An In-Depth Exploration of Quantum Physics
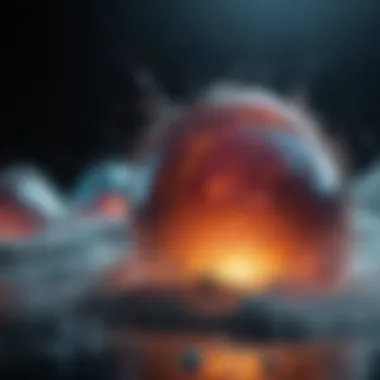
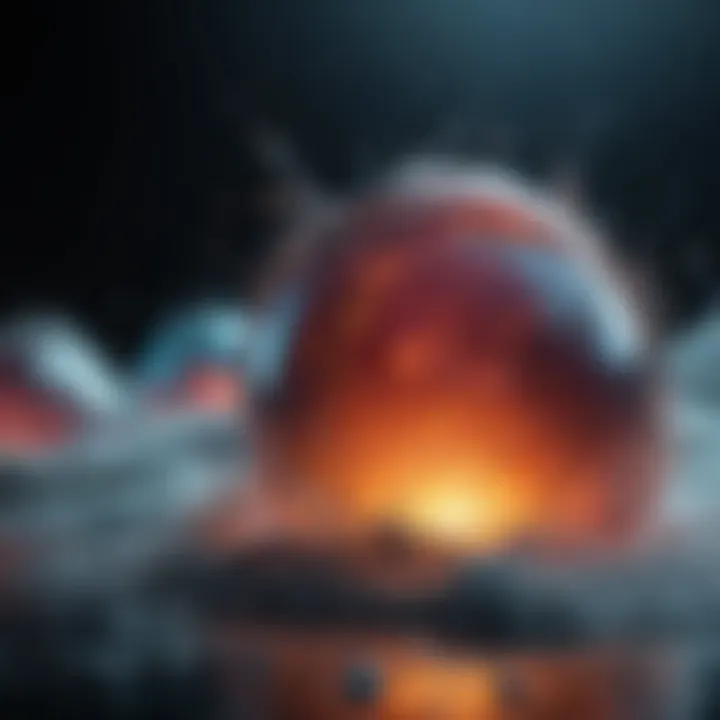
Intro
Quantum physics, a field that fascinates and perplexes, underpins much of modern scientific understanding. As we delve deeper into this realm, it is essential to grasp not only the definitions of fundamental concepts but also the implications these concepts hold for our everyday lives and the universe at large.
Historical context plays a pivotal role in shaping the theories and frameworks that we explore today. From the early 20th century until now, quantum physics has evolved significantly, introduced by iconic figures such as Max Planck, Albert Einstein, and Niels Bohr. Their contributions laid the groundwork for an intricate framework that would transform our understanding of particles and waves.
This article aims to provide clarity amidst the complexity of quantum physics. Each section connects to form a detailed narrative that elucidates key phenomena, like wave-particle duality and quantum entanglement. Additionally, we will discuss debates and ongoing challenges in this exciting field, shedding light on the nature of reality as perceived through the lens of quantum theory.
Prelims to Quantum Physics
Quantum physics forms a cornerstone of modern science, influencing various fields including chemistry, materials science, and information technology. Its principles not only reveal the behavior of particles at microscopic levels but also challenge our perceptions of reality. Understanding quantum phenomena is critical for anyone aiming to grasp the intricacies of fundamental science and its applications.
Definition and Scope
Quantum physics, or quantum mechanics, refers to the branch of physics that deals with the mathematical description of the dual particle-like and wave-like behavior of matter and energy. It also encompasses various principles that govern systems at very small scales, such as atoms and subatomic particles. The scope of quantum physics extends beyond mere academic curiosity; it has practical implications in technologies such as semiconductors, lasers, and quantum computing.
Some key aspects include:
- Wave-Particle Duality: Understanding that particles behave as both waves and particles, a fundamental concept in quantum physics.
- Uncertainty Principle: A principle introduced by Werner Heisenberg that asserts the limitations of simultaneously knowing certain pairs of physical properties, such as position and momentum.
- Quantum States: The idea that physical systems exist in states which can influence their behavior when observed.
