Exploring Tissue Layers: Structure and Function
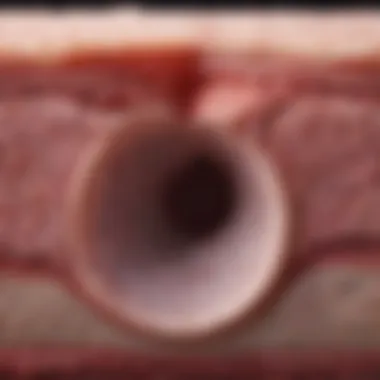
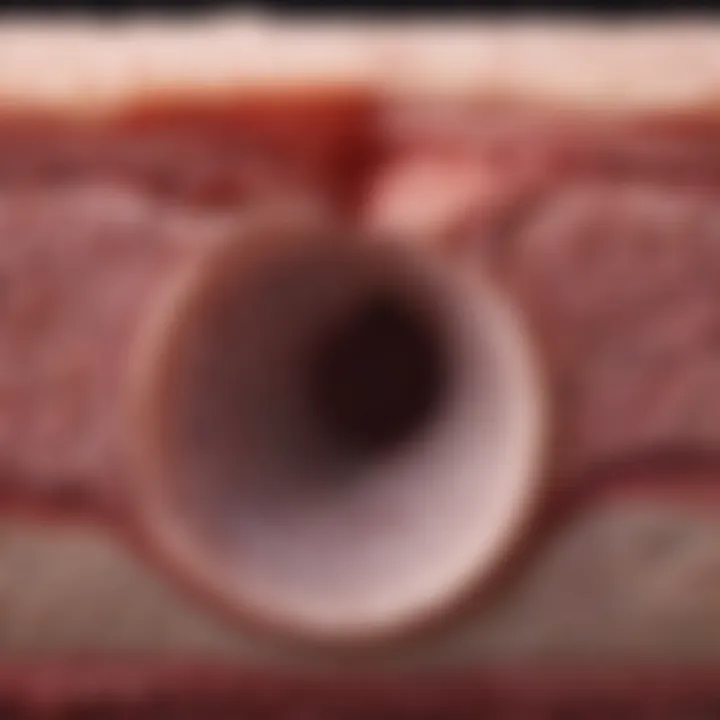
Intro
Understanding the complex architecture of biological organisms involves diving into the foundational elements that make up their structure: tissue layers. Each tissue type plays a fundamental role in the overall function of the organism. From the protective nature of epithelial tissues to the supportive framework of connective tissues, these layers not only contribute to the physical composure of the body but also enable crucial physiological processes. This exploration is essential not just for academic advancement, but also for its implications in health and disease management.
Research Highlights
Overview of Key Findings
In recent studies, researchers have revealed that tissue layers are not merely structures but also active participants in various physiological mechanisms. For instance, epithelial tissues, often viewed as protective barriers, have shown to play a significant role in nutrient absorption and secretion processes. Meanwhile, connective tissues are more than just supporting structures; they are involved in the regulation of immune responses and the wound healing process. Such insights not only enhance our understanding of these layers but position them as pivotal elements in therapeutic developments.
- Epithelial tissues facilitate absorption, secretion, and sensation.
- Connective tissues provide support and regulate various body functions.
- Muscle tissues enable movement and maintain posture.
- Nervous tissues are crucial for signal transmission and reflexes.
Significance of the Research
The implications of these findings extend into medical research and clinical applications. A deeper comprehension of tissue architecture allows researchers to develop targeted therapies, aiming to repair or regenerate damaged tissues. For example, understanding the regenerative capabilities of certain connective tissues can lead to breakthroughs in treating injuries or degenerative diseases. Moreover, such research contributes to advancements in tissue engineering and regenerative medicine, ultimately improving patient outcomes.
"Tissues are not just static entities; they are dynamic and interact intricately with each other to maintain homeostasis throughout the body."
Original Research Articles
Summary of the Article
The articles stemming from this body of research emphasize the multifaceted roles of tissue layers. They detail how variations in tissue structure correlate with specific functions and how disruptions in these tissues can lead to pathological states. Through detailed investigations, the articles underline the necessity of a comprehensive approach to studying tissues, one that considers both their individual contributions and their collective interaction within the organism.
Author Contributions
The collaborative efforts of various researchers have been instrumental in advancing the understanding of tissue layers. Each author brings a unique expertise, whether it's in histopathology, molecular biology, or regenerative medicine. Their collective work highlights the importance of interdisciplinary approaches in unraveling the complexities of tissue functions. The contributions span across laboratory research, clinical trials, and theoretical models, underscoring how multifaceted research can address real-world problems in tissue health.
This narrative on tissue layers illuminates important aspects of biology, fostering a greater understanding of how these fundamental components drive both health and disease. In the following sections, we will further examine the distinct types of tissues, their specific layers, and the interplay that underlies their biological significance.
