Exploring Supercoiled DNA Ladders by Invitrogen
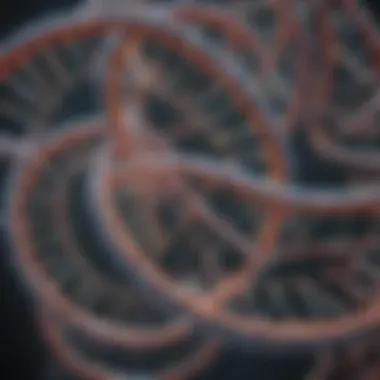
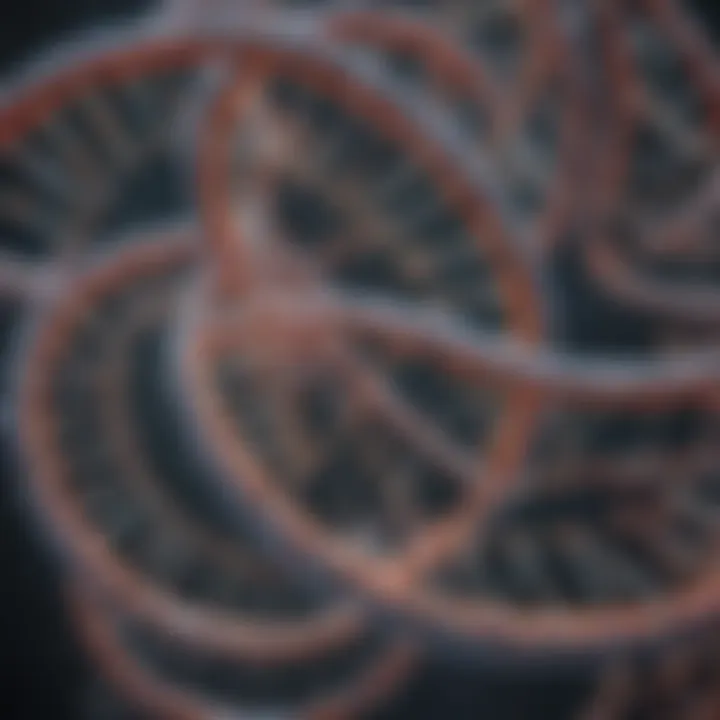
Intro
Supercoiled DNA ladders are crucial tools in the field of molecular biology, particularly for genetic analysis and electrophoresis. This article delves into the fascinating characteristics of supercoiled DNA ladders produced by Invitrogen. By understanding their molecular properties and the techniques used to take advantage of these constructs, researchers can enhance their laboratory practices. The supercoiled form of DNA provides unique advantages for applications in research due to its compact structure, which allows for precise molecular weight determination and improved stability during experimental procedures.
To understand the significance of supercoiled DNA ladders, it is essential to consider their role in genetic analysis. They serve not only as markers in gel electrophoresis, but also as reference standards that enable the consistent evaluation of DNA fragments. This article offers a comprehensive guide, discussing various aspects including their synthesis, functionalities, and applications in contemporary scientific research.
Research Highlights
Overview of Key Findings
The examination of supercoiled DNA ladders leads to several key insights. Firstly, the structural characteristics of supercoiled DNA grant these ladders unique advantages over linear counterparts. This structural integrity enhances the stability and reproducibility of experimental results. Moreover, the supercoiling effect allows for better resolution in gel electrophoresis, facilitating accurate determination of molecular weights.
Furthermore, Invitrogen's supercoiled DNA ladders are designed for optimal performance. This includes considerations such as the DNA concentration, buffer systems, and proper loading techniques. Each factor contributes to successful outcomes in genetic analysis and molecular experiments.
Significance of the Research
Understanding supercoiled DNA ladders goes beyond mere academic interest. The insights gained from research contribute directly to practical applications in genetic engineering, forensics, and biotechnology. The ability to accurately determine molecular weights improves the analysis of DNA samples, which is critical in various fields such as genomics and proteomics.
"Supercoiled DNA ladders are indispensable tools in molecular biology. Their precision aids researchers in unraveling complex genetic information."
