Exploring the Complexities of APS Physiology
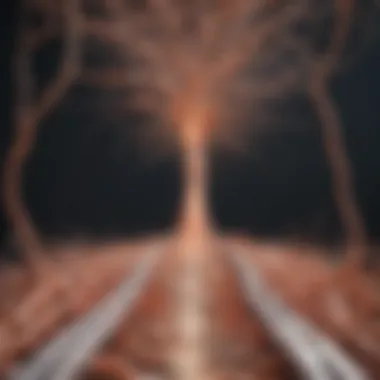
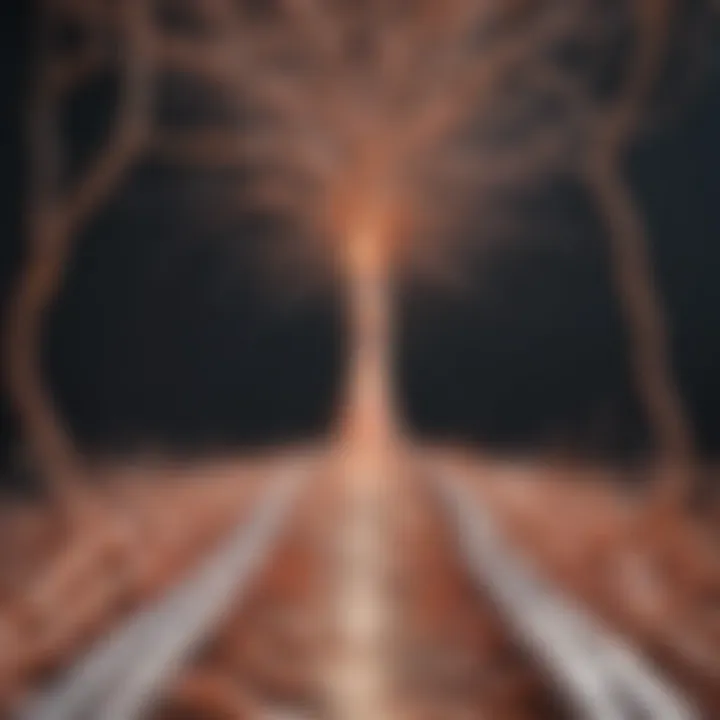
Intro
The APS is divided into two main components: the sympathetic nervous system and the parasympathetic nervous system. Each component has unique functions but works together to maintain balance in bodily functions. Analyzing these systems helps to clarify their regulatory roles in health and disease.
In this article, we will dive deep into key aspects of APS physiology by highlighting research findings and discussing their implications for health and disease. We intend to provide clarity about the autonomic nervous system's role both in typical physiological processes and in pathological states. Understanding these dynamics can lead to better insights into conditions ranging from anxiety disorders to cardiovascular diseases.
As we progress, the article will cover the major mechanisms involved in APS, their pathways, and how they adapt in different situations. This aim is not only to educate but also to foster a thorough appreciation for the complexity of autonomic regulation by providing a scientifically rich yet accessible discussion.
Let's begin with an overview of significant research highlights that illuminate our understanding of APS physiology.
Prelims to APS Physiology
Understanding the autonomic nervous system (ANS) requires careful consideration of its physiological aspects. APS physiology is significant because it holds the keys to understanding how our body manages processes without our conscious input. From heartbeat regulation to digestive functions, the autonomic system significantly impacts overall health. By studying these processes, it becomes clear why disruptions in this system can lead to various health issues.
When exploring APS physiology, it is vital to recognize the interconnected nature of its components. The sympathetic and parasympathetic nervous systems, as key elements, work in opposition to maintain a delicate balance in the body. Similarly, the enteric nervous system operates within the gastrointestinal tract, connecting emotional state and digestive health. This article delves into not only the mechanics of these systems but also the broader implications for health and disease.
In addition, the historical perspective provides insights into how our understanding of APS physiology has evolved. Over the decades, research has unveiled intricate networks and functionalities previously unknown. Understanding the growth of this field can illuminate current research trends and future directions. Thus, this section will explore these foundational elements that are crucial to grasping APS physiology.
