Exploring Bio Genetic Engineering: Techniques and Impacts
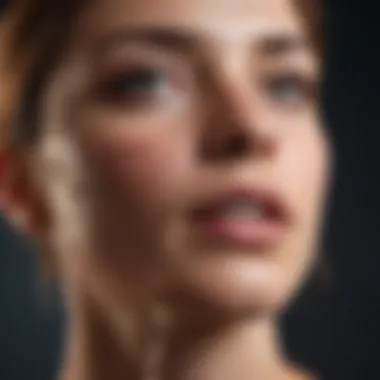
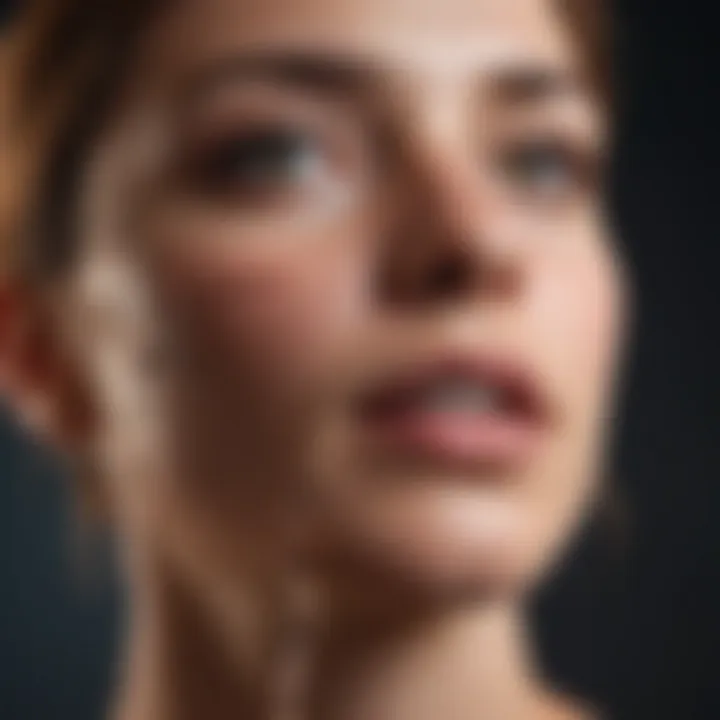
Intro
The field of bio genetic engineering is a tapestry woven with scientific ingenuity and ethical contemplation. As we venture into this domain, it becomes essential to grasp the foundational concepts that underpin genetic engineering, its historical trajectory, and the contemporary tools that drive its progress. This exploration reveals the powerful interplay between genetic manipulation and engineering, setting the stage for revolutionary advancements across various sectors.
Imagine a world where crops are engineered not just to grow faster, but to flourish in arid conditions or resist pests without the need for harmful pesticides. Envision medical therapies tailored to the genetic makeup of individual patients, offering treatments that are precisely attuned to their needs. The implications of bio genetic engineering stretch far and wide, affecting agriculture, medicine, and even our environment, creating a profound impact on society as a whole.
This article’s journey spans from the historical hallmarks of genetic research to the cutting-edge techniques like CRISPR that are currently transforming our understanding and application of genetics. As we delve deeper, we'll highlight ethical dilemmas that accompany these advancements. For instance, who determines the moral boundaries of genetic modification? What responsibility do scientists have when it comes to the potential repercussions of their work?
A discourse on bio genetic engineering is not merely academic; it beckons us to address the societal implications that emerge as technology races ahead. We must consider the delicate balance between innovation and ethical responsibility, driving the discussion toward informed policy-making to ensure our advancements serve humanity's best interests.
Thus, the forthcoming sections will be laden with insights, analysis, and a well-rounded view of a field that stands at the confluence of promise and peril.
Understanding Bio Genetic Engineering
Bio genetic engineering is a subject that sits at the crossroads of biology and technology. Its significance can’t be overstated; it intertwines fundamental biological concepts with engineering principles to create new possibilities in various fields. This section aims to unravel the layers surrounding bio genetic engineering, helping readers grasp its definition, scope, and interdisciplinary nature.
The increasing complexity of global challenges - be it in nutrition, health, or environmental sustainability - underscores the need for innovation in genetic practices. Bio genetic engineering holds the potential to address these issues head-on. Its applications can be seen in everything from genetically modified crops designed for higher yields to innovative medical therapies that target genetic diseases. Thus, understanding bio genetic engineering is not merely academic; it can lead to tangible benefits that touch the lives of many.