Techniques for Utilization
Supercoiled DNA ladders are utilized in several techniques that enhance their usefulness:
- Gel Electrophoresis: Supercoiled DNA can migrate differently compared to linear DNA in an electric field, allowing for better separation and clarity in gel analysis.
- Molecular Weight Determination: Researchers employ these ladders as benchmarks for estimating the sizes of unknown DNA fragments.
By employing these techniques, researchers can ensure that their studies yield accurate and reliable results.
Quality Assurance Measures
To maintain the integrity of supercoiled DNA ladders, specific quality assurance measures are essential. Proper storage conditions, consistent handling, and regular quality assessments guarantee that these DNA ladders perform as expected in various experiments. Training personnel on best practices significantly reduces the risk of contamination, ensuring that the research outcomes are credible and reproducible.
Prologue to Supercoiled DNA
In the space of molecular biology, supercoiled DNA serves as more than just a structural formation. Supercoiling is crucial to the functionality of DNA. Understanding this structure is essential for anyone involved in genetic research, as it influences not only the behavior of the DNA in biological processes but also the methodologies employed in laboratory environments.
Definition and structural characteristics
Supercoiled DNA refers to the coiling of the DNA double helix within a confined space. This structure arises due to the twisting and bending of the helix, leading to a more compact form. Supercoils can be categorized into two types: positive and negative. Positive supercoiling occurs when the DNA is overwound, while negative supercoiling happens when it is underwound. Both forms have significant implications in biological processes, especially in the context of DNA replication and transcription.
The structural characteristics of supercoiled DNA include its ability to exist in a compact state, allowing it to be more readily packed within the confines of a cell nucleus. The supercoiling can facilitate the accessibility of various enzymes involved in replication and transcription, which is pivotal in processes like gene expression. Notably, supercoiled DNA's topological state depends on several factors, including temperature and ionic conditions.
Importance in molecular biology
The significance of supercoiled DNA in molecular biology cannot be overstated. It plays a vital role in various biological processes such as replication, transcription, and packaging in cellular compartments. For instance, during DNA replication, the unwinding and rewinding of DNA creates torsional strain. Supercoiling helps manage this strain, enabling enzymes to function properly.
Furthermore, supercoiling is integral in the design of molecular experiments and genetic engineering methods. This structure is often utilized in techniques such as PCR and gene cloning. The ability to manipulate the supercoiled state of DNA allows researchers to achieve specific experimental outcomes. In summary, supercoiled DNA is not merely a structural feature; it is a pivotal element that underlines both the construct of our genetic material and its practical application in contemporary biological research.
Supercoiled DNA Ladder: An Overview
Supercoiled DNA ladders serve a crucial role in molecular biology, acting as both standards for size estimation and tools for various applications in genetic research. Understanding these ladders is vital for effectively utilizing them in laboratory settings. They offer distinct advantages in ensuring the accuracy and repeatability of experimental results, making them a common resource among researchers.
Description of a DNA ladder
A DNA ladder consists of a set of DNA fragments of known sizes that researchers utilize as reference points during electrophoresis. It allows the assessment of the molecular weight of unknown samples when compared against these known standards. Supercoiled DNA ladders are particularly efficient because they run faster during electrophoresis compared to their linear counterparts due to their compact structure. This means that even small amounts of DNA can be analyzed effectively, enhancing the sensitivity of experiments.
The composition of a DNA ladder typically includes fragments of varying lengths, often ranging from a few hundred base pairs to several kilobase pairs. When formulated by Invitrogen, the supercoiled DNA ladder provides precise fragment sizes, ensuring reliable results across multiple applications.
