Exploring Inkjet Bioprinting in Regenerative Medicine
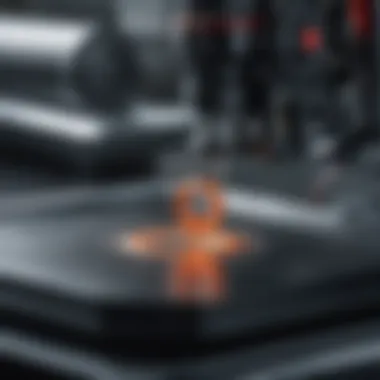
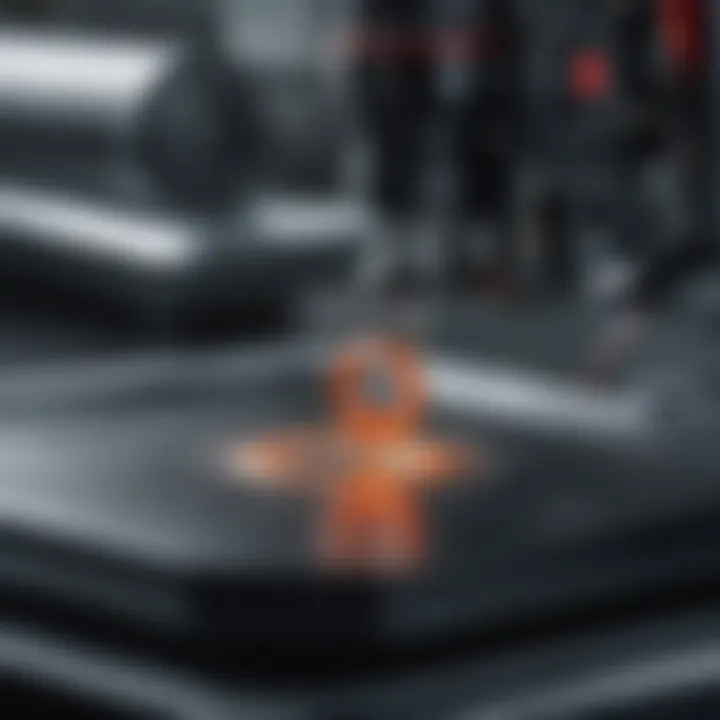
Intro
Inkjet bioprinting is emerging as a crucial technology in regenerative medicine and tissue engineering. Its ability to precisely deposit live cells and biomaterials makes it a promising approach for creating scaffolds that can mimic natural tissue. This technique provides a unique avenue to address various challenges in healthcare, such as organ shortages and disease treatment. The focus on this technology opens up discussions about its mechanisms, applications, and the hurdles that researchers face.
Research Highlights
Overview of Key Findings
Inkjet bioprinting operates primarily on the principles of drop-on-demand technology. This method allows for exact placement of materials, creating complex three-dimensional structures that can resemble human tissues. Research has demonstrated that bioprinting can integrate multiple cell types, creating environments that enhance tissue functionality. Materials used in inkjet bioprinting range from hydrogels to more advanced bioinks that support cell viability and proliferation. These findings establish the foundational knowledge necessary for further exploration of this technique.
Significance of the Research
Understanding inkjet bioprinting is vital as it potentially addresses numerous healthcare issues. The ability to produce customized tissues could significantly impact transplant medicine, reducing waiting lists and increasing the success rates of grafts. Moreover, advancements in bioprinting technologies offer insights into drug development and disease modeling, enabling researchers to create better therapeutic strategies. This research also has implications for personalized medicine, allowing for treatments tailored to individual patient needs.
Inkjet bioprinting transforms how tissues are engineered, providing solutions that previous methods could not achieve.
Original Research Articles
Summary of the Article
Research articles on inkjet bioprinting delve into various aspects of the technology. These include the procedural steps involved in the bioprinting process, the characterization of bioinks, and analysis of printed constructs. The literature frequently emphasizes the importance of maintaining cell activity during and after the printing phase to ensure successful tissue integration in vivo.
