Exploring Relay Transport Mechanisms in Biology
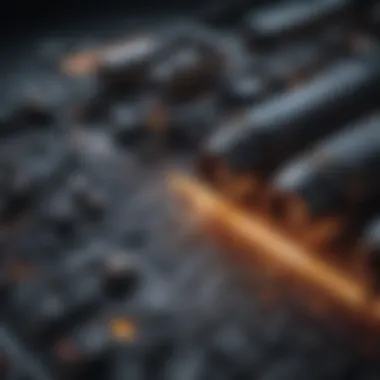
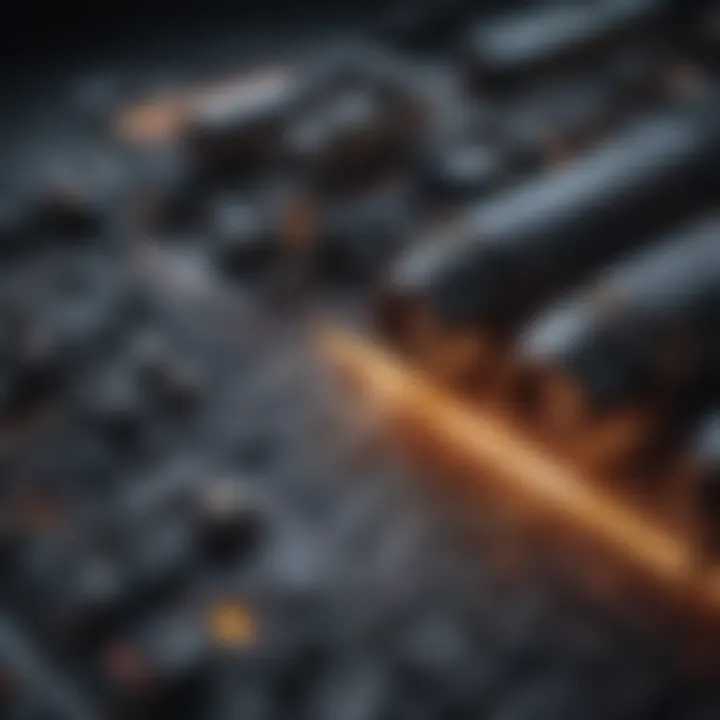
Intro
Relay transport serves as a critical mechanism in the fundamental processes of biological systems. This process involves the transfer of molecules across cellular membranes, facilitating essential functions like nutrient uptake, waste removal, and signal transduction. Understanding how these transport systems work can provide insight into the complex interactions at play within living organisms. Moreover, the implications of relay transport extend beyond basic biology into the realms of biotechnology and medicine, where similar principles can be applied for therapeutic innovations and diagnostic advancements.
As scientific exploration of relay transport mechanisms deepens, it becomes increasingly important to highlight their key features and applications. This article aims to unpack the intricacies of relay transport, discussing its diverse applications and potential future directions that can reshape our approaches in multiple fields. Through a detailed analysis, this examination will address the existing classifications of relay transport systems, their biological significance, and ongoing research efforts that may influence future applications.
By synthesizing current information on relay transport, this article serves as a comprehensive guide for various stakeholders including students, researchers, educators, and professionals. Readers can anticipate an exploration of core principles and practical ramifications of relay transport mechanisms.
Understanding Relay Transport
Relay transport is a fundamental process within biological systems, vital for maintaining cellular functions. Understanding this concept allows researchers to explore various cellular mechanisms that support life. It involves the movement of molecules across cellular membranes, which is essential for processes such as nutrient absorption, waste removal, and signaling. This efficiency in movement is crucial for the overall health of an organism.
The significance of relay transport cannot be overstated. Its mechanisms influence many cellular processes, affecting not just the cell's survival but also its ability to interact with other cells. In biotechnology, insights gained from studying relay transport can lead to innovations in drug delivery systems and therapeutic interventions. An understanding of these concepts will aid in the development of more effective treatments and improve our general knowledge of cellular functions.