Comparison with linear DNA
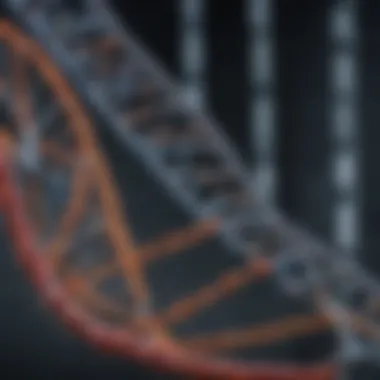
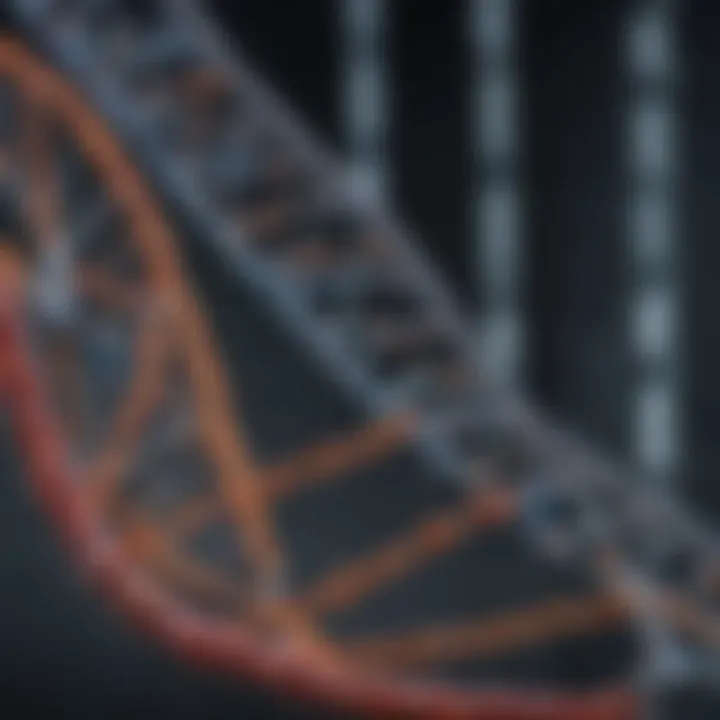
Supercoiled DNA exhibits unique characteristics compared to linear DNA, primarily in terms of structure and electrophoretic behavior. One of the main differences is that supercoiled DNA is more compact than linear DNA, which means it can migrate faster through gel matrices during electrophoresis. This fast migration allows for better resolution during analysis, which is essential in various molecular techniques.
In terms of stability, supercoiled DNA is often less prone to degradation. This added stability is critical when samples are stored for long periods or transported over significant distances. In contrast, linear DNA can be more susceptible to shearing and degradation if not handled correctly.
"The advantages of supercoiled DNA ladders in molecular biology cannot be overstated. They not only provide accuracy in size estimation but also enhance the efficiency of various genetic techniques."
The differences in migration patterns between supercoiled and linear DNA can significantly affect data interpretation. Researchers must consider these factors when designing experiments and selecting appropriate standards for their specific applications. Using supercoiled ladders allows for more precise measurements and comparisons in electrophoretic studies.
Production of Supercoiled DNA at Invitrogen
The production of supercoiled DNA at Invitrogen is a fundamental topic that covers significant aspects of molecular biology. This process is essential because supercoiled DNA serves as a crucial tool in various applications, particularly in genetic engineering and molecular cloning. Understanding how this DNA is produced provides insights into its stability, functionality, and overall quality. By mastering production techniques, scientists can ensure that the supercoiled DNA meets the rigorous demands of modern research and applications.
Methodology used in production
The methodology for producing supercoiled DNA at Invitrogen involves several intricate steps. First, the DNA must be extracted and purified from various sources, often bacteria such as E. coli. The plasmids are then introduced into host cells, where they replicate. The process begins with the isolation of the plasmid DNA, followed by purification that removes contaminants. Then, enzymes play a vital role, particularly topoisomerases, which introduce supercoiling through the manipulation of DNA topology. This becomes crucial because it directly influences the physical properties of the DNA.
Through these procedures, Invitrogen ensures high-quality yields of supercoiled DNA. Factors such as temperature and time are carefully controlled to optimize the yield and to maintain the structural integrity of the DNA.