Prelims to Tissue Layers
Understanding tissue layers is crucial to grasp how biological organisms function at a microscopic level. Tissue layers serve as the very fabric of life, combining cells with specialized functions to maintain health, facilitate communication, and protect various systems in our bodies. Without an appreciation for these layers, one would miss vital insights into both the normal functioning and pathology of organisms.
Definition and Importance
Tissue layers are defined as groups of similar cells that perform a specific function. These structures can be as thin as a paper sheet or as thick as a muscle wall, depending on their location and purpose. Each type of tissue layer—epithelial, connective, muscle, and nervous—has a unique composition and function that contributes to the functionality of organs and systems.
These layers are not merely passive structures; they play active roles in maintaining homeostasis, facilitating the transport of nutrients, and protecting against environmental threats. For instance, epithelial tissue acts as a protective barrier while also allowing selective absorption of substances.
Recognizing the importance of these layers is fundamental in medicine and biology as it shapes our understanding of disease mechanisms and informs therapeutic strategies.
Historical Overview
Historically, the study of tissue layers can be traced back to ancient times. Early philosophers and researchers, like Hippocrates and Galen, laid the groundwork by observing the human form and its functions. However, it wasn't until the advent of microscopy in the 17th century that the fine details of these layers were brought to light. Scientists such as Robert Hooke and Antonie van Leeuwenhoek made significant contributions by drawing and describing what they observed under their microscopes.
As the field advanced through the centuries, key figures like Rudolf Virchow asserted the concept of cellular pathology, connecting tissue structure to disease. The 19th century saw the development of histology as a distinct discipline, facilitating deeper insights into how tissue layers relate to health and disease processes. In the last few decades, technological advancements have dramatically accelerated our ability to study these layers, allowing for greater precision and understanding that has wide-ranging implications in regenerative medicine and cancer research.
In summary, the exploration of tissue layers opens up a world rich with information regarding living organisms. It not only highlights how these layers work together to maintain life but also underscores the historical evolution of biological sciences, showing how far we've come and the journeys yet to be taken.
Types of Tissue Layers
The exploration of tissue layers goes beyond mere biological definitions; it opens the door to understanding how living organisms function and maintain their integrity. Each type of tissue is characterized by unique structures and functions that contribute to the overall health and vitality of an organism. This section dissects the various types of tissue layers, shedding light on their importance within anatomical and physiological contexts.
Epithelial Tissue Layers
Structure and Function
Epithelial tissue layers serve as the first line of defense for the body, acting like a protective wall against harmful pathogens and injury. This type of tissue is structured to be tightly packed, with little space between cell layers, ensuring maximum coverage. The main characteristic of the structure is its ability to regenerate quickly, which is crucial when dealing with wear and tear, especially in areas like the skin and lining of organs. The turnover rate of epithelial cells can be advantageous, but it also raises questions about the maintenance of cell function and stability over time.
For instance, the epidermis regenerates every few weeks, ensuring it remains intact and functional. However, such rapid turnover can lead to the accumulation of mutations over time, potentially increasing cancer risks. This dichotomy emphasizes the necessity of considering both the benefits and potential drawbacks of epithelial tissue.
Types of Epithelial Tissue
When discussing the types of epithelial tissue, it’s essential to recognize that they are categorized based on cell shape and arrangement. A common classification includes simple squamous, cuboidal, and columnar epithelium. Simple squamous epithelium, for instance, is known for its flat, thin shape, allowing efficient gas exchange in the lungs. In contrast, columnar epithelium is found in the digestive tract, where it provides both protection and enhanced absorption.
The primary characteristic of these epithelial types is their specialization for various functions—some are designed for absorption, while others focus on secretion or protection. While the versatility of epithelial tissues is often beneficial, it’s not without its limitations, notably in injury response and susceptibility to infections.
Role in Absorption and Protection
The role of epithelial tissue layers in absorption and protection cannot be overstated. These layers not only guard underlying structures from injury but also play critical roles in absorbing necessary nutrients and signaling molecules from the external environment. For example, the epithelial lining of the intestines is specifically structured to maximize surface area through microvilli, enhancing its ability to absorb nutrients.
However, a unique challenge arises as these layers must balance protective measures with permeability. A disruption, for instance, in the epithelial gut barrier can lead to conditions like leaky gut syndrome, where harmful substances seep into systemic circulation. Thus, while epithelial tissue is fundamentally designed for protection and absorption, its integrity is paramount to overall health.