Definition and Importance
Relay transport refers to the process by which substances move across cell membranes through specific transport mechanisms. This can happen passively, without energy, or actively, requiring cellular energy. The efficacy of relay transport greatly affects how well cells can carry out their functions. Substances such as ions, nutrients, and waste products rely on this transport for proper cellular operation.
The importance of studying relay transport lies in its widespread implications in both biology and biotechnology. For example, in medical settings, an understanding of these transport systems can lead to breakthroughs in drug design. By knowing how drugs move in and out of cells, it is possible to create medications that work more effectively. Moreover, relay transport plays a crucial role in maintaining homeostasis, allowing organisms to achieve balance in their internal environments.
Historical Context of Relay Transport Studies
The study of relay transport mechanisms has evolved over the past century. Initial research focused on simple transport processes that were primarily based on diffusion. However, as the field of cell biology advanced, scientists began to uncover complex systems involved in cellular communication and transport.
In the mid-20th century, major advancements were made in understanding the membrane’s role in transport mechanisms. The introduction of techniques like patch-clamp and fluorescent tagging allowed for detailed studies of how substances move across membranes. This research laid the groundwork for further exploration of specific transport proteins and their functions.
Today, scientists continue to build on this historical foundation. The integration of technology in relay transport studies has opened new avenues for research, allowing insights into molecular dynamics and transport efficiency. Continued exploration of relay transport will undoubtedly lead to advances that could enhance biotechnology and medicine, further highlighting its importance in contemporary research.
Mechanisms of Relay Transport
The mechanisms of relay transport are pivotal to understanding how substances move across cellular membranes. These transport processes are fundamental for various physiological functions in living organisms. Grasping how relay transport operates allows researchers and professionals to devise strategies for manipulating these processes, which can have significant implications in biotechnology and medicine.
The key mechanisms of relay transport include passive and active transport methods. Each serves distinct purposes and operates under different principles. Analyzing these mechanisms aids in identifying how cells maintain homeostasis, facilitate communication, and distribute resources effectively.
Types of Relay Transport
Passive Relay Transport
Passive relay transport refers to the movement of molecules across a membrane without the input of energy. This process relies on concentration gradients, meaning substances move from areas of high concentration to areas of low concentration until equilibrium is achieved. The primary advantage of passive transport is its efficiency; it does not consume metabolic energy, making it a favored method in many biological contexts.
One of the unique characteristics of passive relay transport is its reliance on physical barriers in the form of semi-permeable membranes. This allows essential nutrients to enter the cell while keeping harmful substances out. However, a limitation of passive transport is that it cannot move substances against their concentration gradient, which can be a crucial aspect in certain cellular functions.
Active Relay Transport
Active relay transport requires the input of energy, often from ATP, to move substances against their concentration gradients. This mechanism is essential for maintaining ion balances within cells, which is critical for various cellular processes. The energy dependency highlights its importance in situations where cells need to accumulate essential substances, such as ions, against natural diffusion.