Quality control measures
Quality control is a non-negotiable aspect in the production of supercoiled DNA at Invitrogen. The company employs a range of techniques to assess the integrity and functionality of the DNA before it is released for applications. Among these measures are gel electrophoresis and spectrophotometry.
"Quality assurance is essential to ensure that the supercoiled DNA produced is of high fidelity, essential for downstream applications."
These methods check for correct conformation and purity, determining the absence or presence of any contaminants that may impact the performance of the DNA. This detailed attention to quality control helps to guarantee that the supercoiled DNA used in research will yield reliable and reproducible results. Additionally, batch testing is usually performed to establish consistency and reliability across different production runs. Such stringent measures highlight Invitrogen's commitment to providing dependable products in the competitive field of molecular biology.
Physical Properties of Supercoiled DNA
The physical properties of supercoiled DNA play a crucial role in understanding its function and application in molecular biology. Supercoiling is an intrinsic feature of DNA that affects its stability and behavior during experiments. The key characteristics, such as elasticity and electrophoretic mobility, make it a valuable tool in various research and diagnostic settings. A deeper comprehension of these physical attributes allows researchers to optimize their use of supercoiled DNA ladders for specific experimental needs.
Elasticity and stability
The elasticity of supercoiled DNA is a fundamental aspect that contributes to its overall stability. Unlike linear DNA, supercoiled DNA is more compact, which results from its tightly wound structure. This compact nature means it can better withstand mechanical stress without breaking. Such elasticity is essential during various laboratory techniques, where DNA might be subjected to forces, such as during gel electrophoresis or when undergoing manipulation in cloning processes.
Stable supercoiled DNA ensures that the genetic material maintains its integrity during storage and handling. Invitrogen's protocols for producing supercoiled DNA incorporate mechanisms that enhance this stability. Enhanced stability not only prolongs the shelf life of the DNA but also guarantees that it performs reliably in experimental setups.
Moreover, this stability influences the binding interactions between DNA and proteins. Understanding the elasticity and stability of supercoiled DNA can inform how it interacts with other molecules, which is critical for gene expression studies and other molecular biology applications.
Electrophoretic mobility
Electrophoretic mobility refers to the movement of DNA within an electric field during electrophoresis. This property is vital for separating different DNA constructs based on size and shape. Supercoiled DNA exhibits distinct mobility patterns compared to linear DNA. It generally moves faster through the gel matrix due to its smaller effective cross-section. This property allows researchers to use supercoiled DNA ladders as molecular weight standards in gel electrophoresis.
In electrophoretic analysis, understanding the mobility of supercoiled DNA enables precise measurement of DNA fragments. By comparing the migration patterns of samples against known supercoiled DNA markers, researchers can accurately determine the size of unknown DNA samples.
The ability to differentiate DNA forms based on electrophoretic mobility enhances the reliability of genetic analyses. This is particularly useful in applications such as PCR, genetic fingerprinting, and gene cloning, providing critical insights into the genetic material being studied.
Understanding the physical properties of supercoiled DNA enhances its utility in laboratory practice, making it an indispensable tool for researchers.
In summary, recognizing the physical properties of supercoiled DNA, particularly its elasticity and electrophoretic mobility, is essential. These characteristics not only dictate how supercoiled DNA behaves in various experimental settings but also determine its applications in broader molecular biology fields.
Applications of Supercoiled DNA Ladders
Supercoiled DNA ladders play an essential role in various molecular and genetic studies. Their applications extend from laboratory practices to significant contributions in therapeutic developments. By understanding the specific uses of these DNA ladders, researchers can optimize their experimental outcomes and advance their scientific endeavors.
Molecular weight standard in electrophoresis
In electrophoresis, supercoiled DNA ladders serve as reliable molecular weight standards. This is crucial because accurate sizing of DNA fragments is a fundamental part of genetic analysis. The supercoiled form provides distinct bands on agarose gels, allowing for precise comparison against experimental samples. By knowing the molecular weights of these standards, researchers can effectively determine the sizes of DNA fragments produced during reactions such as restriction digest or PCR amplification.