Historical Development
The journey to quantum physics began in the early 20th century, as scientists sought to explain phenomena that classical physics could not adequately address. Notable milestones include:
- Max Planck’s Hypothesis: In 1900, Planck introduced the concept of quantized energy levels to explain black-body radiation, setting the foundation for quantum theory.
- Albert Einstein’s Photons: In 1905, Einstein proposed that light can be thought of as both a wave and a particle, coining the term photon. This work explained the photoelectric effect and contributed significantly to the wave-particle duality principle.
- Niels Bohr’s Model: In 1913, Bohr developed a model of the hydrogen atom that integrated quantum theory with classical concepts, marking a critical departure from previous atomic models.
These developments laid a framework for further research, leading to advancements in quantum theory throughout the 20th century. Notable contributions by figures such as Erwin Schrödinger and Werner Heisenberg further refined our understanding, introducing tools like the Schrödinger equation and formalizing quantum mechanics.
"The quantum theory has revolutionized our understanding of atomic and subatomic phenomena, challenging traditional views and leading to technological innovations that reshape our world."
Thus, the historical context of quantum physics highlights not only a significant evolution of scientific thought but also its continuing relevance in technology and theoretical research today.
Fundamental Principles
The fundamental principles of quantum physics form the backbone of this field and demonstrate why it stands apart from classical physics. These principles reshaped our understanding of the natural world, introducing concepts that challenge our intuition. They are essential not only for theoretical physics but also for practical applications ranging from computing to cryptography. Engaging with these principles allows researchers and students to grasp how matter behaves at the smallest scales.
Wave-Particle Duality
Wave-particle duality is one of the most intriguing aspects of quantum physics. This principle suggests that particles such as electrons exhibit both wave-like and particle-like properties depending on the conditions of observation. This duality was illustrated famously in the double-slit experiment, where particles create an interference pattern typical of waves when not observed. However, when an attempt is made to measure which slit a particle passes through, the interference pattern collapses, and the particles behave as classical particles. This phenomenon reveals that the act of measurement influences the behavior of quantum systems.
Understanding wave-particle duality is critical because it challenges classical definitions of particles and waves. Researchers must consider both aspects to comprehend phenomena like electron behavior in atoms or how light interacts with matter. It also prompts discussions on the nature of reality, observation, and the potential limitations of human knowledge.
Uncertainty Principle
The uncertainty principle, formulated by Werner Heisenberg, posits fundamental limits on how precisely pairs of physical properties, like position and momentum, can be known simultaneously. Specifically, it states that the more accurately one property is measured, the less accurately the other can be determined. This principle signifies a departure from classical physics, where properties can, in theory, be measured without limitations.
This principle has profound implications for the philosophy of science. It suggests that at a quantum level, uncertainty is not a limitation of measurement but an inherent quality of nature itself. This influences how we conceptualize reality, encouraging a deterministic view to evolve into one that accommodates probability and uncertainty.
Understanding the uncertainty principle is vital for anyone engaged in the fields of quantum mechanics or quantum technologies. Researchers must navigate this principle when designing experiments, interpreting results, and developing new quantum technologies.
Quantum Superposition
Quantum superposition is another cornerstone of quantum physics, stating that a quantum system can exist in multiple states or configurations simultaneously until it is measured. This idea is often illustrated using Schrödinger's cat thought experiment, where a cat in a box can be considered both alive and dead until observed. This concept underpins much of quantum theory and leads to phenomena like quantum entanglement.
Superposition has significant implications in many applications. For instance, in quantum computing, qubits utilize superposition to process information in ways classical bits cannot. This enhances computational capability exponentially.