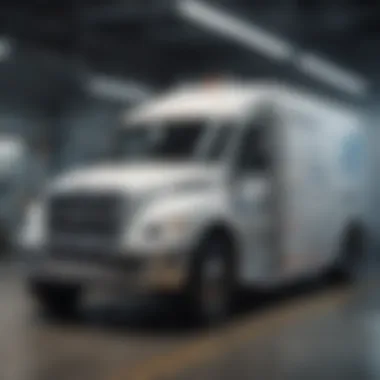
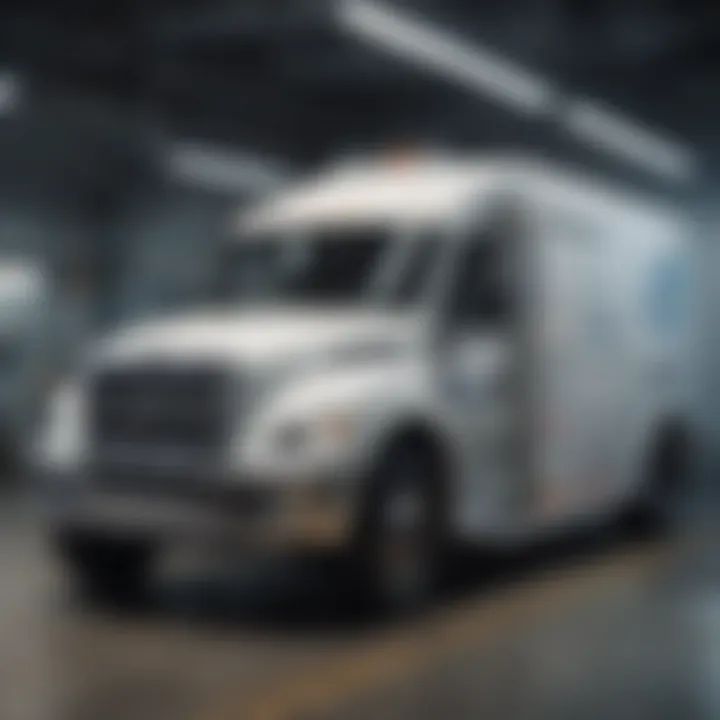
A key characteristic of active relay transport is the use of specific transport proteins, often referred to as pumps. These proteins change shape to transport molecules, which is a unique aspect compared to passive transport mechanisms. While active transport is highly effective for moving nutrients and removing waste, its energetic cost can limit the efficiency of cells, especially under conditions where resources are scarce.
Molecular Players in Relay Transport
Protein Channels
Protein channels are integral membrane proteins that facilitate the movement of ions and small molecules across the cell membrane. By providing a passageway, they contribute significantly to the process of relay transport. Their characteristic ability to selectively allow specific ions or molecules—like sodium or potassium—transforms them into essential components in maintaining cellular function.
The advantage of protein channels lies in their speed and selectivity, allowing for rapid transport in response to cellular signals. However, the regulation of these channels can be complex; improper functioning can lead to disorders, indicating their critical role in health.
Carrier Proteins
Carrier proteins play a significant role in the relay transport by binding to specific molecules and changing shape to shuttle them across the cell membrane. Unlike channels, they undergo conformational changes to transport substances, which can increase their efficiency in certain contexts.
A major strength of carrier proteins is their ability to transport both ions and larger molecules. However, they can be slower compared to protein channels due to the need for conformational changes, which may affect their overall contribution in situations requiring rapid transport.
Transport ATPases
Transport ATPases are energy-driven transport proteins that are crucial for active relay transport. They utilize the energy from ATP to actively transport ions across membranes, maintaining essential concentration gradients vital for cellular functions.
The main advantage of transport ATPases is their ability to move ions against their concentration gradients, making them indispensable for maintaining homeostasis. The downside can be their heavy energy requirement and the potential for malfunction if ATP production is inhibited, leading to severe repercussions for cellular viability.
Understanding these mechanisms helps in addressing various challenges in biotechnology and medicine while also paving the way for innovating solutions to minimize health issues.
Biological Contexts of Relay Transport
Understanding the biological contexts in which relay transport operates is pivotal for grasping its significance. Relay transport mechanisms are fundamental to maintaining cellular homeostasis and facilitating intercellular communication. Each cell type utilizes relay transport differently, which reflects their unique physiological roles. Appreciating the nuances of relay transport across different systems can lead to enhanced biotechnological applications and therapeutic strategies.
Relay Transport in Plant Cells
In plant cells, relay transport plays a crucial role in nutrient uptake and regulation. Plants depend on relay transport to absorb essential minerals like potassium, calcium, and magnesium from the soil. The transport of these ions occurs through specific protein channels situated in the plasma membrane.