Author Contributions
Many prominent researchers contribute to this field. They explore diverse applications of inkjet bioprinting, ranging from skin grafts to organs-on-a-chip. Each article often highlights specific advancements in techniques or materials used, showcasing their impact on future healthcare applications. Their collaborative works create a comprehensive framework that continues to evolve as technology improves.
Inkjet bioprinting represents a convergence of engineering and biology, offering a glimpse into the future of medical therapies and applications. This exploration reveals how the technology shares a pivotal role in reshaping the narrative of regenerative medicine.
Prolusion to Inkjet Bioprinting
Inkjet bioprinting represents a pivotal convergence of technology and biology. It offers a unique methodology for fabricating complex three-dimensional biological structures by precisely depositing bio-materials. This section emphasizes the significance of inkjet bioprinting in the realm of tissue engineering and regenerative medicine. Understanding this technology's nuances is essential, as it bridges the gap between scientific theory and practical, clinical applications.
Definition and Overview
Inkjet bioprinting is a technique that utilizes traditional inkjet technology to deposit living cells and biomaterials onto a surface. Its process is characterized by high precision and the capacity for generating intricate biological constructs layer by layer. Bioinks, typically a mixture of cells, biological materials, and nutrients, play a critical role in this method.
This technology relies on two primary system types: drop-on-demand and continuous inkjet systems. Each employs distinct mechanisms to eject droplets, which permits careful control over cell distribution. Inkjet bioprinting enhances the ability to create complex tissue architectures that are essential for mimicking natural organ structures. Its advantages include reproducibility, adaptability, and a high degree of customization.
Historical Context
The roots of inkjet bioprinting can be traced back to the late 20th century. The evolution of inkjet printing technology in the 1980s laid the groundwork for its application in biological fields. Early experiments involved using inkjet printers modified to handle viscous materials, such as gels and cells.
Initially, bioprinting faced significant technical hurdles. Researchers struggled with issues surrounding cell viability, material compatibility, and the formation of stable structures. However, continuous research and improvements in bioink formulations led to enhanced outcomes over the years.
By the early 2000s, advancements in digital manufacturing began to influence the field positively. The first systematic studies on bioprinting appeared around this time, illustrating the potential of using living cells in combination with printed scaffolds. As this research progressed, various applications emerged, ranging from organ-on-a-chip systems to large-scale tissue reconstruction.
Today, inkjet bioprinting stands at the forefront of biotechnological innovation, paving the path toward more effective solutions in regenerative medicine and beyond.
Principles of Inkjet Bioprinting
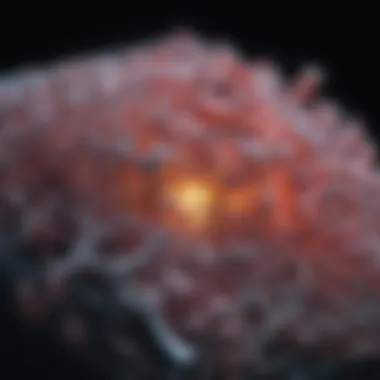
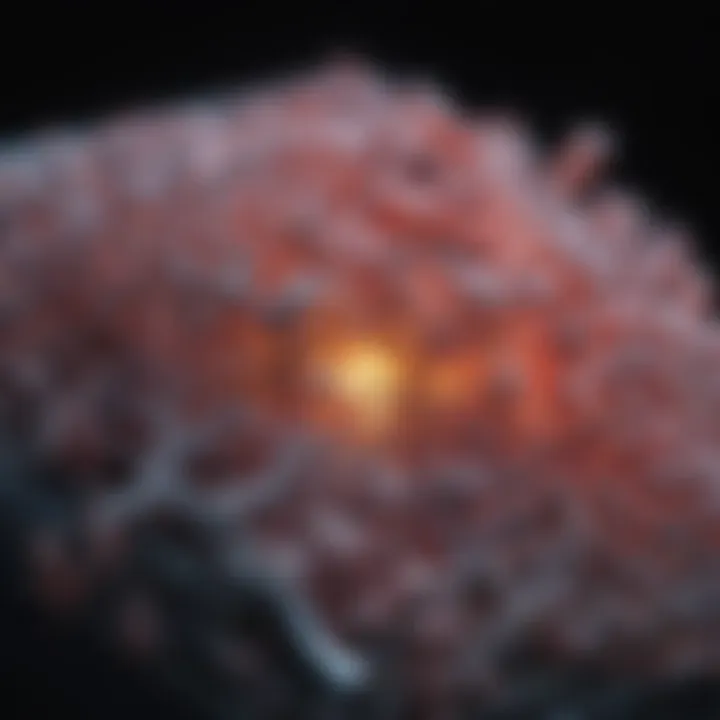
Inkjet bioprinting stands at the convergence of advanced technology and biomedical engineering. Understanding the principles behind this innovative process is vital for grasping its potential applications and impacts. Inkjet bioprinting utilizes drop-on-demand technology and material deposition techniques to create biological constructs with precision. As we delve into these principles, we will uncover how they contribute to enhancing tissue engineering and regenerative medicine.
