Inducible Plasmids: Mechanisms and Applications
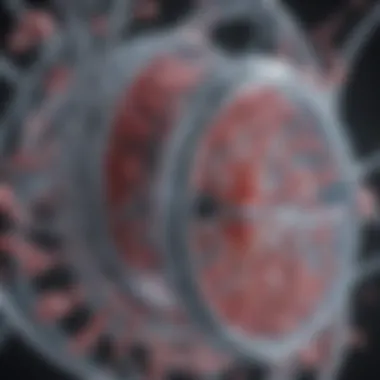
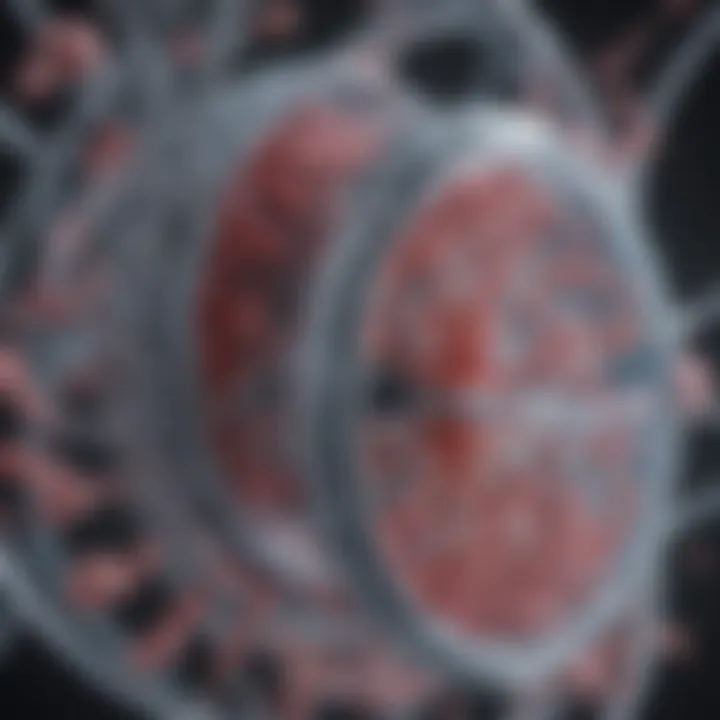
Intro
Inducible plasmids have transformed the landscape of genetic engineering, providing researchers with powerful tools to manipulate gene expression in a controlled manner. Understanding how these plasmids operate not only sheds light on their utility but also lays the groundwork for future advancements in biotechnology. This article aims to take a deep dive into the essential characteristics, operational mechanisms, and diverse applications of inducible plasmids, while evaluating both ongoing research and potential future developments.
Research Highlights
Inducible plasmids stand at the cutting edge of molecular biology. They harness specific environmental cues to activate and deactivate gene expression. This leads to flexible experimental design across varied scientific fields, from synthetic biology to drug development.
- Overview of Key Findings
- Recent studies have illuminated novel ways to enhance the stability and efficiency of these plasmids, making them even more effective for practical applications. Scholars have noted improvements in the design of regulatory elements that can fine-tune geneexpression levels, paving the way for greater applications.
- Significance of the Research
- Inducible plasmids can be constructed to respond to a wide array of inducers, including chemical agents, temperature shifts, and even light. This responsiveness enables the precise control of gene activity, facilitating experiments that mimic physiological processes.
- The research surrounding inducible plasmids is paramount as it contributes to understanding genetic regulation. In a world where gene therapy and synthetic biology hold significant promise, this work may lead to groundbreaking strategies for treating genetic disorders and optimizing microbial processes.
"The advances in inducible plasmid technology present options that were not previously available, expanding capabilities beyond traditional genetic modification."
Original Research Articles
Exploring original research articles provides a foundation for comprehending the practical applications of inducible plasmids.
- Summary of the Article
- Author Contributions
- The insights drawn from various research publications reveal compelling results regarding the flexibility and application of inducible plasmids in different organisms. For instance, studies on Escherichia coli and Saccharomyces cerevisiae have illustrated genes that can be turned on or off based on specific stimuli, showcasing manipulation possibilities that stretch far and wide.
- Authors in these studies have contributed meticulously, often collaborating across disciplines to assemble findings that advance both theoretical knowledge and practical applications in biotechnology. Their interdisciplinary efforts spotlight the importance of collaboration in modern science.
Understanding inducible plasmids involves more than just grasping their mechanics; it requires appreciation for the pivotal role they play in the future of genetic research and application. With steadfast advancements and ongoing challenges in plasmid design and function, the road ahead is replete with potential.