Moreover, as this field bounces against ethical considerations and regulatory frameworks, comprehending it will help pave the way towards informed discussions and policy-making. As students, researchers, educators, and professionals delve deeper, they will uncover a landscape rich in opportunities but also fraught with challenges, requiring careful navigation.
Definition and Scope
At its core, bio genetic engineering involves the direct manipulation of an organism's genes using biotechnology. This manipulation may involve altering sequences to enhance desirable traits, introduce new characteristics, or even knock out non-beneficial genes. The scope of bio genetic engineering is vast and varied, encompassing subfields such as synthetic biology, gene therapy, and agricultural biotechnology.
When defining bio genetic engineering, it is helpful to break it down into several key components:
- Gene Editing: Precise modifications to an organism's genetic material, which include techniques like CRISPR-Cas9.
- Genetic Modification: Broader alterations, which could involve the transfer of genes from one species to another.
- Applications: Fields impacted include agriculture (GMOs), medicine (targeted therapies), and environmental science (bioremediation).
By understanding these components, individuals gain a clearer picture of how far this field has come and where it is headed.
Interdisciplinary Nature
Bio genetic engineering is not solely the domain of geneticists. It draws knowledge from various fields including molecular biology, biochemistry, bioinformatics, and even ethics. This interdisciplinary nature is what makes bio genetic engineering a dynamic discipline.
For example:
- Molecular Biologists study the structure and function of genes, providing the foundational knowledge necessary for any engineering efforts.
- Biochemists contribute insights into metabolic pathways, which are essential for designing organisms for specific productive purposes.
- Ethicists weigh the moral implications of genetic modifications, guiding policies that govern practice.
Each area contributes its unique perspective, leading to a holistic understanding of bio genetic engineering's potential and limitations. This blending of disciplines fosters innovation while keeping the door open for ethical debates and societal considerations.
The future of bio genetic engineering hinges not only on scientific advances but also on responsible governance and public discourse.
As readers explore this article further, they will appreciate the rich tapestry of ideas that come into play when considering the implications of bio genetic engineering, forming a crucial backdrop to the discussions that follow.
Historical Context
The significance of understanding the historical context of bio genetic engineering lies in the realization that this complex field did not spring forth fully formed from the minds of scientists. Instead, it has evolved over decades, fueled by a series of breakthroughs, ideas, and even controversies. By delving into this history, one can appreciate how past discoveries shape present techniques and future applications. Each turning point has laid groundwork fostering not just scientific curiosity but also moral, ethical, and regulatory considerations that continue to influence practices today.
Early Manipulations of Genetic Material
In the annals of science, the earliest attempts to manipulate genetic material can be traced back to the late 19th and early 20th centuries. Gregor Mendel, often dubbed the father of genetics, laid vital groundwork with his experiments on pea plants, revealing the principles of inheritance. However, it wasn't until the discovery of DNA's structure in 1953 by James Watson and Francis Crick that a door opened wide for genetic exploration. Their breakthrough set in motion a wave of scientific inquiry into the molecular foundation of life.
By the 1970s, pioneers like Paul Berg were venturing into uncharted waters. Berg's creation of the first recombinant DNA molecules signaled a paradigm shift. This daring approach not only demonstrated the feasibility of combining genetic material from different organisms but also paved the way for what we know today as genetic engineering. The excitement surrounding these advancements offered a glimpse into the potential that this new science held for agriculture, medicine, and beyond.
Development of Molecular Biology
The evolution of molecular biology is a story intertwined with technological progress. As researchers began to decode the complexities of genes, tools like gel electrophoresis and polymerase chain reaction (PCR) emerged. PCR, developed by Kary Mullis in 1985, allowed scientists to amplify small segments of DNA, making it possible to analyze genes in greater detail than ever before. This technique transformed genetic research, enabling not just deeper insights into biology but also practical applications ranging from forensics to diagnostics.
With the mapping of the human genome in the early 2000s, molecular biology entered a new golden age. The project itself wasn't merely a scientific endeavor; it symbolized the collaborative spirit of the global scientific community. This initiative unveiled the immense potential of genetic data, marking a significant milestone that would expedite advancements in personalized medicine and other areas.