Mechanisms of Inkjet Technology
The main mechanism behind inkjet technology involves controlled droplet formation and ejection from a nozzle. In this process, each droplet is precisely released at the required moment, allowing for exact placement of bioinks. This technology excels in its accuracy and speed, enabling the creation of intricate structures that mimic natural tissues.
The two primary modes of droplet ejection are thermal and piezoelectric. Thermal inkjet technology uses heat to vaporize a small portion of the ink, creating a bubble that forces a droplet out. In contrast, piezoelectric technology relies on electric charges to deform a crystal within the nozzle, enabling the controlled expulsion of bioink droplets.
Types of Inkjet Bioprinting Systems
Inkjet bioprinting systems can be categorized primarily into two types: Drop-On-Demand Systems and Continuous Inkjet Systems. Each type has its own unique advantages and applications, making them suitable for different bioprinting tasks.
Drop-On-Demand Systems
Drop-On-Demand Systems (DOD) are crucial in inkjet bioprinting due to their ability to release droplets only when needed. This characteristic minimizes wastage of bioink, making it a resource-efficient process. DOD systems allow for precise control over droplet size and placement, which is essential for creating complex biological structures.
One unique feature of Drop-On-Demand Systems is their flexibility. They can handle a wide variety of bioinks, including those that contain living cells or biomaterials, without compromising the cell viability. This ability ensures that printed structures retain functionality and can integrate with host tissues effectively.
The advantages of Drop-On-Demand Systems include high reproducibility and low running costs, although they might face challenges with the viscosity of certain bioinks.
Continuous Inkjet Systems
Continuous Inkjet Systems (CIJ) operate by continuously emitting droplets of bioink. Unlike DOD systems, CIJ maintains a steady flow of ink and uses electrostatic charges to direct the droplets to the substrate. This process allows for high-speed printing and is particularly useful for large-scale production.
The continuous nature of CIJ makes it an appealing choice for applications requiring significant throughput. One key characteristic is its ability to produce extremely uniform droplets, which is beneficial for applications where consistency is critical.
However, CIJ systems may have limitations when it comes to intricate designs. The continuous flow can lead to a higher likelihood of clogging and may create challenges in maintaining cell viability during the printing process.
The choice between Drop-On-Demand and Continuous Inkjet Systems ultimately hinges on the specific requirements of the project. Each system offers unique benefits, and understanding these can aid in selecting the most effective approach for the desired application.
"The versatility of inkjet bioprinting technology opens up numerous avenues in tissue engineering, and understanding its principles is essential for further advancements in the field."
Materials Used in Inkjet Bioprinting
The use of appropriate materials in inkjet bioprinting is a fundamental aspect of the technology's success and effectiveness. Good material selection can greatly enhance the performance and functionality of the bioprinted constructs. Bioinks and cell viability are two critical elements in this domain that must be properly understood, developed, and optimized.