Using supercoiled DNA ladders, labs can:
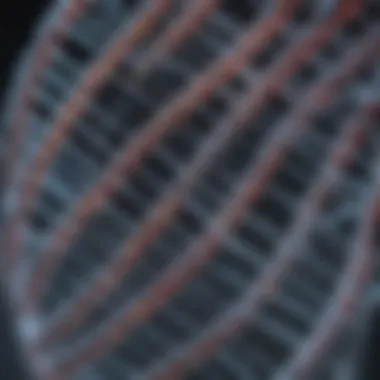
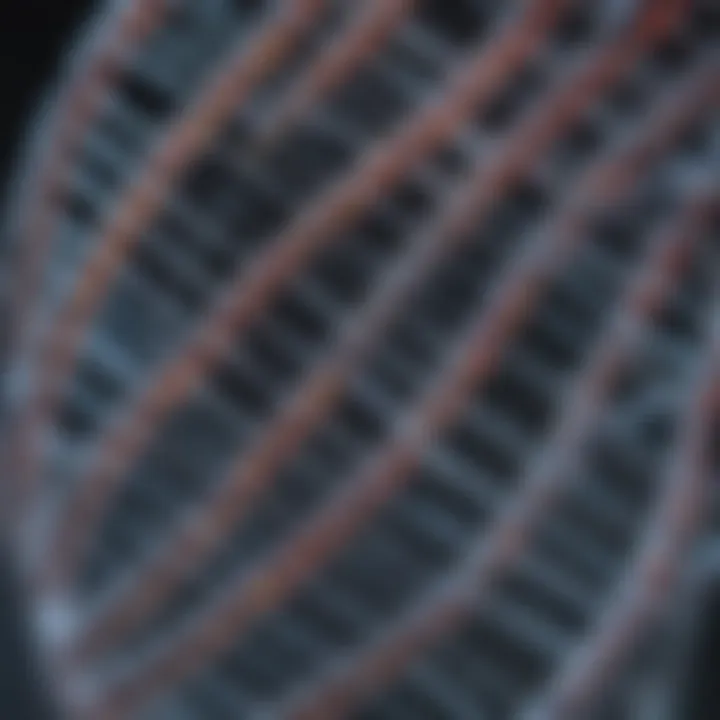
- Obtain clear and sharp bands for accurate measurements.
- Ensure reproducibility since the ladders maintain consistent structure and mobility.
- Facilitate visual assessment of results by comparing unknown samples with known standard sizes.
"The supercoiled DNA ladder is like a ruler for geneticists, allowing them to gauge the size of their DNA fragments accurately."
Gene cloning and transfection studies
Gene cloning is another area where supercoiled DNA ladders are invaluable. In cloning procedures, they can be used to vector DNA, assisting in the insertion of genes into host organisms. The infectivity or transformation efficiency can depend on the supercoiled state of the DNA. Using supercoiled forms enhances the uptake by cells during the transfection processes.
Benefits of using supercoiled DNA in cloning include:
- Higher transformation rates, maximizing the chances of successful gene uptake.
- Better stability compared to linear forms, making supercoiled DNA more effective in laboratory settings.
PCR and genetic fingerprinting
Polymerase chain reaction (PCR) and genetic fingerprinting utilize supercoiled DNA ladders for informative outcomes in identity testing and genetic diversity studies. In PCR, the amplification of target DNA is often evaluated using a DNA ladder to confirm the expected product sizes.
Additionally, genetic fingerprinting can benefit from the use of these ladders in the following ways:
- Distinct band patterns help in identifying unique genetic markers.
- Comparison of individuals or strains can reveal polymorphisms, enabling studies in biodiversity and forensic analysis.
Supercoiled DNA ladders thus support various crucial applications across molecular biology, further emphasizing their significance in research and education.
Experimental Techniques Utilizing Supercoiled DNA
Understanding the experimental techniques that employ supercoiled DNA is essential for anyone involved in molecular biology. These methods are central to various applications, especially in genetic research and analysis. Supercoiled DNA has distinct advantages in the laboratory, particularly regarding its stability and compactness. The ability to visualize and manipulate supercoiled forms of DNA is crucial for accurate analysis and experimentation.