Components of the Autonomic Nervous System
The autonomic nervous system (ANS) plays a critical role in regulating involuntary physiological processes in the body, including heart rate, blood pressure, respiration, digestion, and sexual arousal. Understanding the components of the ANS is pivotal for grasping how these systems interact to maintain balance, a concept known as homeostasis. Each component of the ANS has unique features and functions that contribute to its overall impact on bodily functions. This section delves into the sympathetic nervous system, parasympathetic nervous system, and enteric nervous system, elucidating their structure, function, and significance.
Sympathetic Nervous System
Structure and Function
The sympathetic nervous system (SNS) is part of the autonomic nervous system that prepares the body for stressful or emergency situations. Its structural layout consists of a series of ganglia positioned close to the spinal cord, known as the sympathetic trunk. This organization allows for a rapid response by coordinating multiple organs at once, thus facilitating a comprehensive physiological change. Remarkably, the SNS activates the body's 'fight-or-flight' response, which is crucial for survival during perceived threats.
One of the key characteristics of the SNS is its extensive neural connections. These connections enable the SNS to exert widespread effects across various systems, leading to increased heart rate, dilation of pupils, and redistribution of blood flow towards muscles. This feature supports the body's immediate response to danger. However, the SNS can contribute to negative health outcomes if activated too frequently, leading to heightened stress and anxiety.
Neurotransmitters
The SNS primarily utilizes norepinephrine as its main neurotransmitter, which is released from postganglionic neurons. Norepinephrine's role is essential, as it binds to adrenergic receptors, resulting in the physiological changes associated with the fight-or-flight response. This neurotransmitter's effectiveness is one of the reasons why it is a popular focus in studies of stress and response mechanisms.
A unique aspect of norepinephrine is its ability to influence both alpha and beta receptors, each producing different physiological effects. This versatility allows for fine-tuning of the body's response based on the situation. However, the reliance on this singular neurotransmitter could be seen as a disadvantage in scenarios where a broader range of signaling is necessary for appropriate modulation.
Physiological Effects
The physiological effects mediated by the SNS are numerous and significant. Primary responses include increased heart rate, heightened blood pressure, and enhanced metabolic rate. These changes are necessary for preparing the body for immediate physical activity. The SNS also influences gastrointestinal motility, decreasing it to conserve energy for crucial bodily systems.
The hallmark of these physiological effects relies on rapid mobilization of energy resources. However, while these responses are beneficial in acute situations, chronic activation of the SNS can lead to health disorders, such as hypertension and chronic stress. Thus, understanding the balance between activation and avoidance of frequent stimulation is crucial for health maintenance.
Parasympathetic Nervous System
Role in Homeostasis
The parasympathetic nervous system (PNS) is another vital component of the ANS, primarily responsible for conserving energy and promoting a state of rest and digestion. Its activation leads to bodily functions that occur during restful states, such as stimulating digestion and decreasing heart rate, contrasting the responses triggered by the sympathetic system.
A key characteristic of the PNS is its role in maintaining homeostasis. By supporting the body's relaxed state, the PNS helps restore energy levels and promotes recovery after stress. This restorative aspect makes the PNS a necessary counterpart to the sympathetic system, ensuring that the body can efficiently transition between active and calm states.
However, the unique feature of the PNS, focused on non-essential functions during while resting, might lead to vulnerabilities should the system be overactive or insufficiently engaged during periods requiring heightened alertness. Understanding this balance is vital for achieving optimal functioning.