Bioinks: Composition and Properties
Bioinks are specialized materials designed for bioprinting. They must be biocompatible and support cell attachment, growth, and differentiation. The composition of bioinks typically includes natural and synthetic polymers. Common choices are alginate, gelatin, and polyethylene glycol. These materials allow for a range of mechanical properties, enabling the printed structures to mimic the physical characteristics of the target tissue.
Key properties of bioinks include:
- Viscosity: Essential for ensuring consistent droplet formation during the printing process. Higher viscosity can lead to better shape retention post-printing.
- Shear-thinning behavior: This property ensures that the bioink flows easily during printing but regains its structure post-deposition.
- Degradability: Bioinks should ideally break down at a predictable rate to integrate with the surrounding tissue.
- Cell compatibility: It is vital that the materials in the bioink do not adversely affect cell health.
Cell Types and Viability
Cell viability is crucial for the success of any bioprinting application. Different types of cells can be used, depending on the intended application. Common choices include stem cells, fibroblasts, and endothelial cells. Each cell type presents unique advantages and challenges.
Challenges related to cell viability during and after the bioprinting process include:
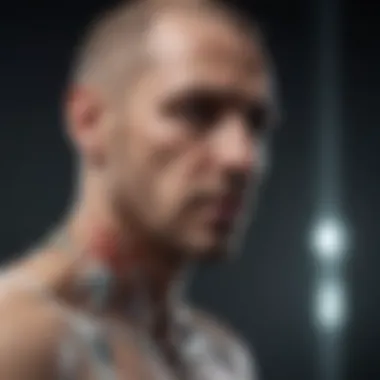
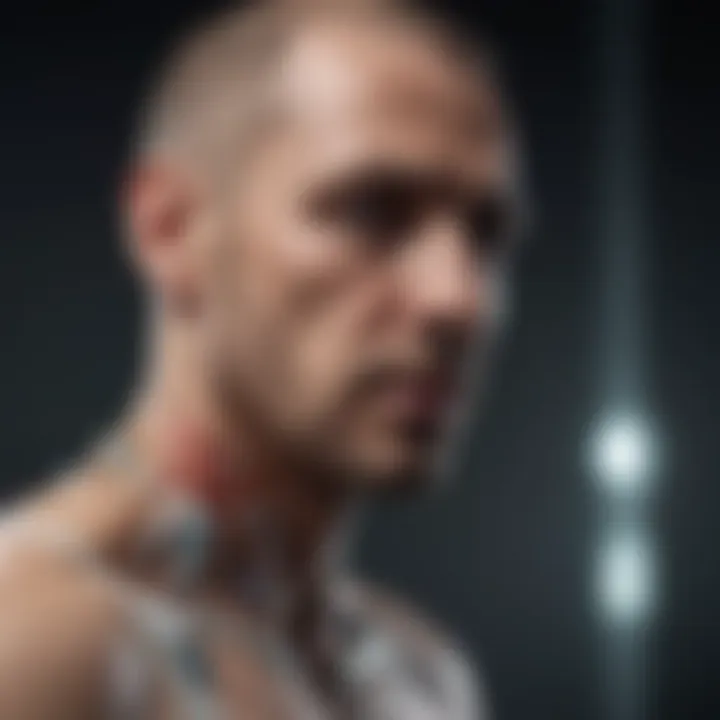
- Shear stress: This can affect cell health during the printing process itself.
- Nutrient diffusion: As bioprinted constructs increase in size, nutrients must adequately reach all cells. Failure to achieve this can lead to cell death in the core of larger constructs.
- Matrix support: The right bioink must be selected to support the specific cell types involved, which can vary widely.
"The choice of materials in inkjet bioprinting is not merely technical; it fundamentally changes how tissues can be engineered and how they might one day function in therapeutic settings."
Applications of Inkjet Bioprinting
Inkjet bioprinting is not just a technological marvel; it is rapidly transforming various fields, particularly in medicine and pharmaceuticals. The applications demonstrate how this technology can solve real-world problems by creating complex biological structures. This section delves into three primary areas: tissue engineering, pharmaceuticals, and cosmetic applications. Each of these domains showcases the versatility and potential impact of inkjet bioprinting on modern science and healthcare practices.