Emergence of Genetic Engineering Techniques
As the foundational elements of genetics and molecular biology solidified, a variety of genetic engineering techniques began to take shape. Techniques such as gene cloning and gene editing rapidly came into play. This era saw the introduction of selective breeding practices that pushed boundaries and set new standards in agricultural productivity.
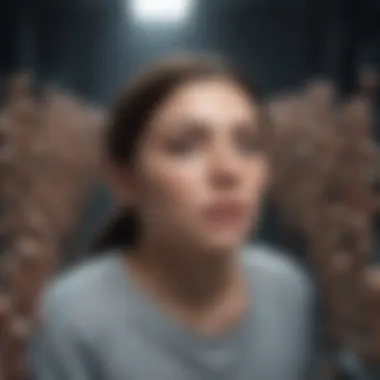
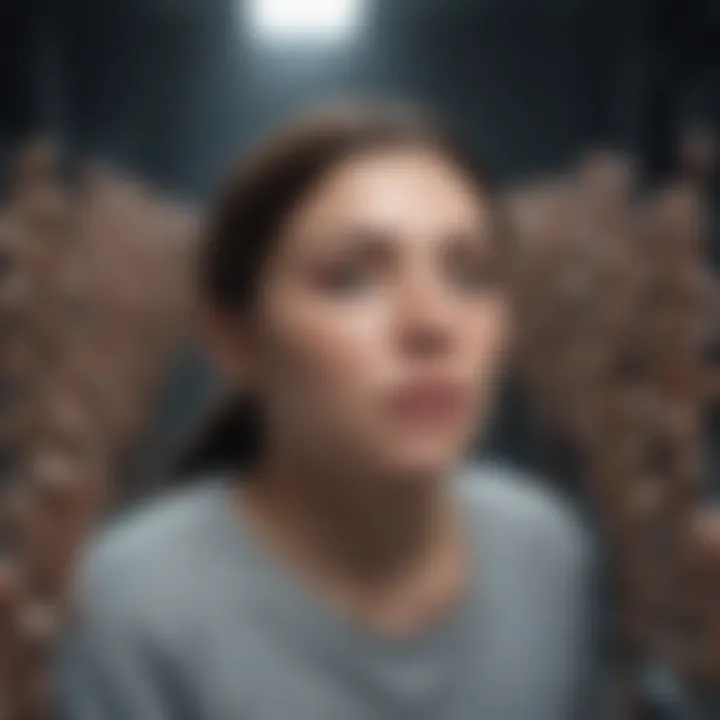
Furthermore, the advent of technologies like CRISPR-Cas9 in recent years revolutionized the landscape, allowing for unprecedented precision in gene editing. The promise of these techniques is undeniable. For instance, while traditional genetic modification might entail introducing foreign genes, CRISPR can alter, delete, or replace genes more efficiently and with fewer unintended effects.
"The history of genetic engineering is a tapestry woven with bold ideas and significant technological strides; it serves as a reminder that innovation is often born out of the curiosity to understand nature better."
In summary, the historical context of bio genetic engineering reveals how innovations cascade into one another, each building on the discoveries that came before it. Understanding this trajectory enriches the discourse surrounding current practices and potential future advancements.
Modern Techniques in Bio Genetic Engineering
In the fast-paced world of genetic engineering, modern techniques are revolutionizing how we manipulate the very fabric of life. These developments are not just scientific curiosities; they represent groundbreaking tools in enhancing agriculture, medicine, and addressing environmental challenges. As we delve deeper into these techniques, it's crucial to recognize their profound implications, benefits, and the ethical guardrails we need to consider.
CRISPR-Cas9 Technology
CRISPR-Cas9 has taken the scientific community by storm. This technology allows for precise editing of DNA sequences in living organisms. The basics of CRISPR involve a guide RNA that is programmed to locate a specific DNA sequence within the genome. Once it finds its target, the Cas9 enzyme acts like a pair of molecular scissors that cuts the DNA. This opens the door for the insertion, deletion, or alteration of genetic material at the desired site.
What makes CRISPR stand out? First, its versatility. Scientists can use it across a multitude of organisms—from bacteria to plants to mammals. This adaptability can lead to innovations, like developing crops resistant to pests without the need for harmful pesticides. In medicine, CRISPR shows promise in treating genetic disorders by fixing mutations that cause diseases.
However, with great power comes great responsibility. Ethical considerations regarding off-target effects—where CRISPR may unintentionally alter other genes—are hot topics in current debates. Ensuring safety while harnessing this technology is paramount.