Additionally, photosynthesis relies on the transport of various molecules, including water and carbohydrates, to ensure optimal energy production. The overall health and growth of the plant can be impacted by any disruption in these relay transport systems. Understanding how plants regulate these mechanisms can inform agricultural practices and crop enhancement initiatives.
Relay Transport in Animal Cells
Animal cells exhibit a different set of requirements for relay transport. Here, the mechanisms are vital for various functions, including nutrient absorption, waste removal, and cell signaling. Transport proteins in animal cells can engage in both passive and active relay transport to navigate the complex demands of ionic balance and molecular exchange.
For example, glucose transporters are essential for providing energy to cells. These transporters facilitate the entry of glucose into cells, thus supporting metabolic processes. Moreover, relay transport in neurons is critical for transmitting signals. Neurotransmitters rely on shuttle systems for their release and reuptake. This makes understanding these transport mechanisms essential for advancements in neuroscience and pharmacology.
Role in Cellular Signaling
Beyond material exchange, relay transport also underpins cellular signaling. This signaling is often initiated by external stimuli. The relay systems translate these signals into internal cellular responses, using various transport proteins and channels to facilitate the process.
One notable example is the role of calcium ions in muscle contraction. When stimulated, calcium is released stores, enabling the process of muscle contraction to occur efficiently. Another instance can be observed in hormone signaling, where specific transport proteins enable the hormonal response to emerge from the cellular interior. This integration of relay transport into signaling networks showcases its complexity and importance within biological systems.
Understanding the biological contexts of relay transport is essential. It enhances insights into cellular mechanics, agricultural technologies, and medical applications.
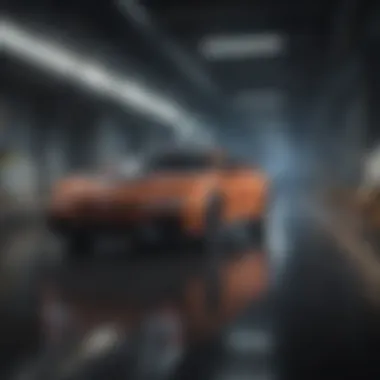
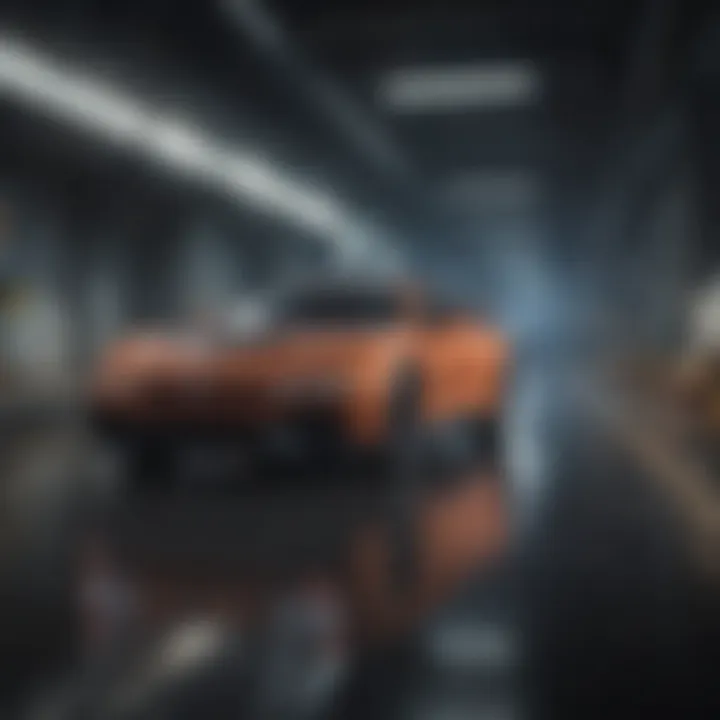
Applications of Relay Transport in Biotechnology
Relay transport has a significant role in biotechnology, impacting various fields such as genetic engineering, drug delivery systems, and synthetic biology. By harnessing the mechanisms of relay transport, scientists can develop more efficient methods for cellular processing. This section delves into two key areas of application: engineering transport systems and their use in drug delivery.