Preamble to Inducible Plasmids
In the bustling realm of genetic engineering, inducible plasmids stand out as crucial tools. They are not just mere conduits for genetic material; rather, they are sophisticated mechanisms designed for controlled gene expression in a variety of organisms. This section highlights the significance of these plasmids by covering their definition, fundamental concepts, and historical evolution. Understanding these aspects is imperative as we embark on exploring how inducible plasmids operate, their diverse applications, and their potential futures.
Definition and Fundamental Concepts
Inducible plasmids are specialized vectors capable of amplifying or silencing gene expression in response to specific signals. At their core, these plasmids possess unique regulatory elements that allow for the manipulation of gene activity. The key features often include an origin of replication, selectable markers, and, most importantly, promoters that can be turned on or off based on certain conditions. For instance, the lac operon is a quintessential example that responds to lactose and regulates the genes involved in its metabolism. This versatility in gene regulation plays a pivotal role in both research and practical applications, from protein production to study of gene function.
Such systems permit researchers to observe the effects of gene expression dynamics without the chaos of continuously active genes. By selectively inducing a gene's activity, scientists can maintain tighter control over experimental conditions, thus leading to more accurate results.
Historical Development
The journey of inducible plasmids has been nothing short of revolutionary within the field of molecular biology. The concept first began to take shape in the 1960s when researchers were deciphering the underlying principles of gene regulation. A major breakthrough came with the discovery of the lac operon in E. coli, which laid the groundwork for understanding how genes could be turned on or off in response to environmental cues. This early groundwork set the stage for the development of plasmids with inducible features.
As the decades rolled by, advancements in techniques like cloning, sequencing, and synthetic biology catalyzed new developments. By the late 20th century, synthetic promoters began entering the scene, allowing for fine-tuning of gene expression. This progression has continued, with researchers now designing highly specific induction systems tailored for particular needs, like the use of Tetracycline-controlled expression systems.
Today, the efficiency and efficacy of inducible plasmids have expanded their utility beyond academic labs to industrial and agricultural fields, demonstrating their enormous potential.
"Inducible plasmids not only serve research but have become vital players in industrial processes, transforming biotechnology."
Structural Components of Inducible Plasmids
Understanding the structural components of inducible plasmids is crucial in the realm of genetic engineering. These elements are not merely parts that go together; they are foundational to the plasmids’ functionality and versatility in various applications. The interplay between different components influences the efficiency of gene expression and the overall effectiveness of the plasmid in research and industrial environments. Each segment can define how well a plasmid performs its targeted role, whether it's producing a valuable protein or enabling controlled gene editing techniques.
Key Elements
Origin of replication
The origin of replication is a critical feature of inducible plasmids. It refers to the specific sequence at which DNA replication begins, allowing the plasmid to be replicated within a host cell. An important characteristic of these origins is their compatibility with the host organism's machinery, ensuring seamless integration and replication.
For example, the pUC origin is popular among researchers because it provides a high copy number, leading to an abundance of plasmid DNA, which is beneficial for experiments requiring large amounts of material. This high replication efficiency is not without drawbacks; if too many copies are made, it may impose a burden on the host cell, potentially affecting growth rates negatively. Therefore, while a strong origin fosters efficiency in obtaining plasmid copies, bioengineers must balance this against potential cellular stress.
Selectable markers
Selectable markers are essential for the identification and isolation of genetically modified cells. These markers typically confer resistance to antibiotics or enable the utilization of specific nutrients unavailable to non-transformed cells. A standout characteristic of selectable markers is their ability to provide a clear ‘yes or no’ answer regarding whether a cell has successfully incorporated the plasmid.
For instance, the use of the bla gene, which provides resistance to ampicillin, has been widely adopted. Its unique feature is that it enables researchers to grow only the transformed cells on media containing the antibiotic, effectively eliminating any non-modified counterparts. However, reliance on antibiotic selection can raise safety concerns and contribute to the development of resistance, necessitating careful consideration of the markers chosen.