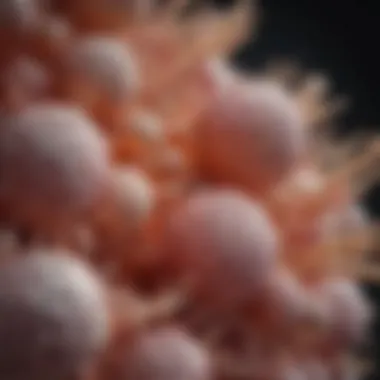
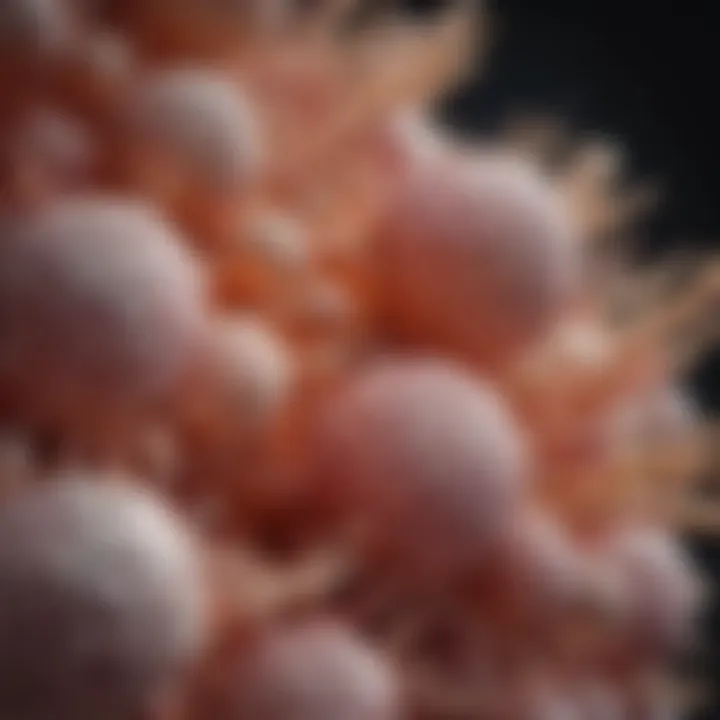
Connective Tissue Layers
Composition and Types
Connective tissue layers are fundamental to the structural framework of the body. Their diverse composition consists of various types of cells and fibers, embedded in an extracellular matrix, which provides support and integrity. Understanding the types—such as loose connective tissue, dense connective tissue, cartilage, and bone—will give further insight into their varying contributions to the body's mechanics.
The key characteristic of connective tissue is its ability to bind other tissues and organs together, providing both physical support and nourishment. While connective tissue very often plays a supportive role, it can also engage in complex processes, such as fat storage and immunity. However, the vast diversity in its composition can also lead to complications, like systemic disorders, which raise concerns over stability and function.
Extracellular Matrix
The extracellular matrix is more than just a supporting structure; it’s a complex network that facilitates communication between cells, dictates tissue architecture, and regulates processes such as tissue repair and growth. A particularly fascinating feature of the matrix is its adaptability; it can change in response to the physiological conditions, thereby impacting the function of associated tissues.
Its composition varies widely across different types of connective tissue, which can be both an advantage and a disadvantage. While adaptability offers resilience to changing conditions, it can also create vulnerabilities, such as fibrosis, when abnormal matrix deposition occurs during healing processes.
Function in Support and Transport
The primary function of connective tissue layers is to provide structural support and facilitate the transport of substances throughout the body. This is evident in cartilage, which provides a flexible yet strong support system, as well as in blood, which is a unique form of connective tissue essential for nutrient and waste transport.
One critical characteristic of this tissue layer is its vascularity. While some connective tissues are richly supplied with blood, others like cartilage are avascular, making healing processes much slower and susceptible. Recognizing these differences is crucial to comprehending how connective tissues respond to damage and repair over time.
Muscle Tissue Layers
Skeletal Muscle
Skeletal muscle tissue, which attaches to bones and facilitates movement, is characterized by its striated appearance and voluntary control. Each skeletal muscle fiber operates individually but cohesively with others to generate force; this integrated approach enables complex movements necessary for daily activities. A prominent advantage is its capacity for rapid contractions.
However, excessive strain or injury can lead to muscle tears, which underscore the need for adequate rest and recovery. Moreover, understanding the correlation between muscle fiber types—slow-twitch vs. fast-twitch—can greatly affect training and rehabilitation practices.
Cardiac Muscle
Cardiac muscle primarily makes up the heart; it combines properties of both skeletal and smooth muscles, having both striations and involuntary control. This unique arrangement allows for synchronized contractions essential for effective blood circulation.
The primary characteristic of cardiac muscle is its enduring nature, as it withstands prolonged use without fatigue. The downside, though, is its limited ability to regenerate; damage to cardiac tissue from conditions like heart attacks may lead to permanent impairment and lifelong complications.