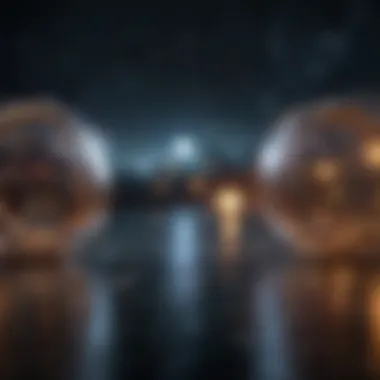
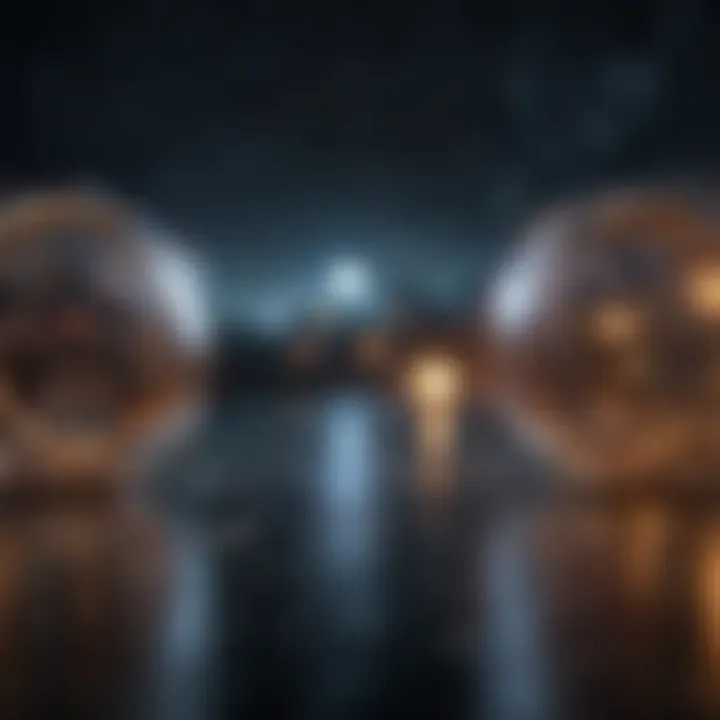
As researchers explore algorithms for quantum computing, they must harness superposition to leverage the potential advantages over classical systems. Understanding superposition is therefore essential for anyone working at the frontier of quantum technologies and fundamental physics.
Key Insights on Fundamental Principles
The fundamental principles of quantum physics are not merely theoretical but serve as practical guiding frameworks that inform cutting-edge research and technology. They reveal the non-intuitive nature of the quantum realm, advancing our quest for deeper understanding and innovative applications.
Key Theories in Quantum Physics
The field of quantum physics has evolved through several key theories that establish its foundations and guide contemporary research. Understanding these theories is vital for grasping the depth of quantum phenomena and their implications. Three theories stand out in this realm: Quantum Mechanics, Quantum Field Theory, and the Many-Worlds Interpretation. Each theory addresses different aspects of reality and expands our understanding of the universe at a fundamental level.
Quantum Mechanics
Quantum Mechanics is a cornerstone of modern physics, dealing with the behavior of matter and energy at very small scales. This theory first emerged in the early 20th century, primarily through the work of pioneers like Max Planck and Niels Bohr. It introduced concepts such as quantization of energy, wave functions, and the probabilistic nature of particle behavior.
Importance of Quantum Mechanics:
- Foundation of Quantum Theory: It serves as the foundational framework from which other theories, like Quantum Field Theory, have developed.
- Technological Impact: Quantum Mechanics has led to advancements in technology, including semiconductors and lasers, which play critical roles in everyday devices.
- Understanding Duality: The theory explains wave-particle duality, shedding light on the strange interplay between particles and waves.
In summary, Quantum Mechanics provides the essential tools for discussing atomic and subatomic phenomena, making it a fundamental theory in quantum physics.
Quantum Field Theory
Quantum Field Theory (QFT) takes Quantum Mechanics into a broader framework, merging it with special relativity. This theory views particles as excitations in underlying fields, rather than as isolated entities. QFT has been pivotal in formulating the Standard Model of particle physics, which describes the electromagnetic, weak, and strong interactions.
Significance of Quantum Field Theory:
- Integration of Forces: QFT successfully unifies various forces, exemplifying how basic particles and interactions can be explained through fields.
- Particle Production: It accounts for phenomena like particle-antiparticle creation and annihilation, which Quantum Mechanics struggles to describe fully.
- Predictive Power: QFT allows scientists to make precise predictions regarding particle behaviors, underscoring its importance in high-energy physics.
Through its principles, Quantum Field Theory enhances our understanding of the universe at its most fundamental level.
Many-Worlds Interpretation
The Many-Worlds Interpretation (MWI) emerges as a significant philosophical perspective in quantum physics. Proposed by Hugh Everett III in the 1950s, it posits that all possible outcomes of quantum measurements are realized in separate, parallel universes. This interpretation challenges traditional views of reality and introduces intriguing implications for the nature of existence.