Promoters
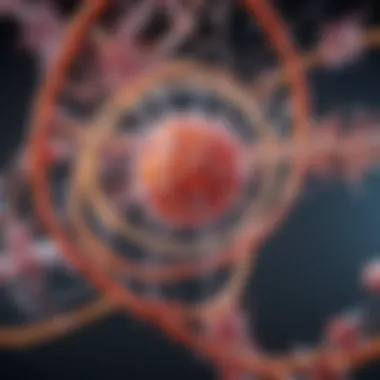
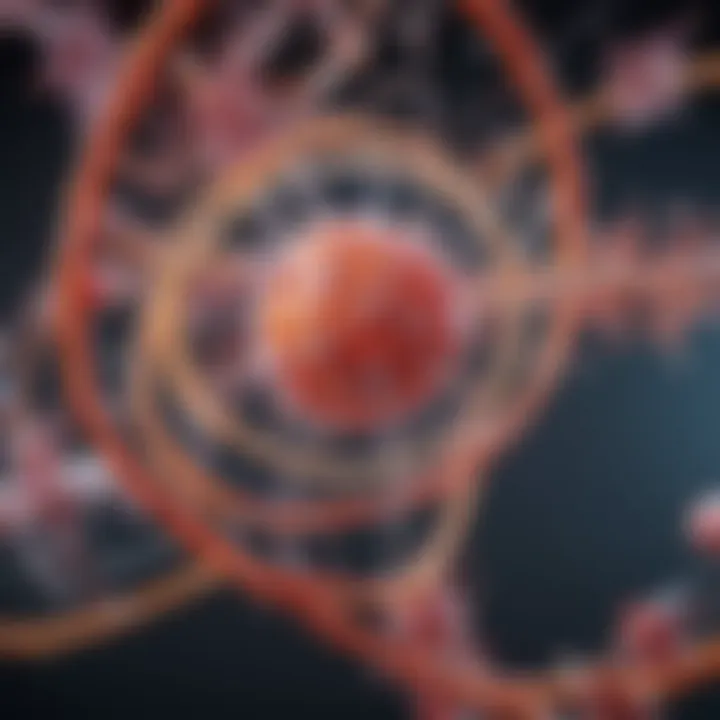
Promoters play a pivotal role in regulating gene expression within inducible plasmids. They are sequences that initiate the transcription of a particular gene, effectively acting as on/off switches. A significant characteristic of promoters in inducible plasmids is their ability to be controlled in response to various stimuli, such as the presence of a specific chemical inducer.
Take, for example, the lac promoter. This promoter is renowned for its tight control over gene expression due to its responsiveness to lactose and derivatives close to it. This unique feature enables researchers to finely tune gene expression levels, providing a refined control of protein production. However, the lac promoter can sometimes exhibit leaky expression, which may result in unintended protein synthesis even in the absence of inducers, complicating experiments when precision is needed.
Types of Inducible Promoters
Delving into the different classes of inducible promoters uncovers a diverse landscape of functionality parameters suited for various applications.
Tight regulation
Tight regulation refers to the precision in controlling gene expression, often facilitated by promoters that respond sharply to inducers. Such promoters are characterized by their ability to express genes only when the appropriate signals are present. The benefit of this strong regulation is the minimized background expression, leading to cleaner experimental results. Using these tightly controlled systems can be essential in sensitive applications, such as therapeutic protein production, where consistency is paramount. However, this high level of regulation can sometimes make it challenging to reach optimal expression levels in certain systems, which can frustrate scientists seeking higher yields.
Leaky expression
Leaky expression describes a scenario where a promoter allows a small amount of gene expression, even in the absence of induction. This can seem counterintuitive at first, but it holds specific advantages in applications where a baseline level of protein is beneficial. Such promoters are generally easier to manage, as they don’t require stringent conditions to express genes. However, the downside here is that they can complicate quantifying results accurately. Identifying expressed levels in the presence of background noise adds a layer of complexity that researchers must account for in their experimental designs.
Comparison of promoter systems
Comparing various promoter systems is paramount when one seeks to optimize plasmid functionality for specific tasks. Different promoters vary in their response to inducers, efficiency of transcription, and control over expression. A significant benefit of such comparisons lies in the ability to select the most suitable option based on the desired outcome. For instance, while the T7 promoter offers high levels of expression in the presence of its corresponding polymerase, another promoter might provide tighter regulation but lower overall yield. However, the trade-offs between high efficiency and precise control can be delicate balancing acts that researchers must navigate according to their experimental goals.
Mechanisms of Action
Understanding the mechanisms of action associated with inducible plasmids is essential for appreciating how these tools manipulate gene expression. At the heart of this knowledge lies the idea that the effectiveness of these plasmids often hinges on two fundamental aspects: how induction occurs and how gene regulation takes place. These processes don't merely enhance the utility of plasmids; they unlock their full potential in diverse applications ranging from research laboratories to industrial and agricultural settings.
Induction Mechanisms
Chemical inducers
Let’s first take a closer look at chemical inducers. These small molecules play a monumental role in initiating gene expression via inducible plasmids. One of the most recognized chemical inducers is isopropyl β-D-1-thiogalactopyranoside (IPTG), which is favored for its ability to activate the lac operon, a classic model for studying gene regulation. The key characteristic of chemical inducers is their specificity; they can be designed or selected to induce expression in a tightly controlled manner.