Engineering Transport Systems
Genetic Modifications
Genetic modifications involve altering an organism's genetic material to achieve desired traits. In the context of relay transport, this method is crucial for enhancing transport efficiency. For instance, specific genes can be modified to increase the expression of transport proteins, leading to improved substrate uptake. This results in more effective metabolic pathways within the engineered organisms.
A main characteristic of genetic modifications is the precision it offers. Researchers can target exact locations within a genome to induce changes. This high level of specificity makes genetic engineering a popular choice in biotechnology. However, it also brings ethical considerations and potential regulatory challenges.
Unique features of genetic modifications include the ability to increase resistance to environmental stresses and to boost product yields in industrial applications. While these modifications can present advantages, like improved transport efficiency and enhanced growth rates, they must be balanced against the risks of unintended effects on the organism's biology.
Protein Engineering
Protein engineering focuses on designing and modifying proteins to improve their functions. Within relay transport, protein engineering allows for the development of bespoke transport proteins tailored to specific needs. Researchers can create proteins with enhanced binding affinity for substrates or better stability under varying conditions.
This area of research highlights the customizable nature of protein engineering. Being able to adjust protein properties allows for innovative applications in various biotechnological settings. One advantage is that it can help create systems that mimic natural transport processes. Yet, it comes with its drawbacks, as engineered proteins may not always perform as predicted.
A unique aspect of protein engineering is the potential for high-throughput screening. Large libraries of engineered proteins can quickly be tested for effectiveness in relay transport. This method can accelerate the development of new transport systems while minimizing time and costs.
Relay Transport in Drug Delivery
The use of relay transport in drug delivery is one of the most promising applications in biotechnology today. The ability to transport drugs effectively to target areas within the body is vital for enhancing therapeutic efficacy. Relay transport systems can be designed to facilitate the controlled release of pharmaceuticals, ensuring that they reach their intended destinations in a timely manner.
These transport systems can significantly improve the specificity of drug delivery. For instance, by utilizing proteins that naturally transport nutrients, researchers can develop systems that allow for targeted delivery of medications. This can potentially reduce side effects and increase the effectiveness of treatments.
Moreover, relay transport mechanisms can be incorporated into nanoparticles, improving the efficiency of drug uptake in cells. This innovative approach optimizes how drugs interact with their targets, making it a valuable strategy in modern medicine.
In summary, applications of relay transport in biotechnology are vast. They provide avenues for improving transport systems, enhancing drug delivery, and developing new therapeutic strategies, which are essential for advancing the field.
Challenges and Limitations
Relay transport mechanisms, while crucial for various biological processes, present significant challenges and limitations that must be addressed. Understanding these factors is pivotal for advancing both research and practical applications in the field. By delving into technical difficulties and biological constraints, we can paint a clearer picture of the hurdles researchers face and the implications for future endeavors.
Technical Difficulties in Study
Studying relay transport is inherently complex due to the intricate nature of biological systems. Several key technical difficulties arise in this context:
- Isolation of Components: Obtaining pure samples of proteins and other transport molecules can be challenging. These components are often embedded in cellular membranes and require intricate techniques for extraction.
- Real-Time Observation: Capturing the dynamic processes of relay transport in real-time necessitates advanced imaging techniques. Microscopy methods, while powerful, often struggle with resolution and temporal limitations.
- In Vitro vs. In Vivo: Experiments carried out in laboratory conditions (in vitro) might not fully translate to living organisms (in vivo). The controlled environment of in vitro studies can obscure the real biological interactions that occur within living cells.
These challenges raise important questions about the reliability and applicability of research findings. Continued development of innovative techniques will be crucial for overcoming these obstacles, fostering a more accurate understanding of relay transport mechanisms.