Smooth Muscle
Smooth muscle is identified by its non-striated appearance and involuntary control. It resides in various hollow organs, such as the intestines and blood vessels, and modulates functions like digestion and blood pressure. Its unique capability to sustain prolonged contractions can be advantageous in functions such as peristalsis.
Still, its involuntary nature, although beneficial for many functions, also means that unconscious signaling is primarily responsible for regulation, which can sometimes result in dysregulation and lead to disorders such as hypertension.
Nervous Tissue Layers
Structure of Neurons
Neurons are the basic functional units of nervous tissue, responsible for transmitting impulses throughout the body. The structure comprises several components, including the cell body, dendrites, and axon. Neurons can vary in shape and size but their essential function remains the same: communication. This structural complexity allows for the integration of information across numerous pathways.
The key characteristic of neurons is their ability to transmit signals over long distances, thus facilitating rapid communication. However, a significant disadvantage is their vulnerability to injury. Damage to neurons is often irreversible, leading to lasting deficits in function.
Glial Cells
Glial cells, often overshadowed by neurons, play essential supportive roles in the nervous system. They assist in maintaining the microenvironment around neurons, providing insulation, nourishment, and protection. A defining feature of glial cells is their ability to proliferate and fill gaps left by injured neurons, a process essential for recovery.
While glial cells contribute greatly to overall health, their over-proliferation can sometimes lead to issues like gliosis, which can adversely affect neuronal function and health.
Role in Communication
The role of nervous tissue in communication is fundamental to the function of the entire body. It enables both voluntary movements and involuntary responses to stimuli. Neurons utilize neurotransmitters to create a dialogue across synapses, allowing for complex behaviors and reactions.
The beauty of this communication is that it is both quick and widespread; however, disruptions, such as neurotransmitter imbalances or damage to pathways, can lead to extensive complications, affecting everything from muscle control to mood. Evaluating the fine balance of signaling within this tissue layer can provide insight into numerous neurological disorders.
Layer Interaction and Communication
Layer interaction and communication forms a bedrock for understanding how tissues function cohesively in the human body. These processes are pivotal, influencing not only the health and functionality of tissues but also the body as a whole. Investigating these interactions sheds light on how different tissues coordinate their roles, offering insight into how organisms maintain homeostasis in the face of various challenges. The intricate dance of signaling pathways and interdependent tissue functions allows the body to respond adeptly to internal and external demands.
Interdependent Functions of Tissues
Tissues don't work in isolation; instead, they thrive on their interdependence, which is often a matter of necessity. For example, consider how epithelial tissues line organs and provide a barrier while simultaneously facilitating communication with the underlying connective tissues. Here’s what you need to know about interdependence:
- Support Systems: Connective tissues provide the structural framework for organs, integrating with epithelial layers to create functional units. The presence of blood vessels within connective tissue not only sustains epithelial cells but also facilitates nutrient exchange.
- Shared Signals: Tissues communicate via biochemical signals that necessitate cooperation. Muscle fibers contract based on inputs derived from nerve tissues; one cannot function effectively without the other. In fact, nerve tissues relay signals that stimulate muscle contraction—demonstrating a dependency that is both essential and complex.
- Holistic Response: During injury or disease, various tissues react in a chain response. For instance, if epithelial tissue is damaged, inflammatory signals emanate from the affected area, prompting immune responses that involve the connective and nervous tissues. This interdependency ensures a swift reaction to preserve health.
Understanding these interdependent functions allows researchers and medical professionals to evaluate treatment strategies effectively. Tailoring interventions can leverage the relationships between tissues to optimize outcomes.