The unique feature of chemical inducers is that their effects can be finely tuned. This tuning ability offers scientists flexibility, allowing them to optimize conditions based on experimental needs. However, their use does come with drawbacks, notably the potential cost of some chemical inducers and the need for careful handling to avoid toxicity in host organisms.
Environmental factors
Next, we turn our attention to environmental factors which can also modulate gene expression. These factors include temperature, pH levels, and nutrient availability. For instance, some plasmids are responsive to temperature shifts, leading to activated gene expression when certain thresholds are crossed. The key characteristic of these environmental inducers is their natural interactions, making them appealing in contexts where engineered solutions might add unnecessary complexity.
The unique feature of environmental controls is their inherent ability to leverage the organism's natural biology, potentially reducing off-target effects and promoting biosafety. However, the reliability of environmental factors can be unpredictable, influenced by fluctuations that occur in real-world settings. Researchers must troubleshoot and mitigate these inherent variabilties in experimental designs.
Gene Regulation
Shifting gears, let’s delve into how gene regulation complements these induction mechanisms. This regulation provides the frameworks within which gene expression can be fine-tuned and controlled, leading to efficient utilization of resources in host organisms.
Transcriptional control
Transcriptional control is a cornerstone of gene regulation in inducible plasmids. By using various systems, transcription factors can enhance or inhibit transcription in response to specific signals. The core of transcriptional control lies in its versatility; it can be tailored to respond to various inducers, both chemical and environmental. This adaptability has made it a beneficial choice in many experimental setups.
The unique feature of transcriptional control is the precision it affords scientists. With multiple layers of control, researchers can achieve a spectrum of expression levels, from a trickle to a torrent, depending on the needs of their experimental design. The downside, however, is that misregulation can lead to unintended consequences, such as metabolic burden or resource allocation shifts within the host organism, which makes sequencing and validation crucial.
Post-transcriptional modifications
Finally, there’s the realm of post-transcriptional modifications. This aspect focuses on how gene expression is regulated after transcription has taken place. Such modifications can include RNA splicing, editing, and degradation rates, all of which significantly influence the stability and translation efficiency of mRNA molecules. These layers of control can be exceptionally beneficial, ensuring that only the desired proteins are synthesized when needed.
The unique feature of post-transcriptional regulation is its role in fine-tuning expression dynamics—alleviating potential bottlenecks that may affect protein synthesis. Nonetheless, the complexity of these mechanisms requires advanced knowledge and might introduce a new layer of potential misregulation, as changes in one area might disproportionately affect others.
When it comes to designing inducible plasmids, understanding both the induction mechanisms and gene regulation is the key to unlocking their true potential.
In summary, the mechanisms of action of inducible plasmids are multifaceted, intricately bound to the nuances of both induction strategies and regulatory systems. By mastering these concepts, researchers and professionals can significantly improve their strategies for utilizing inducible plasmids across various fields.
Applications of Inducible Plasmids
Inducible plasmids have carved their niche in the realm of biotechnology, showcasing a rich tapestry of applications across various fields. They deliver the ability to control gene expression, which in turn is vital for multiple scientific and industrial endeavors. From elucidating gene functions in research to propelling innovations in agricultural biotechnology, the versatility of these plasmids is undeniable. Each application not only highlights the advancements made but also throws light on the challenges faced by researchers and industry professionals alike.
In Research
Gene function analysis
Gene function analysis is a cornerstone of genetic research, enabling scientists to decode the roles of specific genes. By employing inducible plasmids, researchers can regulate the timing and extent of gene expression in a precise manner, a flexibility not typically offered by constitutive systems. This ability to manipulate genes can significantly enhance our understanding of complex biological processes, making this technique a popular choice in labs across the globe.