Biological Constraints
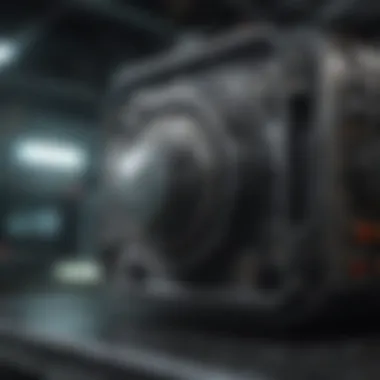
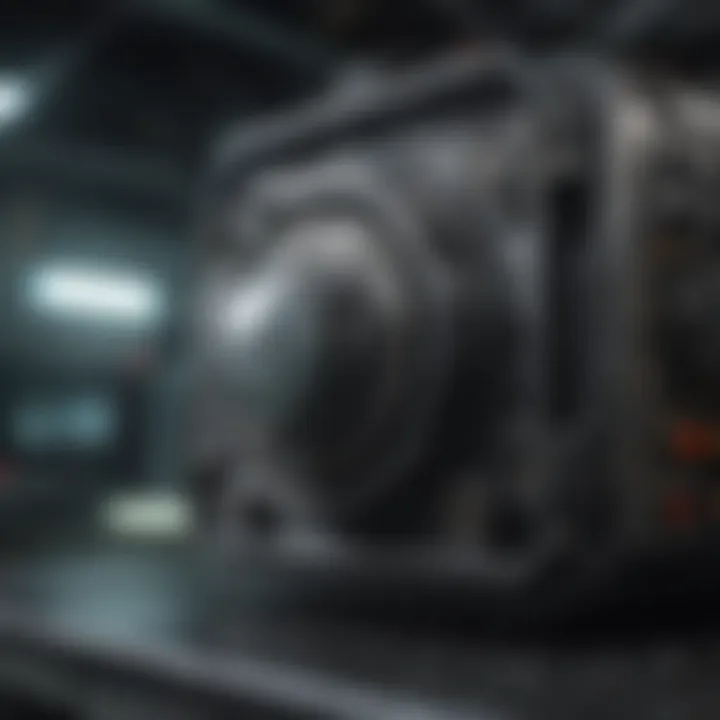
In addition to technical difficulties, biological constraints play a significant role in the study of relay transport. These factors often limit both the study and application of the concepts in real-world scenarios:
- Variability of Biological Systems: Different organisms and cell types can express unique relay transport systems, leading to variations in function and efficiency. This diversity complicates the extrapolation of results from model organisms to humans.
- Regulatory Mechanisms: Cellular signaling pathways intricately regulate relay transport. Changes in environmental conditions or cellular states can impact these systems, making it difficult to establish consistent models.
- Pathological Conditions: Various diseases affect the normal function of relay transport mechanisms. Understanding these effects is essential for developing effective therapies, but the interplay of different disease states adds layers of complexity to the study.
Addressing these biological constraints requires an interdisciplinary approach, leveraging insights from molecular biology, biochemistry, and systems biology. The collaborative effort will not only enhance our understanding of relay transport but also open doors to innovative applications in medicine and biotechnology.
Future Directions in Relay Transport Research
Relay transport remains a pivotal area of research in cellular biology and biotechnology. As scientists delve deeper into cellular mechanisms, future directions in this field promise significant advancements. The exploration of relay transport systems can improve our understanding of complex biological processes. Moreover, it can lead to the development of novel biotechnological applications.
Technological progress will enable researchers to visualize and manipulate relay transport mechanisms with greater precision. This opens up new possibilities for medical treatments and genetic engineering. By addressing current limitations, future research can provide insights that lead to innovative solutions in various fields.
Technological Advances
Imaging Techniques
Imaging techniques are vital for studying relay transport in real-time. These methods allow scientists to observe movement across membranes at a cellular level. The key characteristic of imaging techniques is their ability to provide visual data, making the dynamics of transport systems more comprehensible.