Synthetic Biology Approaches
Synthetic biology takes genetic engineering a step further. It’s about not just editing existing genomes but building new biological parts. Imagine designing whole new organisms or biological systems to carry out specific functions. This could mean engineering microbes that can produce biofuels or pharmaceuticals.
This field employs several methods, including the use of bioinformatics and computational models to predict how changes will affect organisms. The foundational goal is toward greater efficiency and sustainability in industries ranging from agriculture to medicine.
Yet, the ambitious nature of synthetic biology raises eyebrows. The creation of synthetic life, even on a small scale, necessitates robust safety protocols and regulatory oversight. Questions about potential misuse and ecological impact can't simply be swept under the rug. It requires a careful balance between innovation and safeguarding the planet.
Gene Drives: Possibilities and Limitations
The concept of gene drives is both gripping and controversial. Gene drives are designed to propagate specific genes throughout a population, sometimes overriding natural selection. By increasing the chances that a particular trait will be passed on to offspring, gene drives can potentially control pests or invasive species effectively. A famous example involves engineering mosquitoes to suppress malaria transmission.
However, the excitement surrounding gene drives isn’t without its drawbacks. Such powerful tools could have unintended consequences. The ecological effects of releasing engineered organisms into the wild are unpredictable. Would they lead to the collapse of certain ecosystems? Would they affect species we hadn’t even anticipated?
"While gene drives present revolutionary solutions, they also highlight the need for conscientious scientific exploration coupled with public discourse on the implications."
Thus, as we harness modern techniques in bio genetic engineering, it’s essential to maintain a vigilant eye on both the remarkable possibilities and the pitfalls they may introduce. What lies ahead is a landscape filled with potential breakthroughs, but also ethical quandaries that demand thoughtful reflection.
Applications of Bio Genetic Engineering
The realm of bio genetic engineering offers a treasure trove of applications that are shaping various fields, from agriculture to medicine and environmental management. Each application brings its own set of advantages while also posing unique challenges and considerations. Understanding these facets is crucial not only for those directly involved in the field but also for society at large, as these innovations will likely influence the fabric of our daily lives.
Agricultural Innovations
In the agricultural sector, bio genetic engineering has made significant strides. It allows scientists to create genetically modified organisms (GMOs) that possess traits for enhanced productivity or resistance to diseases. For instance, crops such as Bt cotton and Golden Rice have been engineered for specific benefits. The former has been modified to produce a protein toxic to certain pests, reducing the need for chemical pesticides. Golden Rice, on the other hand, has been enriched with vitamin A, aiming to address nutritional deficiencies in many developing countries.
The benefits of these innovations are substantial:
- Increased yield and food security.
- Reduced environmental impact due to less reliance on chemical fertilizers.
- Ability to cultivate in less-than-ideal conditions, thus utilizing lands previously deemed unsuitable for agriculture.
However, there are also considerations to be aware of:
- The potential loss of biodiversity due to monoculture practices.
- The socio-economic implications for smallholder farmers who may struggle to access patented GM seeds.
It's essential for policymakers and stakeholders to navigate these waters carefully, weighing both the potential benefits and ethical concerns.
Medical Breakthroughs
Bio genetic engineering has revolutionized the medical field, especially in drug development and disease treatment. Techniques like gene therapy are changing the way we approach inherited diseases, allowing for targeted treatments. For instance, treatments for conditions such as cystic fibrosis and hemophilia are becoming increasingly viable through gene-editing technologies.
A remarkable application is the development of monoclonal antibodies. Chemists can engineer these antibodies to target specific cells, such as cancerous ones, leading to more effective treatments with fewer side effects compared to traditional therapies.
The positive impacts here include:
- More personaliseed medicine tailored to individual genetic profiles.
- Potential cures for previously untreatable conditions.