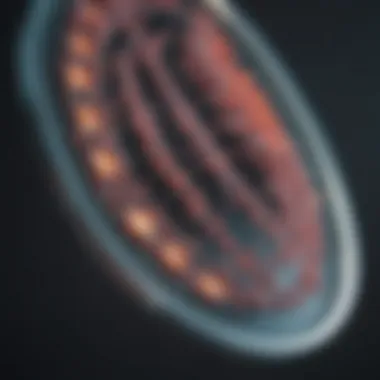
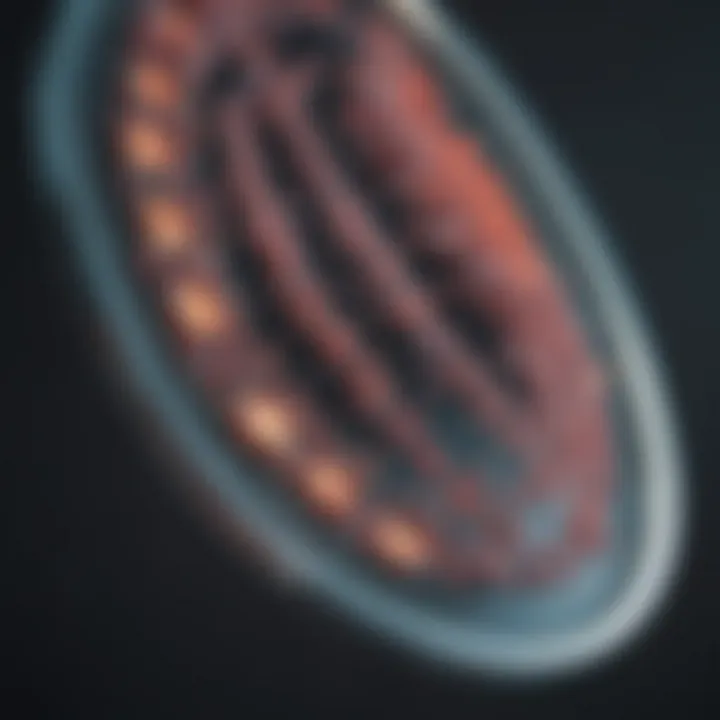
What sets gene function analysis apart is its capability to unravel interactions within cellular pathways. One unique feature is the ease with which researchers can switch gene expression on or off using various inducers such as IPTG or arabinose, depending on the promoted system utilized. However, it’s worth noting that leaky gene expression can sometimes pose challenges. This means researchers must carefully design their experiments to ensure that expression levels are appropriate for achieving reliable and reproducible results.
Protein expression studies
Protein expression studies are vital for producing recombinant proteins, which are crucial for a variety of applications including drug development, enzyme production, and vaccine synthesis. Inducible plasmids provide a more controlled environment where researchers can elicit high yields of the specific proteins needed. One of the main benefits is that these plasmids allow for an easy switch from low to high expression once needed, thereby mitigating unwanted metabolic drain on the host organism during the growth phase.
A key characteristic of protein expression studies using inducible plasmids is the ability to isolate expression conditions. For instance, researchers often use a strong, inducible promoter that is triggered under specific conditions. This feature ensures that proteins are produced in higher quantities only when desired. However, the down side can be that optimizing these systems for maximal yield can require significant time and resources, making it a daunting undertaking.
Industrial Biotechnology
Biopharmaceutical production
The production of biopharmaceuticals represents a leading edge of industrial biotechnology, with inducible plasmids playing a pivotal role. They are employed to express therapeutic proteins, antibodies, and enzymes necessary for treating various diseases. The beauty of using inducible plasmids in this context lies in the reduced risk of contamination and the ability to regulate production levels according to market demand.
A particular strength of using these plasmids in biopharmaceuticals is their high yields of functional proteins. The controlled expression enables manufacturers to ramp up production quickly, which is crucial in responding to health crises such as pandemics. However, scalability can present challenges; the process must ensure that large-scale production retains the effectiveness of the plasmid design used in laboratory settings.
Biofuel generation
In the quest for sustainable energy sources, biofuel generation has become a focal area. Inducible plasmids are now being investigated for their potential to enhance the efficiency of microorganisms used in producing biofuels from biomass. They allow for fine-tuning of metabolic pathways, maximizing the conversion of organic matter into energy resources.
One notable feature in this application is the ability to manipulate microbial strains for optimal performance. This can lead to improved yields of ethanol or biodiesel, making renewable energy more viable. While the promise is great, the disadvantages may include the cost and complexity of the systems required for effective operation, alongside regulatory hurdles that affect the deployment of engineered organisms in the field.
Agricultural Biotechnology
Genetically modified organisms
Genetically modified organisms (GMOs) have stirred considerable debate and interest. Inducible plasmids are crucial in designing GMOs with desired traits like pest resistance or enhanced nutritional value. The ability to precisely control when these traits are expressed opens new avenues in this field.
One key aspect is that researchers can engineer crops to express beneficial genes only under certain conditions, thus reducing unintended consequences and addressing some public concerns about GMOs. This targeted approach can be a beneficial characteristic, offering both ecological sustainability and economic advantages. Yet, on the flip side, the long-term implications of such techniques are still not fully understood, prompting the need for continued research and regulatory oversight.
Crop improvement techniques
Crop improvement techniques using inducible plasmids can significantly increase the resilience and productivity of crops. By allowing scientists to introduce traits that can be activated under specific conditions—such as drought or invasive pests—there’s potential for greater food security and reduced reliance on chemical pesticides.