Signaling Pathways Among Layers
The body communicates through an exciting array of signaling pathways connecting different tissue layers. These pathways are crucial for orchestrating functions, growth, and healing. The signaling mechanisms can be complex but are foundational to cascading reactions:
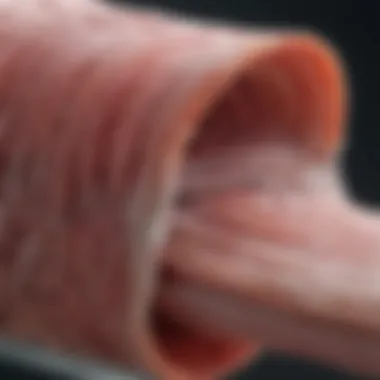
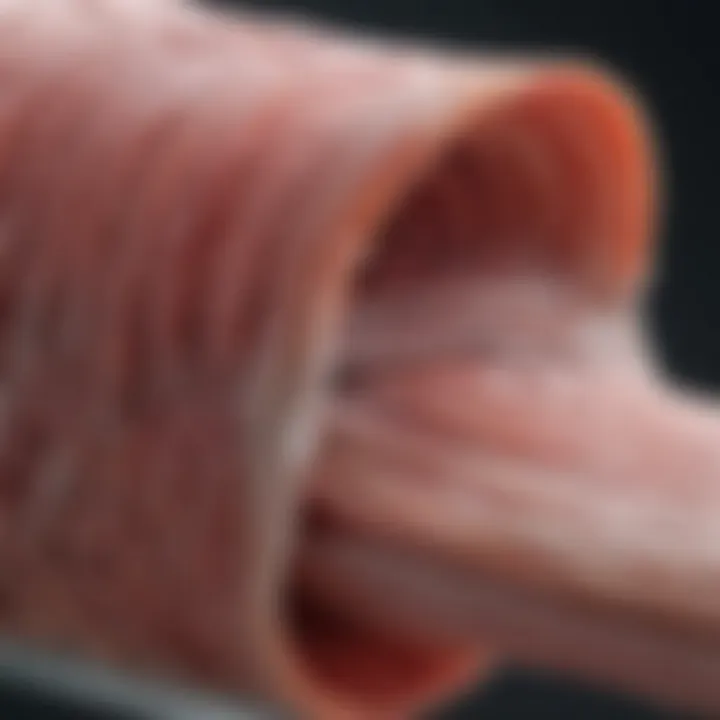
- Chemical Signaling: This involves molecules such as hormones and growth factors that jumpstart communication between tissues. For instance, signaling molecules released from injured tissues can alert neighboring cells, prompting repair processes.
- Mechanical Signaling: Tissues also transmit information through physical forces. Stretching or compressing tissues can initiate biochemical responses, which influences cellular functions and adaptation. Think of how muscles adapt in response to exercise through these mechanical forces.
- Electrical Signaling: Neurons and muscle tissues leverage electrical impulses in communication. In the heart, for instance, a group of specialized cells generates electrical signals that promote coordinated contractions, essential for effective pumping.
"The elegance of layered communication among tissues illustrates the body’s design for interaction—each layer informs and shapes the performance of another."
Understanding these signaling pathways has far-reaching implications for scientific research. Investigations into how these systems function—while identifying potential breakdowns or diseases—can lead to innovative therapies that restore proper communication between layers.
By grasping the nuances of tissue interactions, students and professionals alike can appreciate their vital role in anatomy and physiology. Exploring how these layers not only connect but also influence one another opens avenues for enhanced therapeutic strategies and deeper insights into biological processes.
Tissue Development and Regeneration
Tissue development and regeneration hold profound significance in the study of biological systems. These processes are not just fundamental to the growth and maintenance of organisms; they play a crucial role in understanding how tissues respond to injury and adapt over time. As we take a closer look at the mechanisms of embryonic development and the exciting prospects in regenerative medicine, it becomes clear that knowledge in these areas can lead to groundbreaking treatments for various diseases and injuries.
Embryonic Development of Tissues
The initial formation of tissues begins during embryonic development, a finely tuned process marked by cell differentiation and tissue organization. At this stage, stem cells, which have the unique ability to develop into different cell types, form the building blocks for all the various tissues in the body.
- Gastrulation - This pivotal phase tailors what the future body plan will look like and results in the formation of three germ layers: ectoderm, mesoderm, and endoderm. These layers will give rise to all the organs and tissues in the body.
- Neurulation - In this stage, the neural tube forms, setting the stage for the development of the nervous system, a critical component of tissue interaction.
- Organogenesis - As tissues further differentiate, organs begin to form. The coordination of signaling pathways is crucial here, guiding how cells know when and where to change into specific tissue types.
Embryonic development sheds light on how tissues interact and their fundamental roles in creating a functioning organism. By understanding these processes, researchers can better appreciate not only how tissues develop but also how they might be repaired or regenerated after injury or disease.
Regenerative Medicine Prospects
Regenerative medicine is where current scientific advancements meet the practical need to heal tissues and organs. This field aims to repair or replace damaged tissues, addressing the limitations of traditional therapy. Two promising avenues in this field include stem cell research and tissue engineering.
Stem Cell Research
Stem cell research is a cornerstone of regenerative medicine. What makes stem cells special are their abilities to self-renew and differentiate into various specialized cell types. This characteristic is key for potential treatments for conditions like spinal cord injuries, heart disease, and degenerative disorders. One advantage of using stem cells is their origin from various sources:
- Embryonic Stem Cells - These provide the greatest differentiation potential but come with ethical considerations.
- Adult Stem Cells - Found in tissues like bone marrow, they can regenerate specific cell types but have limited capacity compared to embryonic cells.
However, there are challenges, such as the risk of tumor formation and the need for precise control over differentiation processes. Researchers are actively exploring why these cells sometimes go awry in the hope of mitigating these risks.