Aspects of the Many-Worlds Interpretation:
- No Collapse of Wave Function: Unlike in the Copenhagen interpretation, MWI suggests that the wave function never collapses, leading to the coexistence of multiple realities.
- Determinism: This interpretation implies a deterministic universe at the quantum level, where every event spawns numerous realities based on different outcomes.
- Philosophical Questions: MWI raises profound questions about identity and free will, encouraging debate among physicists and philosophers alike.
The Many-Worlds Interpretation provides a unique viewpoint, prompting deeper examination of reality itself.
"Quantum physics doesn't just challenge our understanding; it reshapes it entirely, delivering insights that extend well beyond the realm of science."
Important Phenomena
The field of quantum physics includes phenomena that challenge classical physics' principles. These important phenomena provide insight into the behavior of the quantum world and play a significant role in shaping our current understanding of nature. The strange behaviors observed in quantum systems give rise to multiple applications in technology and theoretical frameworks that push the boundaries of scientific inquiry.
Quantum Entanglement
Quantum entanglement is one of the most intriguing aspects of quantum mechanics. When two particles become entangled, the state of one particle is directly connected to the state of the other, regardless of the distance separating them. This correlation defies classical intuitive notions about separate systems behaving independently.
Entanglement has profound implications for quantum computing and quantum cryptography. In computing, entangled states can enhance processing capabilities exponentially, allowing for operations that classical computers cannot perform efficiently. In cryptography, entangled states lay the foundation for secure communication methods, providing an unbreakable encryption method due to the inherent properties of quantum systems.
"Quantum entanglement is perhaps the most mysterious aspect of quantum mechanics, blurring the lines of locality and independence."
Quantum Tunneling
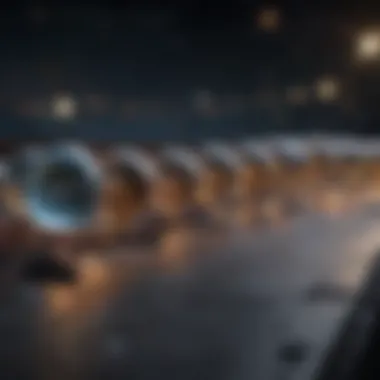
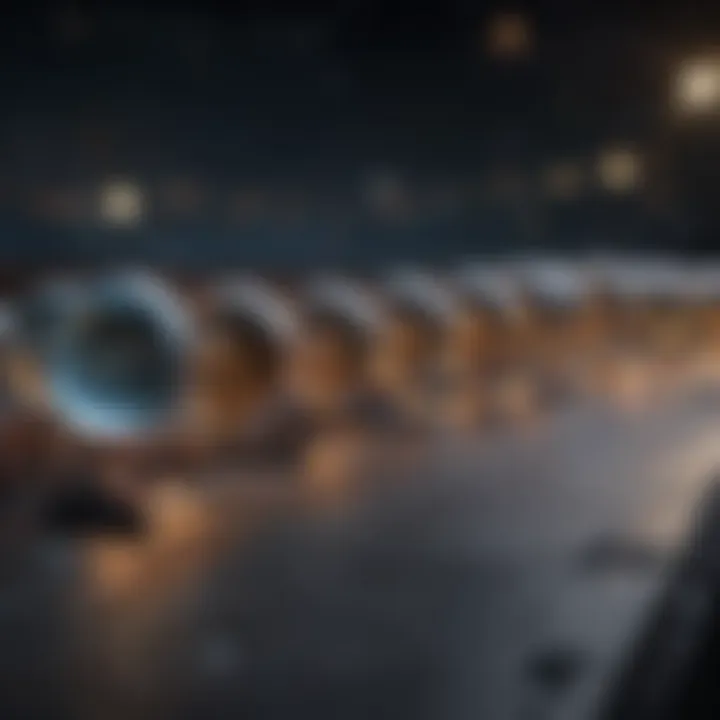
Quantum tunneling refers to the phenomenon where particles pass through potential barriers that they seemingly lack the energy to overcome. This behavior is a direct result of the wave-like nature of particles in quantum physics. In classical mechanics, a particle must have enough energy to surmount a barrier; in contrast, quantum mechanics reveals that particles can ‘tunnel’ through barriers under certain conditions.