The unique feature of utilizing inducible promoters in this field is that it can facilitate the expression of multiple genes simultaneously. This multi-gene expression can lead to more robust responses against environmental challenges. However, the disadvantages may include the potential for cross-contamination with non-GMO crops and the societal pushback that often accompanies these advances.
Innovations using inducible plasmids have the potential to transform not only research methodologies but also industrial and agricultural applications. There’s a continuous interplay between scientific possibility and ethical considerations that shapes the landscape of biotechnology.
In summary, the applications of inducible plasmids resonate profoundly within both research and industry, offering powerful tools for exploration and development. As these fields advance, ongoing innovation in the design and use of inducible systems will further amplify their impact.
Challenges in Designing Inducible Plasmids
Designing inducible plasmids is no walk in the park. While the potential applications of these genetic tools are vast, there are notable challenges that researchers and biotech professionals must navigate. Understanding these hurdles is critical, as successful plasmid design impacts everything from experimental outcomes to regulatory approvals in industrial applications. Addressing these challenges not only enhances the utility of inducible plasmids but also paves the way for innovation in genetic engineering.
Plasmid Stability
Stability is a cornerstone of any plasmid's functionality. Inducible plasmids must maintain their integrity during replication and expression phases to ensure reliable performance. A common issue encountered is the degradation or loss of plasmid DNA over time, especially under conditions that may promote instability, such as high copy number replicons or strong promoters.
Factors influencing plasmid stability include:
- Temperature variations: Fluctuations can affect the replication process.
- Host cell environment: The genetic make-up and metabolic state of the host can significantly impact plasmid stability.
- Selection pressure: Inadequate selective markers may lead to the loss of plasmid in non-selective environments.
To combat these issues, researchers often employ balanced replication strategies and optimize the growth conditions for the host cell. Each step taken to improve plasmid stability contributes to the consistency of experimental outcomes crucial in diverse fields like genetic research and biopharmaceutical production.
Cost of Induction Systems
The financial aspect of induction systems can be a significant bottleneck in research and development. Developing an effective system may require high-quality reagents, specialized media, and sophisticated machinery. These costs can quickly add up. Moreover, when considering long-term projects, the expenses associated with maintaining a robust induction system become a vital consideration.
Several factors contribute to these costs:
- Materials: High-grade chemicals and reagents are often needed for effective induction.
- Equipment: Advanced instruments for monitoring and regulating gene expression can be pricey.
- Training: Personnel need proper training in handling complex inducible systems, adding another layer of expense.
Considering these challenges, organizations may seek cost-effective alternatives, such as exploring natural inducible systems or optimizing existing protocols to reduce reliance on expensive materials. Striking a balance between cost and functionality is essential for maximizing the potential of induced plasmids.
Safety Concerns
Safety is paramount in any biotechnological endeavor, especially when it comes to induced plasmids. As these tools are often manipulated to express genes that can have significant effects on their host organisms and the surrounding environment, the implications of their use can be profound. There are several safety concerns that must be addressed:
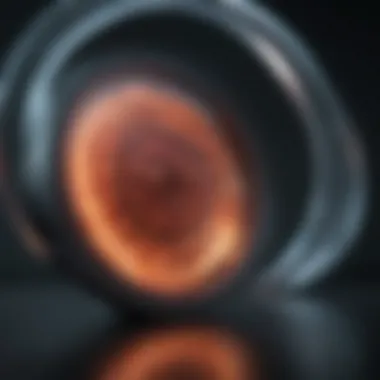
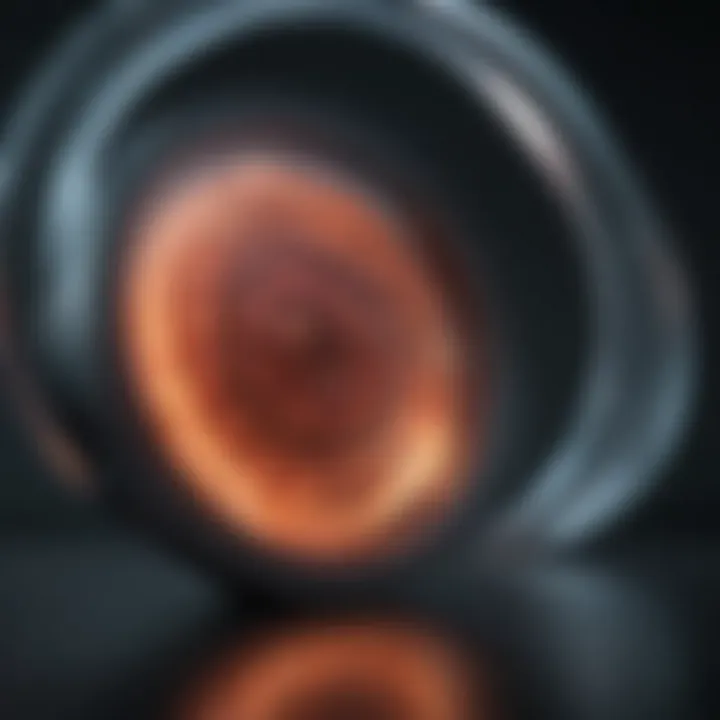
- Biocontainment risks: If an induced plasmid carries a gene that confers a survival advantage, there's a risk of unintended ecological consequences if it were to escape into natural populations.