Tissue Engineering
Tissue engineering takes a slightly different approach by creating scaffolds designed to support the growth of new tissues. The key characteristic of tissue engineering lies in its combination of biomaterials and living cells. A significant benefit of this approach is its ability to model specific tissue types, offering the potential to produce replacement tissues that can integrate with the body.
Unique features of tissue engineering often include:
- 3D Bioprinting - This technology allows for the precision assembly of cells and scaffolds, creating complex tissue structures that mimic real tissue.
- Biocompatibility - Materials used must be compatible with human tissues to promote integration and avoid rejection.
Despite these advances, tissue engineering faces hurdles, including achieving adequate vascularization in fabricated tissues to ensure they survive once implanted in the body.
"The development and regeneration of tissues are not just scientific inquiries but potential lifelines to those who suffer from devastating injuries and diseases."
In summary, the exploration of tissue development and regeneration opens the door to innovative medical solutions. As research progresses in stem cell applications and tissue engineering, the promise of effectively repairing and enhancing human health becomes ever more tangible.
Pathological Changes in Tissue Layers
Understanding pathological changes in tissue layers is paramount in the context of biological research and medicine. These changes provide critical insights into how tissues respond to various insults, whether they originate from physical trauma, infectious agents, or underlying genetic disorders. By dissecting these alterations, researchers can lay the groundwork for treatment protocols and therapeutic interventions. Hence, the complexity of tissue pathology is not merely an academic concern but a practical challenge that holds the potential to save countless lives.
Tissue Injury Mechanisms
When delving into the mechanisms of tissue injury, several factors must be taken into account. From the cellular standpoint, injuries can be classified into two broad categories: acute and chronic. Acute injuries often occur suddenly, commonly due to external trauma, like a cut or a fracture, which provoke immediate responses from the body. In contrast, chronic injuries develop gradually over time, often as a result of long-standing irritation or repetitive stress, leading to gradually worsening conditions.
Key mechanisms include:
- Hypoxia: A condition where tissues receive insufficient oxygen, often crippling cellular functionalities.
- Inflammation: A natural healing response can become detrimental if it spirals out of control, damaging nearby healthy tissue.
- Apoptosis: Programmed cell death, a mechanism that can become dysregulated in many diseases, resulting in the premature loss of functional cells.
Each of these mechanisms highlights the dynamic ways in which tissues react—sometimes effectively; other times, leading to greater complications.
Diseases Affecting Tissue Layers
Cancer
Cancer exemplifies a critical area for exploration when examining pathological changes. Primarily, it’s the unregulated growth of abnormal cells that makes this disease particularly concerning. At the tissue layer level, cancer displays a unique characteristic: the ability to invade adjacent tissues and metastasize. This makes understanding cancerous tissue not just a matter of identifying tumors but of studying how they disrupt normal tissue architecture.
The complexity of cancer contributes extensively to our understanding of tissue dynamics, providing a rich tapestry of interactions and alterations—essential for developing effective treatment plans. However, one must be cautious: the heterogeneous nature of tumors often leads to a variability in treatment response, making it a challenging subject.
Inflammatory Diseases
Inflammatory diseases further complicate the narrative of tissue pathology. At a fundamental level, these diseases turn the body’s defense mechanisms against itself. Rheumatoid arthritis, for instance, is a prime example where the inflammatory response affects joint tissues, resulting in pain and degradation.
One of the key characteristics of inflammatory diseases lies in their chronic nature, which can lead to persistent tissue changes such as hypertrophy, fibrosis, and degenerative alterations. Importantly, understanding these diseases equips scientists and medical practitioners with the tools to formulate intervention strategies that might alleviate symptoms or halt progression, but the risks of long-term medication effect remain a precarious balance.
Degenerative Disorders
Degenerative disorders highlight another significant aspect of disease processes affecting tissue layers. These disorders often involve the gradual degeneration of tissues, with conditions like Alzheimer's disease providing a striking example. A major characteristic here is the progressive loss of function. In this context, the brain's tissue layers undergo significant changes, affecting cognitive functions devastatingly over time.