Preparation of agarose gel electrophoresis
Agarose gel electrophoresis is a widely used technique for separating DNA molecules based on their size. The preparation process begins with the dissolving of agarose powder in a buffer solution. This mixture is then heated until it becomes a clear liquid. Once it cools slightly, the agarose is poured into a gel casting tray to form a solid gel. After solidification, wells are formed to allow samples to be loaded.
The gel’s concentration determines the resolution capacity. Higher concentrations separate smaller DNA fragments more efficiently, while lower concentrations favor larger fragments. Once prepared, the gel is placed in an electrophoresis chamber filled with the same buffer solution. This chamber will facilitate the migration of the supercoiled DNA under an electric field.
Running and visualizing DNA
Running the DNA samples through the agarose gel occurs after loading them into the wells. An electric current is applied, causing the negatively charged DNA to migrate towards the positive electrode. The supercoiled DNA moves faster than linear DNA due to its compact structure. This property allows for a clearer distinction during separation.
After the run is complete, visualization is achieved by staining the gel with a dye, such as ethidium bromide. This dye intercalates with the DNA, emitting fluorescence under ultraviolet light. Care is needed during this procedure, since exposure to UV light can cause DNA damage. This step allows researchers to analyze DNA band patterns effectively, which provide essential information about the size and integrity of the DNA.
The use of agrose gel electrophoresis alongside supercoiled DNA is crucial in various molecular biology applications, facilitating genetic fingerprinting and cloning studies.
Limitations of Supercoiled DNA Applications
Understanding the limitations of supercoiled DNA ladders is crucial for researchers and practitioners alike. Despite their advantages, these molecules possess inherent challenges that can impact their effectiveness in various applications. This section will explore key limitations, shedding light on aspects such as degradation, stability, and contamination issues that might arise during handling and experimental use.
Degradation and stability concerns
Supercoiled DNA, while often preferred for its compact structure and functionality, is not free from degradation risks. Exposure to excessive temperatures, shear forces, or inappropriate storage conditions can lead to the breakdown of these molecules.
- Stable Environment: Ideally, supercoiled DNA should be stored at low temperatures—typically in a -20°C freezer—to minimize degradation risk.
- Handling Procedures: It is essential to handle the DNA with care to prevent mechanical shearing. This can occur with pipetting or centrifugation if performed too vigorously.
The instability of supercoiled DNA can drastically affect experimental outcomes. For instance, in gene transfer experiments, degraded DNA may not replicate correctly, leading to unreliable results. Therefore, understanding these risks and employing best practices in handling and storage is necessary.
Potential contamination issues
Contamination is another significant concern when working with supercoiled DNA ladders. The introduction of unwanted substances during the amplification, extraction, or application phases can compromise results.
- Sources of Contamination: Contaminants can include nucleases, primers, reagents, and even the environment in which the experiments are conducted. Nucleases, in particular, can degrade DNA, making it crucial to maintain a clean workspace.
- Preventive Measures: To reduce the risk of contamination, using sterile equipment and reagents is essential. Employing techniques such as the aseptic technique during handling can minimize the introduction of contaminants.
Notably, contamination can lead to false positives or negatives in assays, undermining the reliability of genetic analyses. Thus, recognizing these potential issues surrounding supercoiled DNA will help researchers implement strategies to mitigate them.