This phenomenon is not just theoretical. Quantum tunneling is essential in nuclear fusion, allowing particles to overcome repulsive forces between them and participate in the reactions that power stars. It also has applications in semiconductor technology, where tunneling effects play a critical role in the functionality of devices like tunnel diodes.
Quantum Decoherence
Quantum decoherence describes the process by which quantum systems lose their quantum behavior and transition into classical states. When a quantum system interacts with its environment, it can no longer maintain coherent superpositions of states. This phenomenon explains why we do not observe macroscopic quantum phenomena in everyday life.
Debates on the implications of decoherence center on the measurement problem in quantum mechanics. Understanding decoherence is crucial for developing quantum technologies, as controlling or manipulating this process can enhance the stability of quantum systems. It is a critical aspect in quantum computing, where maintaining coherence is necessary for efficient information processing.
In summary, studying these important phenomena is key for advancing our grasp of quantum physics. Each phenomenon offers unique insights and practical applications, influencing both technological advancements and theoretical discussions in the field.
Applications of Quantum Physics
The applications of quantum physics are profound and varied. They extend beyond theoretical confines, entering real-world utilizations that impact everyday technology and sophisticated scientific fields. This section highlights several key areas where quantum principles are being applied, emphasizing their significance and implications.
Quantum Computing
Quantum computing represents a paradigm shift in computational capabilities. Unlike classical computers, which process information in binary (0s and 1s), quantum computers leverage the principles of superposition and entanglement. This allows them to perform many calculations simultaneously.
The core of quantum computing lies in qubits, analogous to classical bits but with the capacity to exist in multiple states at once. Consequently, quantum algorithms can solve complex problems much faster than traditional algorithms. Key potential applications include:
- Cryptography: Quantum computers can break traditional encryption, demanding new, quantum-safe methods.
- Material Science: They enable simulations of molecular behavior, leading to advances in drug discovery.
- Artificial Intelligence: Quantum computing holds promise for processing massive datasets more efficiently than classical systems.
Despite the potential, several challenges persist, including error rates in qubit operations and the need for stable environments to maintain quantum states. Researchers continue to explore ways to address these issues, aiming at practical, scalable quantum computers in the future.
Quantum Cryptography
Quantum cryptography leverages the principles of quantum mechanics to create secure communication channels that are theoretically invulnerable to eavesdropping. The key technology underpinning quantum cryptography is Quantum Key Distribution (QKD). In QKD, any attempt at intercepting communication alters the quantum state of particles involved, giving away the presence of an eavesdropper.
Some key benefits include:
- Enhanced Security: The security model of QKD does not rely on computational assumptions but rather on the laws of quantum physics.
- Key Generation: QKD allows two parties to generate a shared random secret key, which can be used for future encrypted communication.
- Scalability: Although currently limited, ongoing research aims at making quantum cryptography more practical for widespread use.
However, technological and logistical hurdles remain, such as the need for specialized hardware and the current limitation of transmission distances in QKD setups. Addressing these challenges is crucial to realizing the full potential of quantum cryptography in securing sensitive information.
Quantum Sensors
Quantum sensors leverage quantum states to achieve unprecedented precision in measurement tasks. Unlike conventional sensors, quantum sensors exploit phenomena such as superposition and entanglement, allowing for enhanced sensitivity and accuracy.
Applications of quantum sensors are broad and impactful:
- Gravitational Wave Detection: Instruments like LIGO utilize quantum technology to detect minuscule shifts in spacetime, enabled by the precision afforded by quantum mechanics.
- Medical Imaging: Quantum sensors could revolutionize MRI technology, offering higher resolution and faster imaging capabilities.
- Magnetic Field Sensing: Quantum sensors can measure magnetic fields with astonishing sensitivity, applicable in geological surveys and Mars exploration.
These applications illustrate the increasing integration of quantum technologies across various domains. Nevertheless, ongoing research is required to overcome practical issues, such as miniaturization and cost reduction, to enhance their adoption.
"Quantum technologies promise new frontiers in precision and security, reshaping how we interact with the world and each other."