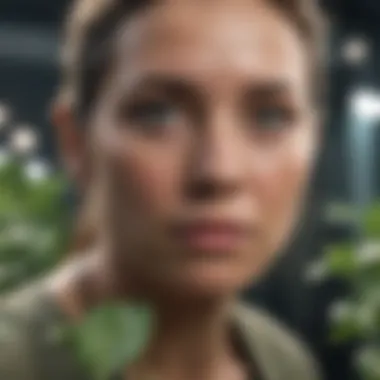
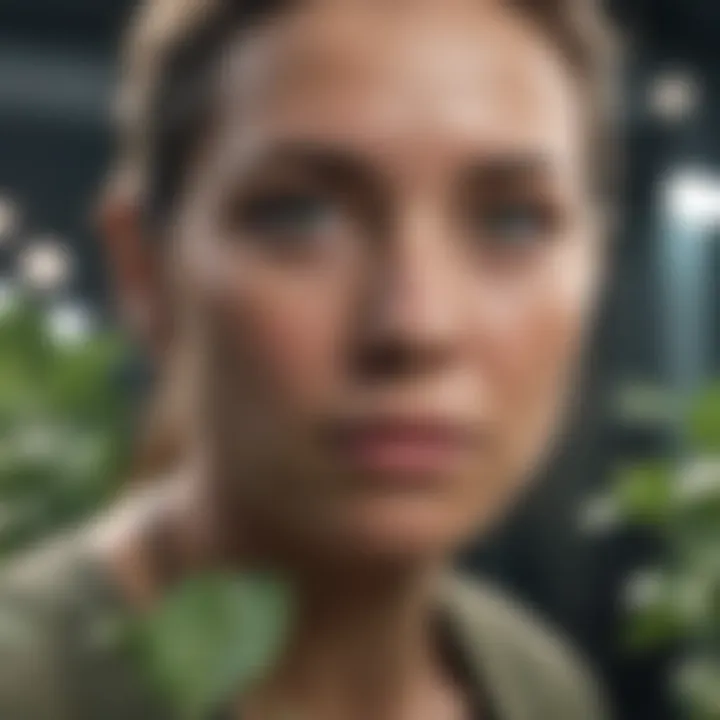
Nonetheless, there are profound ethical and regulatory hurdles that must be tackled:
- The high costs associated with gene therapies can make them inaccessible.
- Concerns over long-term effects of gene modifications on future generations.
Environmental Bioremediation
Another area where bio genetic engineering shines is in environmental bioremediation. This application involves the use of engineered organisms, such as bacteria or plants, to detoxify polluted environments. For example, genetically modified microbes can degrade oil spills or absorb heavy metals from contaminated water.
- Advantages of using bioengineered organisms include:
- However, it is crucial to weigh the risks involved:
- Cost-effectiveness compared to traditional cleanup methods.
- Speedier recovery of contaminated sites.
- The possible unintentional spread of GMOs into natural ecosystems.
- The need for strict regulatory measures to monitor engineered organisms.
"Bio genetic engineering is not just a tool; it is a bridge for our future, connecting innovation with sustainable practices."
Ethical Considerations
Ethical considerations are paramount in the realm of bio genetic engineering. This field is not simply a technical pursuit; it embodies profound questions about the moral responsibilities scientists hold. As we delve into the manipulation of genetic materials, we must grapple with the implications of such power and its impact on living organisms, human health, and the environment.
A key element in this discourse is the moral implications of genetic modifications. The ability to design and modify organisms brings forth questions like, Should we play God? This reflects concerns about the extent to which humanity should intervene in natural processes. For instance, creating genetically modified organisms (GMOs) opens up potential benefits, such as enhanced food security and disease resistance. Yet, ethical dilemmas arise when considering unintended consequences. Could modifying genes create new allergens in food? Will we inadvertently disrupt local ecosystems? These questions necessitate a careful approach, balancing innovation with caution.
Another significant aspect is the regulatory frameworks across nations. Various countries adopt different stances on bio genetic engineering, reflecting their cultural values and ethical perspectives. For instance, the European Union maintains strict regulations concerning GMOs, focusing heavily on safety assessments and public consultation. In contrast, countries like the United States tend to embrace a more permissive approach, favoring innovation and economic growth. This diversity can lead to challenges, particularly in global commerce and trade. Striking a balance between promoting scientific advancements and ensuring public safety becomes a vital discussion point for policymakers worldwide.
Lastly, public perception and acceptance of bio genetic engineering often wields a substantial influence over policy and practice. Misinformation and a lack of understanding can foster resistance among the general populace. For example, campaigns against GMOs have gained traction due to fears about food safety and environmental impact.
Understanding public concerns is crucial for scientists and policymakers alike. Open dialogue and education can aid in demystifying bio genetic engineering, fostering a more informed public discourse.
Efforts to improve perception might include transparent communication about the benefits and risks associated with genetically modified organisms. Engaging communities in discussions is essential to build trust and acceptance. Approaching education about bio genetic engineering with sensitivity to public sentiment can help bridge the gap between scientific innovation and societal values.