- Toxicity: Some genes may produce harmful byproducts or alter cellular functions in a way that is detrimental to the host or surrounding organisms.
- Horizontal gene transfer: The potential for plasmids to transfer between organisms raises concerns about the spread of undesirable traits.
To mitigate these risks, researchers must implement stringent safety protocols. This involves designing plasmids with
Comparative Analysis of Inducible Plasmids
The examination of inducible plasmids through a comparative lens holds significant value in validating their varied applications and effectiveness. Such an analysis allows us to discern key differences and advantages inherent in both natural and synthetic systems, as well as evaluating the diverse induction methods that can be utilized. This section aims to explore how these comparisons can inform best practices and improve outcomes in genetic engineering endeavors.
Natural vs. Synthetic Systems
Understanding the distinction between natural and synthetic inducible plasmids is crucial for their application in different biotechnological settings. Natural systems often leverage elements that evolved to function seamlessly within organisms, allowing for high levels of specificity. For example, naturally occurring plasmids in bacteria may integrate into the host's metabolic pathways efficiently, thus demonstrating a reduced level of toxicity and a stable replication process. Though they provide a reliable framework, they can also be limited in flexibility and control over gene expression.
On the contrary, synthetic systems are tailor-made and thus offer immense versatility. Researchers can modify these plasmids to suit specific experimental needs, enabling a much tighter regulation of gene expression. By incorporating specific promoters and response elements, synthetic plasmids can be designed to switch on or off with high precision and reliability. However, this adaptability often comes with trade-offs, such as potential for instability and unexpected interactions within the host organism's cellular machinery.
In evaluating these two frameworks, some relevant points to keep in mind include:
- Effectiveness: Natural systems often have proven reliability, while synthetic systems excel in customization.
- Risk of Toxicity: Natural plasmids tend to have lower toxicity, as they are compatible with host functions compared to some synthetic variants.
- Flexibility: Synthetic plasmids score high on modifying regulatory controls.
Comparison of Induction Methods
To truly appreciate the efficacy of inducible plasmids, one must analyze the various induction methods utilized. Each method has its own advantages and implications for gene expression control. Here, we explore some of the most common approaches:
- Chemical Induction: This method involves using compounds that trigger promoter activity. The benefit of chemical induction lies in its ease of use and fast reaction times. Examples include the use of isopropyl β-D-1-thiogalactopyranoside (IPTG) in lac operon systems. However, the downside can be an accumulation of toxic metabolites that may affect cell health over prolonged exposure.
- Environmental Induction: Certain conditions, such as temperature shifts or light exposure, can also regulate gene expression. This less predictable method can lead to complex temporal controls and is useful in scenarios requiring a more gradual expression of gene products.
- Genetic Induction: In some cases, the introduction of genetic elements that respond to specific triggers can create built-in control systems. Though potentially powerful, these methods require careful design to ensure the desired outcomes without unintended consequences.
By comparing these induction methods, researchers can make more informed decisions on which method aligns best with their experimental goals. It might not be a "one-size-fits-all" situation; practicality often guides which method will be optimal for a given context.
"The potential of inducible plasmids is vast, and understanding the comparative advantages of different systems can be the key to unlocking innovative biotechnological solutions."
Future Directions in Inducible Plasmid Research
As we look to the horizon of molecular biology, the future directions in inducible plasmid research shine a light on untapped potential and challenges that lie ahead. The exploration of this area is not merely a matter of academic interest; it holds significant implications for biotechnology, agriculture, and medicine. Unraveling the mechanisms and optimizations of inducible plasmids will allow for more precise control over gene expression, paving the way for innovative solutions to longstanding biological questions and practical problems.
In considering the future, two key elements stand out: emerging technologies and ethical considerations. The rapid development of genetic tools such as CRISPR and advancements in synthetic biology are front and center in shaping how inducible plasmids will be utilized. However, these advancements come with a responsibility to address the ethical implications of manipulating genetic material.