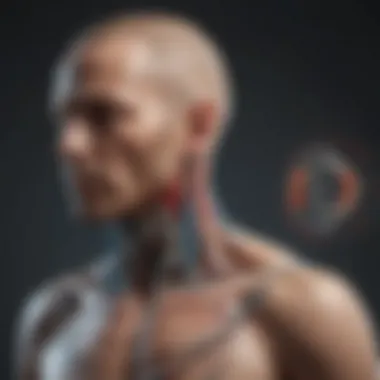
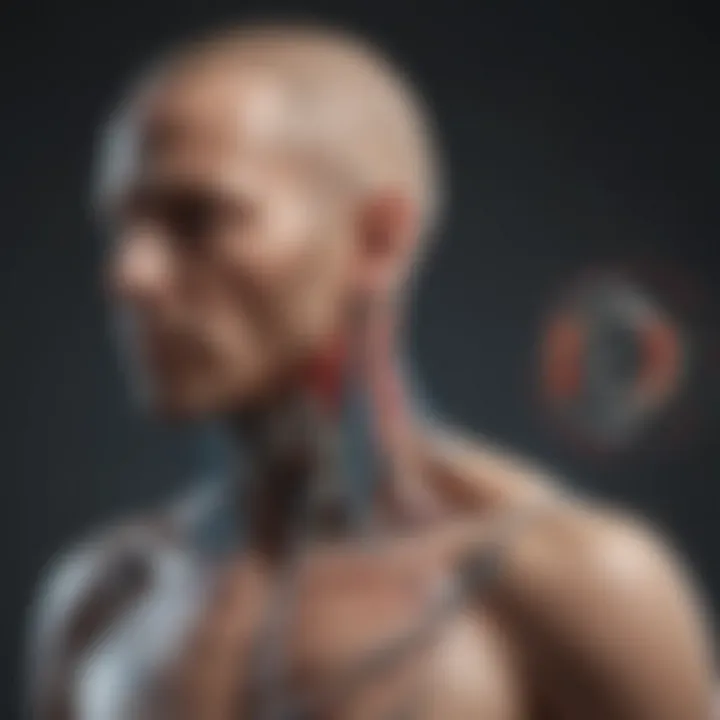
Neuroanatomy
The neuroanatomy of the PNS is characterized by long preganglionic fibers and short postganglionic fibers, in contrast to the SNS. This structure allows for localized responses, impacting specific organs rather than multiple systems at once. The vagus nerve, a significant component of the PNS, innervates major organs, bringing about changes in heart rate and digestive activity.
The localized nature of this neuroanatomy makes it beneficial for targeted physiological effects, such as stimulating salivation or slowing the heart rate. However, this specificity can be a disadvantage in emergencies when a swift, coordinated response across multiple systems is required.
Effects on Organ Systems
The effects of the PNS on organ systems are primarily restorative, enhancing digestive functions, stimulating secretion of saliva, and promoting blood flow to the gastrointestinal tract. These effects play a crucial role in nutrient absorption and overall health maintenance.
A clear advantage of the PNS is its ability to stimulate processes that support recovery and energy conservation. Yet, if overactive, it may lead to digestive issues or lower heart rates that can become problematic under certain situations.
Enteric Nervous System
Characteristics and Functions
The enteric nervous system (ENS), often referred to as the "second brain," is a complex network of neurons embedded in the walls of the gastrointestinal tract. This system regulates digestive functions independently yet works closely with both the sympathetic and parasympathetic systems. A key characteristic of the ENS is its autonomy; it can function independently of the central nervous system, responding directly to changes in the digestive environment.
The unique feature of the ENS is its ability to coordinate complex reflexes, such as peristalsis, digestion, and secretion of enzymes. This capability allows for efficient digestion without direct oversight from the brain, demonstrating a remarkable degree of functional independence.
Interactions with Other Systems
The ENS interacts extensively with the sympathetic and parasympathetic systems, underscoring its role within the broader autonomic framework. This interaction demonstrates how the ENS influences environmental responses through the digestive process. For instance, signals from the sympathetic nervous system may inhibit gastrointestinal functions during stress, while the parasympathetic system may enhance it during restful states.
The interaction feature of the ENS emphasizes the interconnectedness of bodily systems. However, these interactions can also be disadvantageous. Complications can arise from pathological events where dysregulation leads to functional gastrointestinal disorders, highlighting the need for further research and understanding of these interactions in health and disease.
Understanding these components provides an essential foundation for exploring the autonomic nervous system's roles in various physiological processes. Each system's distinct structure and functions work in concert, ensuring both immediate responses to challenges and long-term recovery and regulation.
Neurotransmission in APS
Neurotransmission plays a critical role in the functioning of the autonomic nervous system (APS). It is the means by which signals are transmitted across synapses, allowing communication between neurons as well as between neurons and their target organs. This mechanism not only facilitates various physiological responses but also governs how the body reacts to internal and external stimuli. Understanding neurotransmission is therefore essential for grasping the full complexity of APS physiology.
Cholinergic Transmission
Cholinergic transmission is based on the release of the neurotransmitter acetylcholine. It has pivotal roles within both the central and peripheral nervous systems. In the context of the APS, this transmission is most prominently noted in the parasympathetic nervous system. Here, acetylcholine acts to decrease heart rate, enhance glandular secretion, and increase gastrointestinal motility.