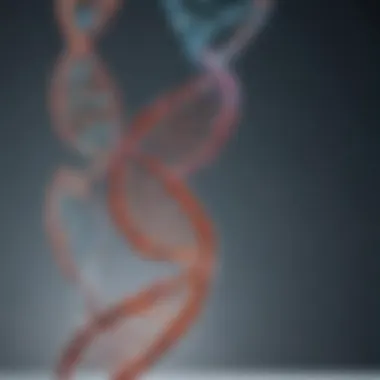
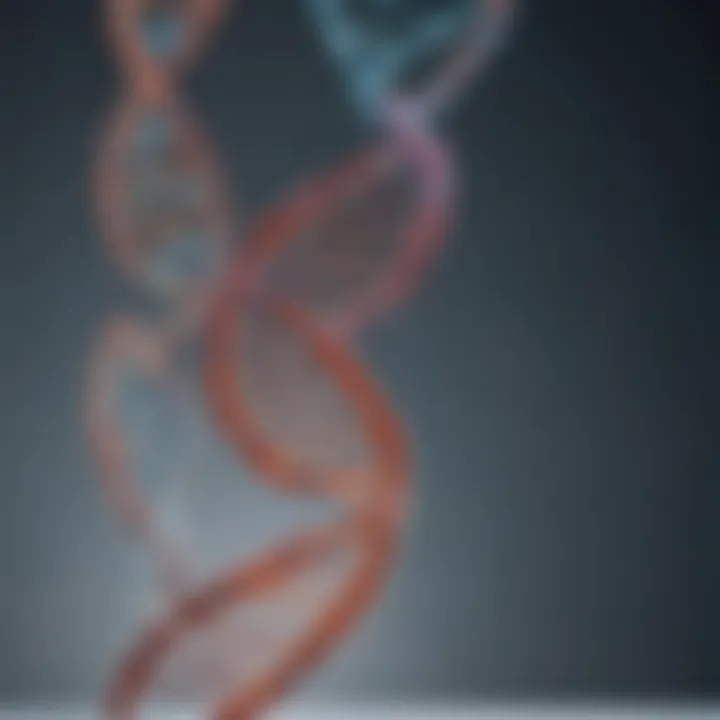
"The robustness of supercoiled DNA ladders is often tempered by their susceptibility to degradation and contamination; therefore, diligence is paramount in all phases of handling these biomolecules."
By acknowledging the limitations, researchers can take proactive steps to enhance their experimental designs and improve outcomes.
Advancements in Supercoiled DNA Research
The field of molecular biology continues to evolve with significant advancements in supercoiled DNA research. These developments not only enhance our understanding of DNA's structural properties but also improve its applications in various scientific domains. This section delves into the importance of innovations in production techniques and the enhanced applications in synthetic biology. Each breakthrough contributes to improved research methodologies and practical applications, offering a more robust understanding of genetic material in modern science.
Innovations in production techniques
Innovations in the production of supercoiled DNA are critical. Advances in purification methods have led to higher yields and greater purity of DNA samples. Techniques such as affinity chromatography allow for more precise separation of supercoiled DNA from contaminants. These innovations ensure that researchers work with the highest quality DNA, ultimately leading to more reliable experimental results.
Moreover, the development of new expression systems has also been beneficial. Systems using bacterial hosts can optimize the efficiency of supercoiled DNA production. Improved plasmid design, such as the incorporation of strong promoters, enhances the replication and expression of the desired genes. These modern approaches facilitate a faster turnaround in producing DNA ladders that can be used in various experiments.
Key advancements include:
- Use of high-throughput screening methods
- Genetic modifications for higher yield
- Automation of DNA extraction and purification processes
"The evolution of production techniques makes supercoiled DNA more accessible for various applications, thus fostering innovation in research."
Enhanced applications in synthetic biology
Synthetic biology represents a burgeoning area where supercoiled DNA plays a fundamental role. Enhanced applications of supercoiled DNA ladders allow for more sophisticated genetic modifications, including CRISPR-Cas9 systems. This technology relies heavily on the precise accuracy that supercoiled DNA provides when being used as a template for gene editing.
Additionally, supercoiled DNA serves as an essential tool for creating complex biological systems. Its stable structure enables the development of genetically engineered organisms designed for specific tasks, such as bioremediation or biofuel production. As researchers harness these capabilities, the interdisciplinary aspects of synthetic biology become increasingly evident.
Advantages in synthetic biology include:
- Creation of specialized organisms for environmental applications
- Improved gene delivery systems in therapeutic research
- Enhanced genetic circuits for better control over biological functions
The ongoing advancements in both production techniques and applications in synthetic biology highlight the dynamic nature of supercoiled DNA research. These innovations pave the way for future breakthroughs, impacting fields such as genetic engineering and therapeutic research. As the disciplines intersect, the possibilities for application expand exponentially.