In summary, ethical considerations in bio genetic engineering encompass a rich tapestry of moral implications, regulatory frameworks, and public perception. Each of these elements warrants careful examination as we navigate the complex landscape of genetic manipulation, ensuring that advancements genuinely serve humanity while preserving the integrity of natural ecosystems.
Impact on Biodiversity
The profound implications of bio genetic engineering on biodiversity cannot be overstated. As we fine-tune the genetic material of organisms, both intentionally and inadvertently, we shape the natural world in ways that can lead to significant shifts in ecological balances. Genetic modification doesn't just pave the way for agricultural efficiency; it alters ecosystems, affecting habitats, native species, and interspecies relationships. The ramifications of these biological interventions are far-reaching, and understanding them helps navigate the complexities of modern environmental stewardship.
Effects of Genetically Modified Organisms
The rise of genetically modified organisms (GMOs) has initiated a re-evaluation of biodiversity dynamics. On one hand, these organisms can lead to remarkable innovation in food production and disease resistance. For example, crops such as Bt corn possess the gene from Bacillus thuringiensis that grants them pest resistance, consequently reducing the need for chemical pesticides. This has positive implications for non-target species, promoting healthier ecosystems.
However, the introduction of GMOs also brings about an array of concerns regarding nature's intricate web. Some genetically modified plants might outcompete their wild relatives. This can result in a sort of biological imperialism, where a modified variant effectively sidelines native species, potentially leading to a loss of genetic diversity. An alarming scenario could arise if the genetic traits of these GMOs infiltrate wild populations, which might push certain species to extinction due to their inability to adapt.
"The complexity of nature means that any modification can have unintended consequences, echoing further through the ecosystem."
Potential Risks of Gene Flow
A pressing issue in the realm of bio genetic engineering is the potential for gene flow, a process where genes from genetically modified organisms move into wild populations or conventional crops. This phenomenon underscores an essential consideration: once these genes enter the wild, tracking them becomes an arduous task.
For instance, if transgenic traits are unwittingly transferred to wild relatives, it could spark unintended ecological consequences. A key concern is the hybridization between GMOs and wild relatives, creating strains that could outcompete native flora and fauna, thus diminishing biodiversity.
Several factors exacerbate this risk:
- Pollinator activity: Bees and other animals can carry pollen over considerable distances, facilitating gene transfer.
- Adaptability of modified traits: Some modified traits may provide competitive advantages, leading to a dominance of GMOs in natural or agricultural ecosystems.
- Seed dispersal: Human activities, such as agriculture, can inadvertently contribute to the spread of altered genes when seed stock mingles.
In summary, while the advancements in bio genetic engineering present remarkable opportunities, they also carry significant responsibilities. The push for innovation must coincide with safeguarding biodiversity. Thus, an ongoing dialogue among scientists, policy-makers, and the public is essential to ensure that bio genetic engineering enhances rather than undermines the intricate tapestry of life on Earth.
Future Directions in Bio Genetic Engineering
The realm of bio genetic engineering stands at a crossroads, poised to transform numerous sectors through advancements in its techniques and technologies. This topic is crucial for understanding how the future will shape society, agriculture, healthcare, and ecological management. By looking ahead, we can unravel the potential benefits and challenges that the evolution of this field may bring.
Advancements in Techniques and Technology
Advancements in techniques and technology within bio genetic engineering are expected to accelerate at a breakneck pace. One notable example is the ongoing refinement of the CRISPR-Cas9 system. Researchers are exploring not only its precision but also its capabilities to edit multiple genes simultaneously. This could lead to significant breakthroughs in treating complex diseases where multiple genetic factors are at play.