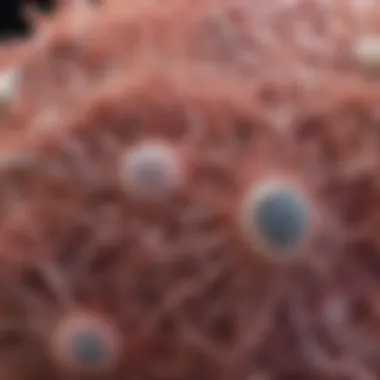
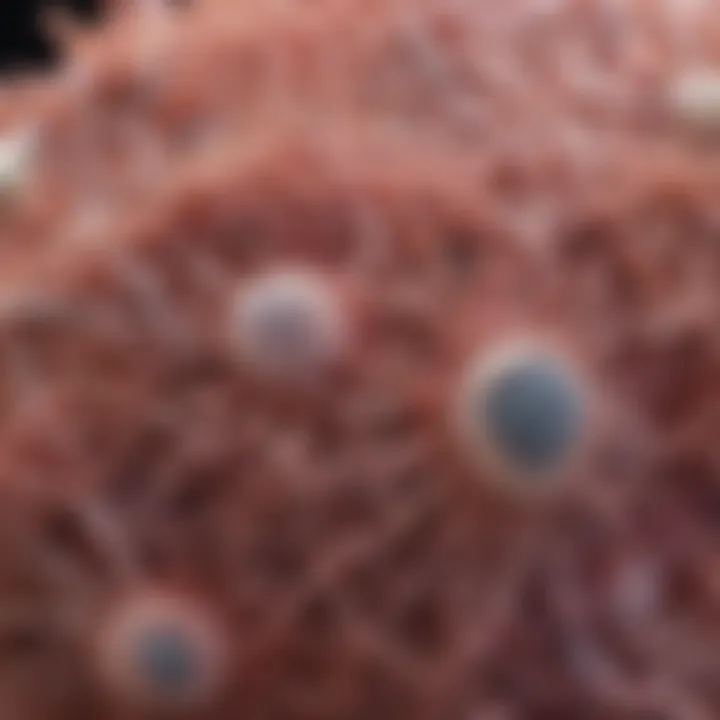
A unique feature of degenerative disorders is the reorganization of tissue structures that often leads to a lack of effective communication both locally, within the affected region, and systemically, across different bodily functions. While understanding degenerative processes is vital for developing care strategies, the challenge lies in their often irreversible nature, posing deep implications for public health and resource allocation.
Pathological changes in tissue layers reflect a myriad of responses and adaptations, revealing the intricate dance between normal physiology and disease. Studying these phenomena opens doors to innovation in therapies and preventive measures across various medical fields.
Technological Advances in Tissue Study
In today's fast-paced world of biomedical research, the study of tissue layers has greatly benefited from technological advancements. These innovations have fundamentally altered how we analyze, visualize, and understand tissues on a microscopic level. They not only enhance our understanding of tissue architecture but also pave the way for significant breakthroughs in personalized medicine and innovative treatments.
Imaging Techniques
Histology
Histology serves as the cornerstone of tissue analysis. By examining thin slices of tissue under a microscope, researchers can discern detailed structures and identify pathological changes. One of the key characteristics of histology is its ability to reveal intricate cell organization. This makes it a preferred method in both research and clinical settings. Histology allows for the visualization of normal and abnormal tissue, making it invaluable in diagnosing diseases.
However, histology does have its quirks. The need for tissue samples means that the process can be invasive, and sample preparation can alter the native state of the tissue. Still, its benefits often outweigh these drawbacks, particularly when coupled with modern staining techniques that enhance the visibility of various cellular components.
Microscopy
When it comes to visualizing tissues at an even finer scale, microscopy takes center stage. This technology enables scientists to observe structures that are simply beyond the reach of the naked eye. Advanced forms of microscopy, such as confocal or electron microscopy, provide high-resolution images that allow researchers to delve deeper into tissue layers. The significant advantage of microscopy is precision; it can reveal minute details, such as cellular substructures or interactions among different cell types.
On the flip side, microscopy can be resource-heavy, requiring expensive equipment and specialized training to interpret the results. Yet, its role in understanding tissue complexity cannot be overstated.
Molecular Techniques
Genomics
As research progresses, genomics has taken the lead in exploring the genetic basis of tissue-specific behaviors and responses. Genomics focuses on the entirety of an organism's genes and their functions, which has made it increasingly relevant in the study of tissue layers. Its primary characteristic is its ability to identify gene expression patterns in various tissue types. This information is crucial for understanding diseases that have a genetic component.
Genomics is popular among researchers due to its potential to unveil pathways involved in tissue development and disease. Nonetheless, like many technologies, it suffers from complexities in data interpretation and may require extensive computational resources.
Proteomics
Proteomics complements genomics by analyzing the entire set of proteins expressed in a tissue. This field focuses on understanding the functional aspects of proteins and their interactions within the tissue environment. By providing insights into how proteins behave within different tissue contexts, proteomics enhances our grasp of biological processes such as signaling, repair, and disease progression.
Similar to genomics, proteomics is celebrated for its comprehensive approach, revealing the dynamic nature of proteins. Yet, it demands meticulous experimental design and data analysis, presenting its own set of challenges.
"The advancement in technological tools is not just an evolution; it’s a revolution, changing the dynamics of tissue study at a fundamental level.”
By capitalizing on these technological innovations, researchers can derive deeper insights into tissue layers, which ultimately contributes to advancements in medical science and patient care. As we move forward, these tools will only grow in relevance, shaping the future landscape of tissue research.
