enhances its utility, leading to more comprehensive datasets.
Moreover, EDX promotes interdisciplinary research collaboration. By providing valuable compositional data, it assists researchers from various fields such as biology, material science, and environmental studies in tackling complex problems. As research continues to push boundaries, EDX's adaptability will ensure its relevance in future studies.
EDX is not just a tool; it is a bridge connecting diverse scientific inquiries through its elemental insight.