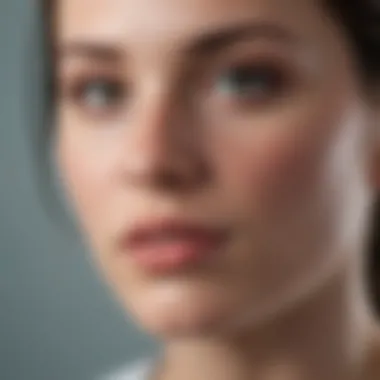
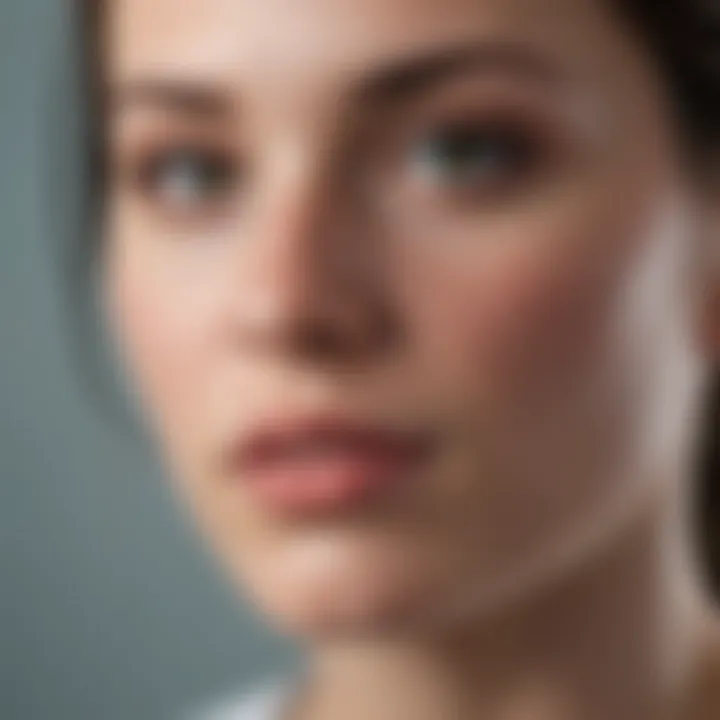
Furthermore, synthetic biology raises the bar by allowing scientists to design and construct entirely new biological parts. With tools like DNA synthesis becoming more cost-effective, the potential for bioengineering organisms to produce high-value chemicals and even biofuels independently has become increasingly likely.
"The rewriting of life's code opens doors we once thought were nailed shut."
Another exciting avenue is the development of gene drives, which can increase the chances of certain genes being passed to offspring. This technology presents both promising and troubling possibilities, such as eradicating disease vectors like mosquitoes but also raises ethical questions about its ecological impact.
Moreover, advancements in computational biology and AI are ushering in new paradigms for data analysis and predictive modeling. With these tools, researchers can better understand complex biological interactions and enhance their ability to forecast outcomes of genetic modifications. This confluence of technology signals that we are on the brink of a new epoch in biological research and discovery.
Predicting Societal Impact
As we gaze into the future, the societal implications of advancements in bio genetic engineering are paramount. The numerous possibilities this field presents can shape cultural norms, economic stability, and ethical conversations. Happiness and wealth could hinge on how effectively we navigate these innovations.
There are several societal aspects to consider:
- Health Equity: If gene-editing technologies become widely accessible, there may be a chance to bridge gaps in health inequalities. This could revolutionize treatments for conditions that have long affected marginalized communities.
- Food Security: With climate change and population growth, bio genetic engineering offers promising solutions for agricultural resilience. Genetically engineered crops that can withstand harsh conditions may become vital to food supply sustainability.
- Employment Shifts: The rise of automated biotechnology processes could alter the job landscape. While new job opportunities may arise in engineering and data analysis, there may also be a decline in traditional agricultural jobs.
- Ethical Dilemmas: The ethical implications of gene editing must not be overlooked. Societal acceptance of these technologies will likely evolve, but will it happen swiftly enough to prevent potentially harmful regulations?
In summary, the future of bio genetic engineering is fraught with challenges and opportunities. By embracing the advancements and preparing for the societal shifts, we can better navigate the uncharted territories that lie ahead.
Policy and Governance
Navigating the roadways of bio genetic engineering unfolds numerous complexities, and at the heart of these challenges lies the crucial aspect of policy and governance. The swift advancement in genetic manipulation requires policies that not only address current issues but are also adaptable to future developments. The pivotal role of policy ensures that the transformative potential of bio genetic engineering is harnessed responsibly. Understanding these regulations uplifts discourse, guiding researchers, practitioners, and policymakers through a maze of ethical, legal, and societal ramifications.
In essence, sound governance frameworks facilitate the safe development and application of genetic technologies. The importance of stringent and clear regulations cannot be overstated, especially when one considers the potential for unintended consequences. Benefits often associated with genetic advancements come with their own set of risks, necessitating a balance that safeguards both innovation and public interest.