Recent studies suggest that cholinergic signaling is essential for maintaining homeostasis. For instance, when an individual is resting, cholinergic transmission supports body functions like digestion and nutrient absorption. Moreover, the receptors involved, such as muscarinic and nicotinic receptors, have varying effects based on their location in the body, adding layers of complexity to the cholinergic system.
Adrenergic Transmission
Adrenergic transmission primarily involves the hormones epinephrine and norepinephrine. This system is a crucial component of the sympathetic nervous system, often associated with the body's fight-or-flight response. When faced with stressors, adrenergic transmission can lead to increased heart rate, dilated bronchial passages, and elevated blood glucose levels. These responses are achieved through adrenergic receptors, which are broadly classified into alpha and beta categories.
Understanding adrenergic transmission is not just theoretical; it has practical implications in clinical settings. Drugs that manipulate this transmission can be used to treat conditions such as hypertension and asthma. Thus, studying this aspect of neurotransmission can uncover potential therapeutic avenues that harness these pathways effectively.
Peptidergic Transmission
Peptidergic transmission involves neuropeptides, which are more complex than traditional neurotransmitters. Neuropeptides like substance P and neuropeptide Y have been shown to play roles in modulating various functions within the APS. This form of transmission often influences pain perception, stress-related responses, and eating behaviors.
One remarkable feature of peptidergic transmission is its prolonged effects compared to classic neurotransmission. Neuropeptides can bind to their receptors for an extended period, resulting in lasting changes to physiological states. This makes understanding peptidergic mechanisms particularly important for comprehending chronic conditions where signaling may be disrupted.
The complexity of APS neurotransmission demonstrates intricate networks of communication, vital for both adaptive responses and the maintenance of homeostasis.
In summary, neurotransmission within the APS encapsulates a range of mechanisms involving cholinergic, adrenergic, and peptidergic systems. Each pathway contributes uniquely to the body's overall function, and comprehending these interactions is essential for advancing our knowledge of autonomic physiology and its implications.
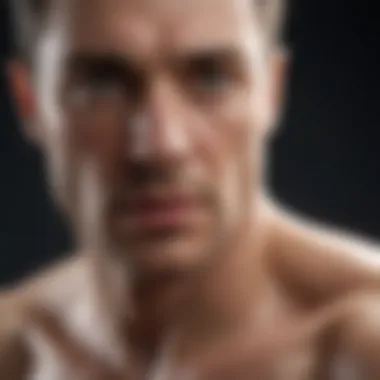
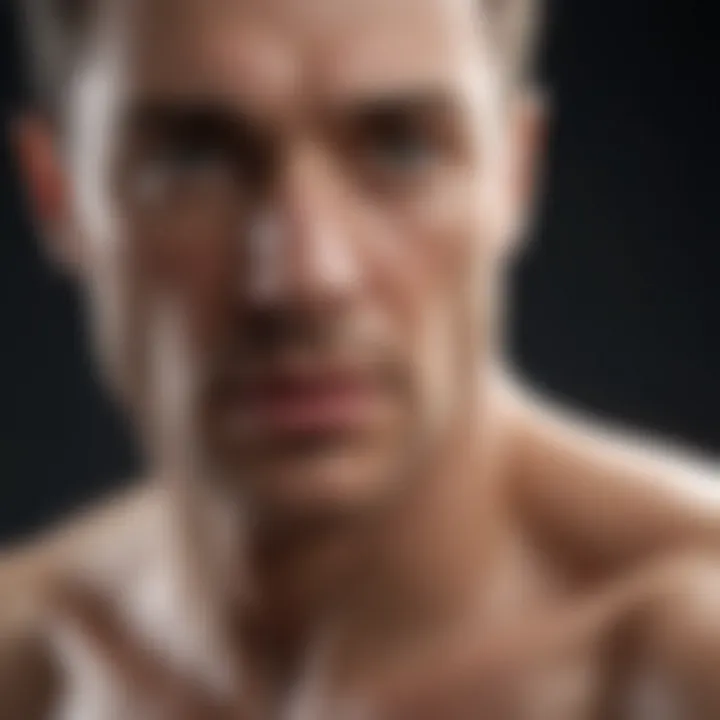
Physiological Responses Mediated by APS
The autonomic nervous system plays a critical role in the body's physiological responses. It regulates involuntary bodily functions such as heart rate, respiratory rate, and digestive processes. Understanding these responses is vital as they reflect how the body adapts to various stressors and maintains homeostasis. This section delves into key responses mediated by the APS, focusing on their significance in health and the implications of their dysregulation.