Tissue Engineering
Organ Models
Organ models produced through inkjet bioprinting represent a significant advancement in the field. These models offer highly realistic and functional replicas of human organs. The primary characteristic is their ability to closely mimic the architecture and functions of actual tissues. This high fidelity is critical in research and education as it allows scientists to study diseases and test drugs in more realistic environments compared to traditional models.
One crucial aspect of organ models is their ability to support cell viability, which means that cells can live and function as intended in the bioprinted structures. This leads to better outcomes in experiments and can advance personalized medicine approaches. However, one downside is that creating fully vascularized organ models remains a challenge, limiting their immediate clinical applications.
Scaffolds for Regenerative Medicine
Scaffolds are another vital application of inkjet bioprinting in regenerative medicine. They serve as frameworks that promote cell attachment and growth. The key characteristic of scaffolds is their biocompatibility. A well-designed scaffold must interact well with the body without causing adverse effects. This makes them crucial in facilitating tissue repair and regeneration.
A unique feature of these scaffolds is their customizable structure. Researchers can tweak the porosity and surface topography to enhance cell migration and growth, optimizing tissue engineer processes. However, a noteworthy disadvantage is that safety and testing phases can be lengthy, potentially delaying clinical implementation of new scaffold designs.
Pharmaceuticals and Drug Testing
The application of inkjet bioprinting in pharmaceuticals is expanding. It provides a unique opportunity for high-throughput drug testing. Using bioprinted tissue models, researchers can assess drug efficacy and safety in a controlled environment. This can significantly reduce the time taken to bring new drugs to market.
Incorporating patient-specific models allows for personalized drug testing. This means treatments can be tailored based on an individual's unique biological makeup. The emerging trend is focusing on 3D-printed microfluidic devices that facilitate simultaneous testing of multiple drug candidates. Nonetheless, the need for rigorous standardization and regulatory approval is a challenge in integrating these technologies into everyday practice.
Cosmetic and Dermatological Applications
Inkjet bioprinting is making strides in the cosmetic industry as well. It is used to create skin models for testing new products. These models can simulate human skin more accurately than traditional testing methods, allowing for a deeper understanding of skin reactions to new formulations.
The advantage of bioprinted skin models is their ability to exhibit normal cellular behavior, providing a realistic environment for testing. Companies benefit from faster and more reliable results, reducing the reliance on animal testing. However, high costs and the necessity for regulatory compliance can restrict widespread adoption.
Inkjet bioprinting, through its diverse applications, is ushering in a new era in several fields. From organ models and scaffolds in medicine to drug testing and creating skincare products, the potential is vast. Each application brings its unique benefits and challenges, contributing to the ongoing evolution of this promising technology.
Challenges in Inkjet Bioprinting
The field of inkjet bioprinting represents a merging of engineering and biological science, yet it is far from flawless. Understanding the challenges inherent in this technology is essential for anyone involved in tissue engineering and regenerative medicine. This section highlights the technical limitations, regulatory hurdles, and ethical considerations that currently hinder the widespread adoption of inkjet bioprinting, offering important insights for students, researchers, and professionals working in the industry.
Technical Limitations
Inkjet bioprinting is celebrated for its precision and ability to create intricate biological structures. However, it faces significant technical hurdles. One primary concern is the resolution of the printed structures. Fine details often become blurred at the micro level, which can compromise the functionality of the printed tissues. Moreover, droplet size variability during printing can also pose challenges in achieving consistent results.
Another crucial element is cell viability post-printing. Cells can become stressed or damaged during the printing process, negatively affecting their growth and functionality. The temperature and shear stress during the process require careful management to ensure that cells remain alive and functional.
Lastly, the compatibility of bioinks with living tissues is still under exploration. Each bioink must exhibit appropriate viscosity and mechanical properties. Any mismatch can lead to a failure in the intended application, thus highlighting the need for more advanced formulations and better understanding of biomaterials.