Future Implications of Supercoiled DNA Use
The implications of supercoiled DNA use extend well beyond immediate laboratory applications. As various fields of biology and medicine progress, understanding these implications becomes crucial. Supercoiled DNA's inherent properties allow for advancements in genetic engineering and therapeutic research, reinforcing its significance in current and future studies. This section delves into these areas, detailing their relevance and potential.
Impact on genetic engineering
Supercoiled DNA is a major tool in the realm of genetic engineering. Its compact and stable structure offers numerous advantages when manipulating genes. The supercoiling allows for easier insertion and expression of foreign genes within host organisms.
- Efficient Transformation: The ability of supercoiled DNA to enter cells more readily enhances transformation efficiency. This is especially true for bacteria, making it an ideal candidate for cloning and gene expression studies.
- Enhanced Gene Delivery: In organisms, supercoiled DNA can improve gene delivery systems. It enables more precise targeting and reduces the risk of insertional mutagenesis during gene therapy applications.
- Versatile Application: Researchers utilize supercoiled DNA for various techniques, including CRISPR and transgenic organism creation. Both methods benefit from the efficiency and effectiveness brought by supercoiling.
Role in therapeutic research
Supercoiled DNA's applications also play a pivotal role in therapeutic research. In this context, its utility spans numerous therapeutic avenues, including cancer treatment and vaccine development.
- Gene Therapy: By delivering therapeutic genes in a stable and efficient manner, supercoiled DNA has the potential to treat genetic disorders. The proper delivery of these genes can lead to the restoration of normal function in affected cells.
- Cancer Treatment: In some cancer research contexts, supercoiled DNA serves as a vehicle for delivering therapeutic agents directly to tumor sites. This localized approach minimizes systemic side effects while maximizing treatment efficacy.
- Vaccine Development: With the rise of DNA vaccines, supercoiled forms are being used to enhance immune response. These vaccines leverage the body’s own cellular machinery to produce antigens, paving the way for more effective immunization strategies.
"The future of supercoiled DNA in science lies not only in its current applications but also in its continual adaptation to emerging technologies."
Overall, the future implications of supercoiled DNA use highlight its potential to revolutionize biological research and therapy. As science advances, its role will likely expand, further emphasizing the need for rigorous study and understanding of this important molecule.
End
The conclusion marks an essential part of the article, as it encapsulates the critical insights and implications of supercoiled DNA ladders produced by Invitrogen. This section synthesizes the findings discussed throughout the article, providing clarity on the role these DNA constructs play in molecular biology and genetic analysis.
Summary of key findings
The research outlined several key points regarding supercoiled DNA:
- Structural Properties: Supercoiled DNA offers unique structural advantages that enhance its stability during experiments compared to its linear counterparts. This property is vital for ensuring accurate results in various molecular applications.
- Applications: From serving as molecular weight standards in electrophoresis to its use in gene cloning and PCR, supercoiled DNA ladders demonstrate versatile applications across multiple research avenues.
- Quality Control: The methodology used in the production of supercoiled DNA at Invitrogen incorporates rigorous quality control measures. This ensures that the DNA ladders are reliable, reproducible, and suitable for scientific use.
- Research Advancements: Recent advancements in production techniques have improved accessibility and functionality, thereby broadening the applications in synthetic biology and genetic engineering.
Final thoughts on future research
Future research into supercoiled DNA holds significant promise. Expanding the understanding of its applications can benefit numerous fields, ranging from medicine to environmental science.
- Innovative Techniques: Researchers should explore innovative methodologies that enhance the production and application of supercoiled DNA ladders, potentially increasing their efficiency.
- Therapeutic Applications: Further investigation is warranted into the therapeutic applications of supercoiled DNA, particularly in gene therapy, where its stability can significantly impact delivery and efficacy.
- Interdisciplinary Studies: Collaborations across disciplines can yield novel insights, combining genetics, bioinformatics, and synthetic biology to harness the full potential of supercoiled DNA.