One popular method is fluorescence microscopy. This technique utilizes fluorescent markers to label transport proteins. Through real-time imaging, researchers can track these proteins as they move across cellular membranes. The unique feature of imaging techniques lies in their non-invasive nature. They minimize disruption to the cells while providing detailed insights into transport mechanisms.
However, there are disadvantages. The interpretation of imaging data can be complex and often requires advanced analytical skills. Moreover, the resolution might limit the observation of small structures involved in relay transport. Despite these challenges, imaging techniques remain an essential tool for advancing research in this field.
Computational Models
Computational models play a significant role in predicting and analyzing relay transport mechanisms. They help researchers simulate transport processes, providing valuable insights into how different variables affect these systems. A key characteristic of computational models is their ability to integrate vast amounts of data, making them a beneficial tool for simulation.
Through algorithms and mathematical frameworks, these models can mimic the dynamic nature of cellular transport. One unique feature is their predictive power. By analyzing various scenarios, researchers can anticipate the outcomes of different transport conditions. This is especially useful when considering the design of new drugs aimed at enhancing or inhibiting transport processes.
However, computational models have limitations. The accuracy of these models often depends on the quality of input data and the assumptions built into the models themselves. Misleading results can result from over-simplified assumptions. Despite these potential pitfalls, computational models offer unparalleled insights and have become a cornerstone for future research.
Interdisciplinary Approaches
Interdisciplinary approaches are crucial for enhancing our understanding of relay transport. Collaboration between researchers from various fields can lead to innovative discoveries. For instance, biologists can work alongside engineers to develop new transport systems.
Combining insights from physics, chemistry, and biology can lead to a more holistic understanding. Such collaboration fosters the development of cutting-edge technologies, such as targeted drug delivery systems. It allows for the merging of theoretical knowledge with practical applications. This synergy can drive research in relay transport to new heights.
The End
The conclusion is a vital part of this article as it synthesizes the complexity of relay transport mechanisms and their various implications for biological systems and biotechnological advancements. It underscores the principle mechanisms discussed, reiterating their relevance in cellular behaviors, plant and animal functions, and the overall understanding of biological transport systems. Furthermore, the conclusion provides a final reflection on the potential of relay transport in medical applications, particularly in drug delivery and genetic engineering.
Recapitulation of Key Points
In this article, we explored several key elements surrounding relay transport:
- Definition and importance of relay transport as a fundamental biological process.
- The mechanisms involved, including types of relay transport and molecular players such as protein channels and transport ATPases.
- The biological contexts that illustrate the role of relay transport in both plant and animal cells, as well as its significance in cellular signaling.
- Applications in biotechnology, from engineering transport systems through genetic modifications to the role of relay transport in drug delivery.
- Challenges faced in the study of relay transport which include technical difficulties and biological constraints.
- Future directions in relay transport research that consider technological advances and interdisciplinary approaches.
These topics form the basis for understanding how relay transport operates within various contexts and its potential for future innovations in science and medicine.
Implications for Future Research
The exploration of relay transport presents several avenues for future research that are compelling. As our understanding of biological transport systems grows, researchers can apply these insights to address real-world problems. Specific implications for future investigations include:
- Technological Innovation: Advancements in imaging techniques and computational models could provide deeper insights into the dynamics of relay transport, enhancing the precision of studies in this field.
- Interdisciplinary Collaboration: Bridging disciplines, such as combining biology with materials science or engineering, may lead to innovations in transport systems, particularly in drug delivery mechanisms and targeted therapies.
- Personalized Medicine: With a refined understanding of transport mechanisms, future research may focus on individual variability in transport efficiency, improving medical treatments tailored for specific patient profiles.
- Biotechnological Applications: Further studies could explore novel applications of relay transport in bioengineering, potentially leading to the development of more efficient genetic modifications or synthetic transport systems that better mimic natural processes.