Stress Responses
The Fight-or-Flight Response
The fight-or-flight response is perhaps one of the most well-known physiological reactions and it underscores the body's innate ability to respond to perceived threats. This mechanism engages the sympathetic nervous system, leading to immediate physiological changes such as increased heart rate, heightened alertness, and redirection of blood to essential muscles. In scenarios of stress, this response can be beneficial as it prepares a person to either confront or flee from danger. However, the unique feature of this response is its activation can be problematic when triggered excessively or inappropriately. Chronic stress can lead to long-term health concerns, including hypertension and anxiety disorders, illustrating the importance of balance in these responses.
Cortisol Release Mechanisms
Cortisol is often dubbed the "stress hormone," as its release is pertained to the body's response to stress. When a stressful event occurs, the hypothalamus triggers the pituitary gland to release adrenocorticotropic hormone, which then stimulates the adrenal glands to produce cortisol. This process aids in energy regulation. A key characteristic of cortisol release mechanisms is its dual role; it not only supports immediate energy needs but can also suppress non-critical functions like digestion and immune responses. While its acute release can be necessary, prolonged exposure raises issues such as metabolic disturbances and immune suppression. Moreover, the potential adverse effects prompt a more nuanced understanding of its role in health and disease.
Digestive Regulation
Role of the Parasympathetic System
The parasympathetic nervous system is essential for regulating digestive processes. It activates during restful states, promoting bodily functions like salivation, secretion of digestive enzymes, and gut motility. This facilitation is crucial since digestion requires energy and resources that thrives in a calm environment. The unique aspect of this system is its opposing role to the sympathetic system. By promoting relaxation, it encourages restorative processes. However, disruptions, such as high stress levels, can impede its function, leading to symptoms like indigestion or irritable bowel syndrome.
Gastrointestinal Motility
Gastrointestinal motility refers to the contractions of smooth muscle in the digestive tract that move food from the esophagus to the intestines. This process heavily relies on autonomic regulation. A key characteristic of gastrointestinal motility is its coordination through both the enteric nervous system and the autonomic nervous system. Healthy motility is vital for digestion efficiency and nutrient absorption. However, disturbances in this area—whether related to hormonal imbalance, stress, or medications—can result in conditions such as gastroparesis, causing delayed gastric emptying. Thus, acknowledging the intricate balance in motility is essential for addressing digestive health.
Heart Rate Variability
Mechanisms of Heart Rate Regulation
Heart rate regulation is a complex interplay between the sympathetic and parasympathetic systems. The balance of these components influences heart rate variability (HRV), where higher variability is generally associated with better cardiovascular health and stress resilience. One key characteristic of heart rate regulation is its responsiveness to both physical and psychological stressors. Internal cues can trigger adjustments in heart rate, indicating the body's adaptive capability. These mechanisms can be beneficial in healthy individuals; however, low HRV may signal poor autonomic function, requiring deeper examination into underlying health issues.
Clinical Significance
Clinical significance in the context of heart rate variability lies in its potential as a non-invasive biomarker. Parameters of HRV can assist healthcare providers in assessing autonomic function and overall health. Changes in HRV are linked to various conditions, such as heart disease, diabetes, and mental health disorders. The unique aspect of HRV's clinical relevance is that it provides insight into both physical and emotional health under stress. This dual perspective is vital, particularly as it can prompt the need for therapeutic interventions aimed at restoring autonomic balance.
Clinical Implications of APS Dysregulation
The autonomic nervous system (ANS) plays a critical role in maintaining homeostasis within the body. Dysregulation of the ANS can lead to significant clinical implications, resulting in a wide range of health issues. Understanding these implications is integral for identifying effective treatment strategies and improving patient outcomes. This section focuses on three major aspects of clinical implications stemming from autonomic nervous system dysregulation: autonomic neuropathy, cardiovascular disorders, and the impact on mental health.
Autonomic Neuropathy
Autonomic neuropathy refers to damage to the autonomic nerves that control involuntary bodily functions. This condition can stem from diabetes, infections, or autoimmune diseases. Symptoms of autonomic neuropathy can vary but often include:
- Orthostatic hypotension: A drop in blood pressure upon standing, causing dizziness or fainting.