Challenges and Controversies
Quantum physics is a field that not only reshapes our understanding of the microscopic world but also sparks ongoing discussions and controversies among physicists, philosophers, and even ethicists. These challenges can influence the direction of future research and technological innovations. Understanding the obstacles and debates within this discipline adds depth to our comprehension of quantum physics. These issues highlight the unresolved questions and the boundaries of knowledge, which are crucial for students, researchers, educators, and professionals.
Interpretational Challenges
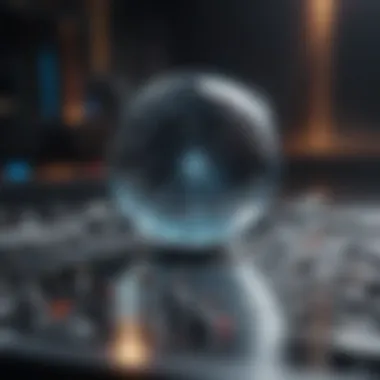
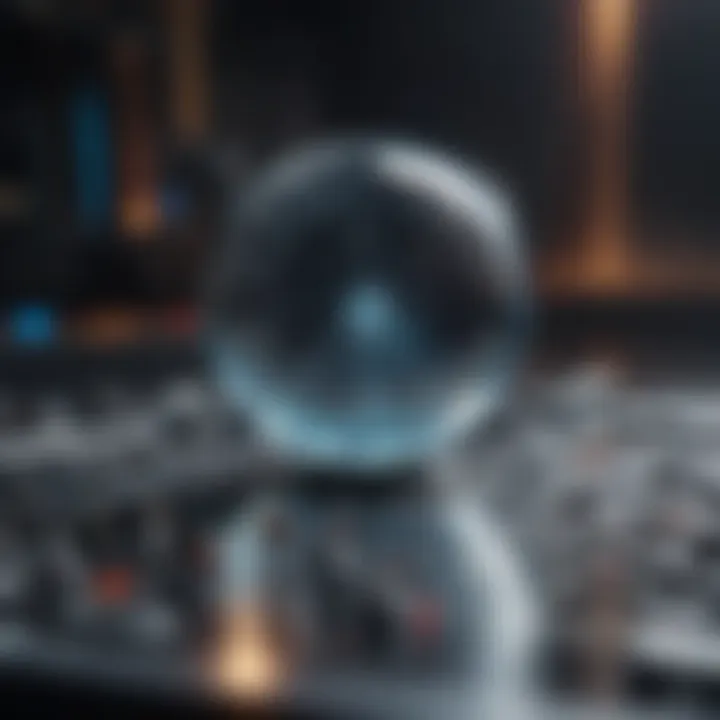
One of the most significant challenges in quantum physics lies in its interpretation. The mathematical formulations of quantum mechanics lead to different philosophical implications. Various interpretations try to explain the behavior of particles and waves, yet no single interpretation has gained universal acceptance.
Some of the prominent interpretations include:
- Copenhagen Interpretation: This approach posits that quantum particles do not have definite states until they are observed. It raises the question of the role of the observer in quantum mechanics.
- Many-Worlds Interpretation: In this view, all possible outcomes of quantum measurements are realized in separate, branching universes. This interpretation complicates the notion of reality.
- Pilot Wave Theory: This proposes that particles are guided by deterministic waves, suggesting a hidden variable theory that conflicts with the standard Copenhagen view.
Each interpretation carries its own philosophical implications and raises questions about determinism, reality, and the nature of existence. "The lack of consensus among scientists regarding quantum interpretations reflects deeper issues about reality and our understanding of it." Philosophers and physicists alike grapple with these interpretations, making them a crucial discourse in the study of quantum physics.
Experimental Limitations
Despite the theoretical advancements, experimental verification remains a significant hurdle in quantum physics. Some of the most notable limitations include:
- Precision of Measurements: Quantum phenomena are incredibly delicate. For example, in experiments on quantum entanglement, measuring equipment must maintain extreme precision to avoid disrupting the states being measured.
- Scalability Issues: Many quantum systems are studied under ideal conditions. Translating these results to larger, real-world systems is difficult. For instance, realizing a scalable quantum computer faces numerous technical challenges, such as error rates and qubit coherence.
- Replicability: Some quantum experiments yield results that are hard to replicate under different conditions. This lack of replicability raises questions about the validity of certain experiments, stifling the consensus on certain quantum theories.