Regulatory Hurdles
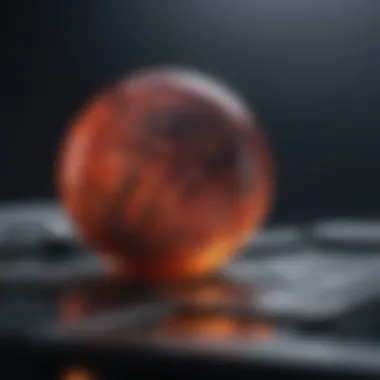
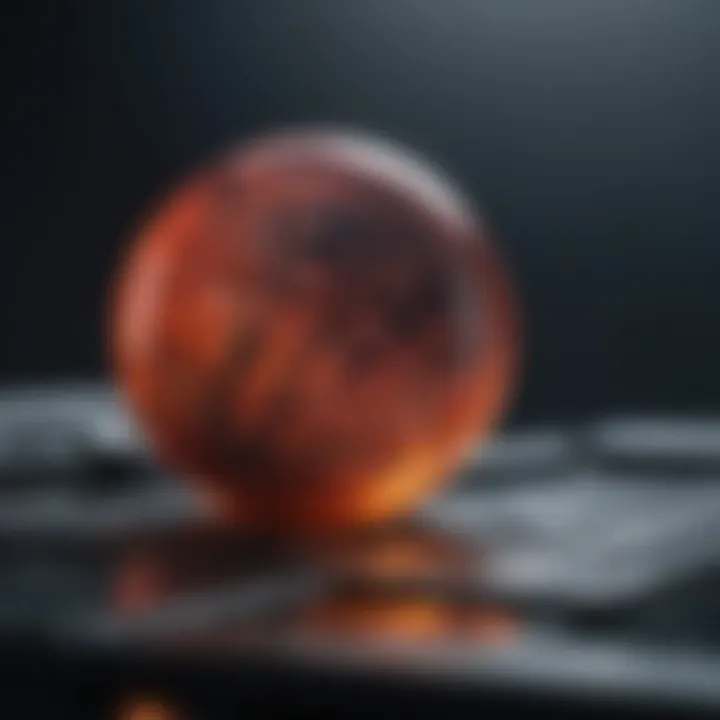
Navigating the regulatory landscape presents its own set of challenges. As inkjet bioprinting touches upon areas such as genetic modification and cell therapy, establishing reliable guidelines becomes a complex task.
Current regulations may not fully accommodate the rapid advancements in bioprinting technology. For instance, entities such as the FDA in the United States have restrictive policies regarding the approval processes for new bioprinting products. The classification of bioprinted materials can be ambiguous; thus, obtaining clearance can be prolonged.
As the field evolves, regulatory agencies must update existing frameworks to provide clear pathways for product approval. This is vital to foster innovation while ensuring patient safety and efficacy.
Ethical Considerations
Ethics is an integral part of the discourse surrounding inkjet bioprinting. The creation of living tissues raises profound questions about the manipulation of biological materials. Concerns over bioprinting human tissues touch upon moral boundaries and the potential consequences of individual ownership of biological materials.
Furthermore, the risk of bioweaponization, or the misuse of bioprinting technology for harmful purposes, looms large in the background. This reality amplifies the need for stringent ethical guidelines to govern research and application.
Lastly, there is the issue of accessibility. As advancements are made, ensuring equitable access to bioprinting technology across different populations poses significant challenges. Balancing innovation with the responsibility to serve all sectors of society is a pressing concern that must be addressed thoughtfully.
"Understanding the challenges we face in inkjet bioprinting is crucial for advancing this exciting technology."
As inkjet bioprinting continues to develop, overcoming these challenges will enhance its potential in medical applications. This area remains ripe for research and innovation, with a clear necessity for collaborative efforts among scientists, ethicists, and policymakers.
Future Directions and Trends
The exploration of future directions and trends in inkjet bioprinting reveals significant advancements and potential pathways. These developments may redefine its applications in tissue engineering and regenerative medicine. Understanding these trends is crucial for researchers, medical professionals, and institutions aiming to adopt or invest in bioprinting technologies. The effects of such innovations can enhance functionality, improve outcomes, and expand applications, which adds value to the healthcare system.