Need for Comprehensive Regulations
The landscape of genetic engineering is rife with opportunities, yet equally fraught with uncertainties. As the science evolves, so too must the regulations that govern it. Without comprehensive regulations, the field risks falling into a chaotic free-for-all, where advancements outpace ethical considerations and public safety. A solid framework aids in ensuring that the developments correlate with societal values and ethical norms.
Policymakers must consider several key factors when formulating regulations:
- Public Health and Safety: Regulations must prioritize the health of individuals and the ecosystem.
- Ethical Standards: As technologies like CRISPR gain traction, it is imperative to instill guidelines that reflect ethical deliberations among stakeholders.
- Consumer Transparency: Educated consumers can make informed choices, an aspect reinforced through transparent processes and labelling of genetically modified products.
A collective approach to regulation can prevent misuse and promote innovation that aligns with community values both now and in the future.
International Collaboration Efforts
In a world increasingly interconnected, the dialogue surrounding bio genetic engineering must transcend borders. International collaboration is a linchpin that can foster a cohesive and comprehensive global regulatory approach. By sharing insights and best practices across nations, stakeholders can tackle common challenges and create standards that transcend individual legislative contexts.
Key aspects of international collaboration include:
- Shared Research Initiatives: Countries can engage in joint research projects that address global issues such as food security and environmental sustainability.
- Standardized Regulations: Collaborating states can work towards unified guidelines that ensure safety and ethical standards are met on a global scale.
- Cross-National Forums: Establishing platforms for dialogue facilitates knowledge sharing and allows diverse perspectives to be considered, giving a broader context to the implications of bio genetic engineering.
"Science knows no borders, and neither should our response to its challenges."
These collaborative efforts not only amplify the understanding of genetic technologies but also ensure that policy and governance are informed by a variety of viewpoints, ultimately leading to more holistic regulations that reflect global concerns.
In summary, effective policy and governance structures are instrumental in shaping the future of bio genetic engineering. By addressing the need for comprehensive regulations and fostering international collaborations, stakeholders can navigate the complexities of this field responsibly. As we usher in advancements in genetic technologies, the importance of thoughtful governance will become even more clear.
Closure
The conclusion holds a significant place in discussing bio genetic engineering. It serves as a capstone for the knowledge shared, allowing readers to distill the essential insights that were presented throughout the article. Here, we reflect on the core principles connected to bio genetic engineering, recognizing its impact on diverse fields such as agriculture, medicine, and environmental science.
This article has detailed not only the historic evolution of the subject but also the cutting-edge techniques that propel the field forward. Importantly, it has addressed the accompanying ethical considerations that provoke thought and debate. By synthesizing these elements, we can appreciate the complex interplay between innovation and morality, which is a recurring theme in bio genetic engineering.
Synthesis of Key Findings
In reviewing the article's core points, several critical findings emerge:
- Historical Context: The journey from past genetic manipulations to modern practices highlights how far the scientific community has come. Early discoveries laid fundamental groundwork, which has now blossomed into sophisticated methodologies.
- Modern Techniques: Technologies like CRISPR-Cas9 have revolutionized genetic editing, making it more precise and accessible. The expansion into synthetic biology showcases human ingenuity in reshaping life.
- Applications: The practical benefits, especially in agriculture through genetically modified organisms that promise increased yield and resistance to pests, underscore the potential advantages bio genetic engineering brings.
- Ethical Considerations: As we progress, the importance of establishing clear moral boundaries and regulatory frameworks cannot be overlooked. Society must grapple with the implications of altering life at a genetic level.
By understanding these findings, readers can grasp that bio genetic engineering offers both immense opportunities and significant challenges that require careful navigation.
Future of Bio Genetic Engineering
Looking ahead, bio genetic engineering is poised for remarkable transformations. Anticipating advancements involves recognizing key trends:
- Technological Innovation: Developments in gene editing, artificial intelligence, and bioinformatics will likely enhance the precision and scope of genetic modifications.
- Interdisciplinary Collaborations: The convergence of biology with fields such as computer science will cultivate new perspectives and solutions. Collaborative efforts among researchers, policymakers, and ethicists will be pivotal.
- Public Engagement: As the general public becomes more educated on these matters, discussions surrounding bio ethical considerations will intensify. Transparency in research and open dialogues with communities are essential.
"With great power comes great responsibility."
This notion resonates profoundly in the realm of bio genetic engineering, emphasizing the importance of responsible scientific practice.