- Gastroparesis: Delayed gastric emptying, leading to nausea and bloating.
- Erectile dysfunction: Impaired blood flow affecting sexual function.
The condition's impact can significantly reduce quality of life. Recognizing and addressing elements such as medication management and dietary modifications can help mitigate symptoms. Early intervention is crucial for preventing complications that arise from untreated autonomic dysfunction.
Cardiovascular Disorders
The links between autonomic dysfunction and cardiovascular health are well documented. Dysregulation of the sympathetic and parasympathetic branches can lead to severe cardiovascular disorders. Two primary aspects deserve attention:
- Heart rate abnormalities: An imbalance in autonomic regulation can lead to tachycardia or bradycardia, both of which may increase vulnerability to arrhythmias.
- Increased risk of heart disease: Chronic exposure to stressors can lead to prolonged sympathetic activation, contributing to hypertension and atherosclerosis.
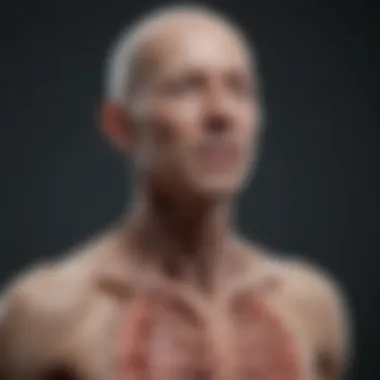
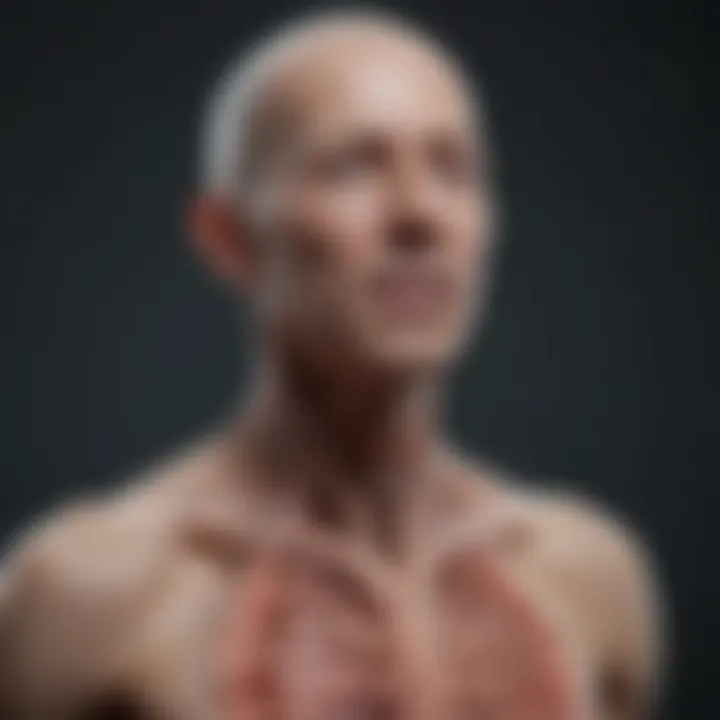
Managing cardiovascular disorders requires a multifaceted approach, including lifestyle changes, pharmacological interventions, and regular monitoring of heart function. Increasing awareness of how autonomic dysregulation contributes to cardiovascular risk can improve prevention and management strategies.
Impact on Mental Health
The interplay between the autonomic nervous system and mental health is an area of emerging interest. Dysregulated autonomic function is often linked to mental disorders. Key aspects include:
- Anxiety and depression: Both conditions display alterations in autonomic functions, such as heart rate variability. This relationship creates a feedback loop, where mental health issues exacerbate autonomic dysregulation.
- Stress response: Chronic stress can lead to sustained sympathetic activation, impacting mood and emotional regulation.
Treatment for mental health issues related to autonomic dysregulation may include psychotherapy, lifestyle modifications, and if needed, medication. Understanding the physiological underpinnings of mental health can facilitate more effective therapies.
Autonomic dysregulation can impact various bodily systems, influencing overall health outcomes.
In summary, addressing the clinical implications of ANS dysregulation is paramount. A comprehensive understanding can not only enhance patient care but also foster ongoing research into specialized treatment options. Recognizing these implications allows healthcare professionals to implement targeted strategies aimed at restoring balance in autonomic function.