Future Directions in Tissue Research
The universe of tissue research is at a precipice of thrilling advancements that promise to revolutionize healthcare and scientific understanding. As we voyage further into the intricacies of biological tissues, the horizons of personalized medicine and innovative tissue engineering are emerging as frontiers of exploration. Each step taken in these directions holds invaluable potential for improving treatment outcomes and optimizing regenerative techniques. The necessity of delving into future tissue research is underscored by several factors that merit attention.
Towards Personalized Medicine
Personalized medicine is not merely a catchphrase; it’s an approach that tailors medical treatment to individual characteristics of each patient. The path towards achieving this goal relies heavily on understanding tissue layers at a molecular level. By deploying techniques such as genomics and proteomics, researchers aim to identify unique tissue markers. Such markers can guide bespoke treatment plans, enhancing efficacy while minimizing adverse effects.
- This tailoring will mean not just customized drug dosages but also the potential for engineered tissues that suit a patient’s specific biome.
- The influence of genetic makeup on tissue response to treatments is another critical area of study, shedding light on how different bodies can react to the same intervention in disparate ways.
- For instance, in cancer treatment, recognizing the differential response of tumor tissues can lead to optimized therapies that target cancer cells specifically while sparing healthy tissue.
Beyond individualization, one must consider the ethical implications as well. Personal genetic data brings questions about privacy and consent that researchers must navigate carefully. Mapping a patient’s tissue characteristics is a double-edged sword; while it can lead to breakthroughs, maintaining the integrity and privacy of patient information is paramount.
Exploring Tissue Engineering Applications
The realm of tissue engineering is where creativity meets scientific rigor. By mimicking natural tissue structures, scientists and engineers are not just patching wounds but are also creating entirely new tissues to replace those lost due to disease or injury. The convergence of bioprinting techniques, scaffold technology, and stem cell research has opened up avenues previously deemed unattainable.
For example,
- Three-dimensional bioprinting has gained traction, producing tissues that can function effectively in the human body.
- Advances in scaffold design materials enhance cell attachment and proliferation, encouraging healthy tissue growth and stability.
Moreover, the harnessing of stem cells is shedding light on alternative healing pathways.
- Researchers are actively exploring how stem cell-derived tissues can recreate complex organ structures, potentially paving the way for organ transplants without the concern of donor compatibility issues.
- Furthermore, combining tissue engineering with regenerative medicine raises tantalizing prospects—a future where damaged organs are not just repaired but replaced with lab-grown counterparts.
"Innovations in tissue engineering not only aim to repair but to replace. This advancement could significantly reduce the reliance on organ donors and the accompanying ethical dilemmas."
The scope of tissue engineering extends into combating age-related degenerative disorders. Knowledge of tissue compositions can guide interventions that promote healing and regeneration. In summary, as we step into the future of tissue research, it’s clear that the fusion of biology with technology will reshape our understanding of health and disease management. The implications for personalized medicine and tissue engineering applications promise a new era in treating some of humanity's most persistent medical challenges.
Finale
The conclusion serves as a vital recap that ties together the threads of our exploration into tissue layers. It’s essential because it distills the myriad of points discussed throughout the article into digestible bites. Each type of tissue—epithelial, connective, muscle, and nervous—contributes to the overall framework not just of anatomy but also of functionality within biological organisms. By synthesizing complex information, the conclusion aids readers in grasping the significance of these layers in everyday health and in moments of pathology.
Summary of Key Points
- Diverse Functions: Each tissue type performs critical roles, from protective barriers in epithelial tissue to the supportive roles played by connective tissues.
- Layer Interactions: The interdependence of various tissue layers creates a well-oiled machine that maintains the body's health and normal functioning.
- Development and Repair: The information on how tissues develop and heal provides insights into regenerative medicine, a booming field that fosters advancements in health care.
- Technological Impact: With emerging imaging and molecular techniques, researchers are better equipped to understand and manipulate tissue layers, enhancing both study and applications.
- Future Directions: As we step into an era more focused on personalized medicine, understanding these basics can guide future research and innovations.
Implications for Future Research
The insights gleaned from the examination of tissue layers have profound implications for the direction of future research. The detailing of cellular structures opens doors for further studies into various diseases affecting tissue integrity. For example:
- Cancer: As we uncovered, altered tissue layers can lead to malignancy. Future research can aim at targeting these transformations at the cellular level.
- Regenerative Therapies: Delving deeper into stem cell research can accelerate breakthroughs in healing damaged tissues, leading toward sustainable solutions for many chronic conditions.
- Personalized Medicine: The embrace of individual variations in tissue architecture can refine treatment methods, tailoring them to specific patient needs. This could revolutionize how healthcare providers approach diseases like cardiovascular disorders and diabetes.