Innovations in Bioink Development
Innovations in bioink development are pivotal for the progress of inkjet bioprinting. These bioinks must exhibit biocompatibility, adequate mechanical properties, and the ability to support cellular functions. New formulations are emerging that combine synthetic and natural materials to optimize printing performance and cellular interaction. For example, alginate and gelatin blends are becoming popular due to their favorable properties. These innovations lead to improved cell survival rates during and after printing, enhancing the viability of the printed constructs.
Integration with Other Technologies
Integrating inkjet bioprinting with other technologies can enhance its capabilities. Such integrations include:
Nanotechnology
Nanotechnology is particularly relevant in improving bioink characteristics. It involves manipulating materials at the nanoscale to create unique properties. The incorporation of nanoparticles can enhance mechanical strength and electrical conductivity. Additionally, nanotechnology can facilitate better cellular interactions through surface modifications. This characteristic makes nanofeatures beneficial for targeted applications in tissue engineering. However, potential concerns include toxicity and the need for careful regulatory considerations.
Biotechnology Advances
Advances in biotechnology have a profound impact on inkjet bioprinting practices. These developments include genetic engineering methods to produce cells tailored for specific purposes. This capability allows for the creation of more specialized tissues. Biotechnology also allows for improving the availability of stem cells and other critical cell types. The robustness of bioproduction systems can be a unique feature, providing efficiency and reproducibility in mass production. Still, the complexity of these systems may require extensive research and validation before widespread adoption occurs.
Perspective on Clinical Applications
The future of clinical applications for inkjet bioprinting is promising. As technology improves, the capability to produce complex tissues accurately can lead to more effective treatment options. Regenerative medicine may achieve revolutionary advancements through precise cellular placement and functional tissue creation. Furthermore, personalized medicine can progress by bioprinting patient-specific grafts, leading to higher success rates in surgical interventions and transplantations.
"The exciting future of inkjet bioprinting lies not just in what we can print but how we can print it to transform patient care and outcomes."
With these advancements, professionals can expect more effective collaborations between disciplines, including materials science, medicine, and engineering. The drive towards more integrated approaches signifies a shift in the landscape of medical technology and its applications.
Closure
In the intricate field of inkjet bioprinting, understanding the conclusion is pivotal for appreciating the transformative potential of this technology in healthcare. This article consistently emphasizes that inkjet bioprinting stands at the confluence of innovation and necessity, particularly within tissue engineering and regenerative medicine. The ability to create cellular structures with precision opens new pathways for medical advancements, accelerating the development of personalized medicine and complex organ models.
Summary of Key Points
- Definition and Overview: Inkjet bioprinting is a revolutionary technology combining aspects of biology and printing. It offers diverse possibilities for producing tissues and organs on demand.
- Principles of Bioprinting: The mechanisms of inkjet technology, including drop-on-demand and continuous systems, provide a foundation for effective bioprinting processes.
- Materials Utilized: Bioinks contain living cells and biomaterials essential for creating viable tissue constructs. The choice of materials impacts the outcome significantly.
- Application Spectrum: Applications span tissue engineering, pharmaceuticals, and even cosmetic uses, showcasing the wide-ranging implications of bioprinting.
- Challenges: Issues such as technical limitations, regulatory hurdles, and ethical considerations pose challenges that the field must address to evolve further.
- Future Directions: Innovations in bioink development and integration with technologies like nanotechnology herald an exciting future for inkjet bioprinting in clinical applications.
Final Thoughts on the Future of Inkjet Bioprinting
The future of inkjet bioprinting remains optimistic. As researchers continue to address current challenges, the integration of new technologies will likely enhance the capabilities of bioprinting. With ongoing advancements in materials and techniques, there is a potential to transition theoretical models into concrete solutions for real-world medical challenges. The application of inkjet bioprinting could revolutionize organ transplant procedures and personalized drug tests.