Emerging Technologies
CRISPR adaptations
CRISPR technology stands out as a groundbreaking addition to molecular biology, offering a unique adaptability that makes it a fitting ally to inducible plasmids. It allows researchers to modify genes with an exceptional degree of precision. The ability to induce or inhibit specific genes via plasmids can be enhanced through CRISPR, which enables site-specific genome editing. This synergy amplifies the capabilities of traditional plasmids, as they can now be better tailored to express or silence genes in real-time under chosen conditions.
A noteworthy characteristic of CRISPR is its versatility. Unlike older methods that often required extensive modifications to plasmids for gene targeting, CRISPR can be directed towards various targets without the need for cumbersome restructuring. This makes it a popular choice among researchers aiming for efficiency in their experimental designs.
However, CRISPR adaptations come with potential downsides, such as off-target effects where unintended regions of the genome maybe modified, leading to unpredictable outcomes. Balancing efficacy with safety will be crucial as the field progresses.
Synthetic biology applications
Synthetic biology takes a bold step further by combining engineering principles with biological systems. The application of synthetic biology to inducible plasmids functions on the premise that biological systems can be designed to perform real-world tasks. This may include creating cells that respond to environmental stimuli through controlled gene expression, thereby leading to advancements in biomanufacturing and therapeutic strategies.
The hallmark of synthetic biology applications lies in their capability to construct entirely new biological parts, systems, or devices. This not only broadens the scope of what inducible plasmids can achieve, such as more tailored applications in agriculture and healthcare, but also enhances reliability in biotechnological applications. For instance, envisioning a crop that can produce therapeutic proteins upon exposure to specific conditions showcases the forward momentum this integration can provide.
Yet, it's essential to be mindful that with such power comes a responsibility. Synthetic biology's inherent complexity can lead to unforeseen consequences. Ensuring reproducibility, safety, and ethicality in engineered organisms must remain at the forefront.
Ethical Considerations
Turning to the ethical dimension provides a necessary counterbalance to the enthusiasm surrounding emerging technologies. The manipulation of genetic material can invoke profound questions about the implications of such interventions within ecosystems and public health. Society must grapple with whether we are crossing lines that should remain uncrossed, especially in applications involving agricultural modifications or gene therapy.
Risk assessment and public consultation are vital in navigating these challenges. Legislation and guidelines surrounding the use of inducible plasmids should not only focus on the technology but also consider long-term effects on biodiversity and human health. Ensuring that research aligns with societal values will be paramount in gaining public trust.
Ultimately, future directions in inducible plasmid research represent a blend of innovation and caution. Maximizing the utility of these tools while simultaneously honoring ethical considerations will define the trajectory of this exciting field.
Ending
In reviewing the intricate dynamics of inducible plasmids, it becomes evident that these molecular entities are not merely tools in a laboratory; they embody a profound understanding of gene regulation and manipulation in biotechnology. The ability to control gene expression through various inducible systems enables researchers to explore complex biological processes, assess gene functions, and produce heterologous proteins with remarkable precision. This capability of inducible plasmids fundamentally alters our approach to genetic engineering, providing a flexible platform for experimentation across a range of applications.
Summary of Key Findings
The journey through the world of inducible plasmids reveals several key findings:
- Plasmid Design: Understanding the structural elements that comprise inducible plasmids, including origins of replication and promoter types, is crucial for effective manipulation in genetic research.
- Mechanisms of Action: The mechanisms by which these plasmids induce gene expression, whether through chemical inducers or environmental stimuli, are diverse and tailored, allowing for a wide array of experimental conditions.
- Applications: Inducible plasmids have found their way into numerous fields, from industrial biotechnology in biopharmaceutical production to agricultural applications that enhance crop performance.
- Challenges and Future Directions: While significant progress has been made, challenges regarding the stability of plasmids, cost concerns, and safety issues persist, demanding ongoing research and alternative strategies.
Implications for the Field of Biotechnology
The implications of inducible plasmids for biotechnology are extensive. They offer a pathway not only for enhancing productivity in existing biotechnological processes but also for driving innovation and discovery in fields such as synthetic biology. A better understanding of how these plasmids function can pave the way toward more robust, efficient models of gene expression and manipulation. Additionally, as society grapples with ethical considerations surrounding genetic engineering, the insights gained from inducible plasmid research will be imperative in framing discussions and policies that govern their use.
Inducible plasmids serve as a bridge between fundamental research and applied science, highlighting the value of controlled genetic tools in the pursuit of biotechnological solutions.