These experimental limitations not only pose challenges for validating theoretical concepts but also influence how research is directed and funded in quantum physics. Such challenges push researchers to develop new methodologies and better technologies to address these issues.
By recognizing and engaging with these interpretational challenges and experimental limitations, we open doors to new avenues of inquiry. It is in grappling with these complexities that we continue to deepen our understanding of quantum physics and its implications.
Future Directions in Quantum Physics
Exploring future directions in quantum physics is crucial for understanding how this field may impact various domains of science and technology. As fundamental uncertainties fade, researchers push boundaries further, unveiling new horizons. The potential benefits are vast, ranging from revolutionary computing methods to breakthroughs in health care.
Emerging Research Areas
The study of quantum physics is fertile ground for several emerging research areas. Some notable topics include:
- Quantum Biology: This interdisciplinary field examines the role of quantum phenomena in biological processes. Researchers investigate how quantum coherence influences photosynthesis and enzyme reactions, fostering insights that may lead to novel medical treatments.
- Quantum Networks: Scientists are developing sophisticated quantum communication networks. These systems will utilize entanglement for secure data transmission. The ultimate aim is to create a global quantum network that supports secure communications.
- Quantum Machine Learning: This intersection between quantum computing and machine learning promises enhanced processing capabilities. Researchers explore algorithms that leverage quantum mechanics to solve complex problems more efficiently than classical algorithms.
As these areas evolve, the importance of multidisciplinary collaboration in quantum research cannot be overstated. Engaging experts from different fields can spur innovative applications and theories.
Potential Technological Advancements
Potential technological advancements in quantum physics herald transformative effects. Here are some technologies under exploration:
- Quantum Computing: Companies like IBM and Google are spearheading the development of quantum computers. These devices could tackle complex computations currently infeasible by classical systems, impacting sectors like cryptography and drug discovery.
- Quantum Sensing: By harnessing quantum effects, these sensors can achieve higher precision and sensitivity. Applications range from medical diagnostics to navigation systems, which could enhance various fields and drive down costs.
- Quantum Cryptography: This technology promises impenetrable data protection. Quantum key distribution enables secure transmission channels, safeguarding against eavesdropping and cyberattacks.
"Understanding future directions in quantum physics is not just about scientific inquiry; it is about preparing for the next wave of technological breakthroughs that could redefine society."
To learn more about emerging theories, visit Britannica.
For ongoing discussions and community insights, refer to Reddit.
Explore additional resources on Wikipedia.
For updates from your network, check Facebook.
Culmination
The conclusion serves a pivotal role in synthesizing the insights presented throughout the article. It is essential for readers to grasp not only the broad concepts of quantum physics but also the intricate details that define its modern interpretation. In this exploration, we have raised key principles, significant phenomena, and emerging applications that illustrate the dynamic nature of the field.
Summary of Key Points
In summary, the article has covered several critical areas of quantum physics, including:
- Fundamental Principles: Wave-particle duality, uncertainty principle, and quantum superposition.
- Key Theories: Quantum mechanics, quantum field theory, and many-worlds interpretation.
- Important Phenomena: Quantum entanglement, quantum tunneling, and quantum decoherence.
- Applications: Quantum computing, cryptography, and advanced sensors.
- Challenges: Interpretational dilemmas and experimental limits.
- Future Directions: Emerging research areas and potential technological advancements.
These sections not only elucidate the foundational elements of quantum physics but also underscore its relevance in today’s scientific landscape. Each topic interconnects with one another, forming a holistic understanding of how quantum physics shapes modern technology and theoretical frameworks.
Implications for Future Research
The implications for future research in quantum physics are vast and transformative. As technology advances, there are significant opportunities to deepen our understanding of quantum behaviors and their applications. For instance:
- Researchers may explore novel quantum algorithms that can revolutionize computing efficiency.
- There is ongoing investigation into quantum cryptography techniques that promise enhanced security in information transfer.
- Researchers can further study the mysterious nature of quantum entanglement to unlock new communication technologies.
Given these potential pathways, future research holds the promise of not only expanding theoretical foundations but also translating these insights into practical innovations. Quantum physics encourages a rigorous examination of reality and challenges our conventional perceptions, making it a cornerstone of contemporary scientific inquiry.
"Quantum physics is not only a theory of matter and energy; it symbolizes a paradigm shift in how we understand the universe."