Research Advances in APS Physiology
Research advances in APS physiology are crucial for understanding how the autonomic nervous system impacts various bodily functions and overall health. As we explore new findings, we gain insights that may lead to improved clinical treatments for autonomic dysfunctions. Innovation in this field is vital, as it can reveal the intricate mechanisms that underpin physiological responses in both health and disease.
New discoveries in autonomic regulation have opened new pathways for the management of several disorders. Analyzing how the autonomic nervous system interacts with other systems can help us appreciate its complexity. Recent studies highlight the potential for targeted therapies that can correct dysregulation in the autonomic pathways.
New Discoveries in Autonomic Regulation
Recent years have seen significant progress in the understanding of autonomic regulation. Researchers are focusing on how the autonomic nervous system adapts to stressors and environmental changes. One noteworthy discovery is the role of neuroplasticity in autonomic function. This adaptability can influence heart rate, blood pressure, and gastric motility.
Another significant finding involves the gut-brain axis, which has gained attention for its involvement in autonomic regulation. The interplay between brain and gastrointestinal function suggests that mental health can affect digestive health and vice versa. Researchers are now investigating how interventions in this axis could improve outcomes for conditions such as irritable bowel syndrome and functional dyspepsia.
Technological Interventions
Technology is reshaping the landscape of research in APS physiology. Devices that monitor autonomic function, such as heart rate monitors and wearable technology, are revolutionizing how we gather data. These devices offer real-time insights into physiological changes in response to different activities, providing valuable information for both patients and clinicians.
Another notable technological advancement is in the area of neurostimulation therapies. Electrical stimulation of specific pathways within the autonomic nervous system shows promise for treating conditions like hypertension and heart failure. These interventions target the sympathetic nervous system's overactivity and can lead to noticeable improvements in patient outcomes.
Future Directions for Research
The future of APS physiology research appears promising. One important area of study is the role of genetics in autonomic function. Understanding genetic predispositions to autonomic disorders could facilitate earlier diagnoses and more effective treatments. Similarly, further investigation into the molecular mechanisms underlying autonomic regulation can illuminate new therapeutic targets.
Another direction for future research is the integration of multidisciplinary approaches. Combining insights from psychology, neurology, and cardiology can enhance our understanding of how autonomic regulation affects overall health. Collaborative efforts among scientists and clinicians will likely yield innovative solutions to complex health challenges.
Closure
The conclusion serves as a culminating reflection on the vital role of APS physiology in both health and disease. By synthesizing the information from various sections of this article, we highlight critical insights into how autonomic regulation impacts physiological processes.
Summarizing Key Points
Several key points emerge from the exploration of APS physiology. First, understanding the components of the autonomic nervous system—sympathetic, parasympathetic, and enteric—is fundamental to grasping their overall influence on body functions. Each system contributes uniquely to maintaining homeostasis, adapting responses to stress, and regulating bodily functions such as digestion and heart rate.
Second, neurotransmission plays a crucial role in how the APS communicates internally and externally. Cholinergic, adrenergic, and peptidergic transmissions form an intricate web that ensures precise and appropriate physiological responses.
Third, the implications of APS dysregulation are significant. Autonomic neuropathy, cardiovascular issues, and potential mental health impacts are just a few consequences of disrupted autonomic function.
Lastly, the advances in research and technology provide hope and insights into future studies. Understanding how to manipulate these pathways could lead to new therapeutic interventions, improving the quality of life for affected individuals.
The Importance of APS Regulation in Health
Regulation of the APS is central to maintaining health. A well-functioning autonomic nervous system is essential for adapting to changing environmental demands, managing stress, and ensuring vital functions occur seamlessly.
- Homeostasis: The APS is critical in sustaining balance among bodily systems. It regulates heart rate, respiration, and vascular tone, ensuring that organs function properly.
- Mental Well-being: Evidence suggests a strong link between APS functioning and mental health. Disorders such as anxiety and depression can manifest due to autonomic dysregulation.
- Chronic Conditions: Long-term dysregulation can lead to or exacerbate chronic health issues like hypertension and diabetes. Addressing these imbalances is crucial for disease management and prevention.
In summary, understanding APS physiology is not merely academic; it is an essential component of effective healthcare strategies. Ensuring proper autonomic functioning is linked to both immediate responses to environmental challenges and long-term health outcomes.