Insights into Surface Plasmon Resonance Protein Interactions
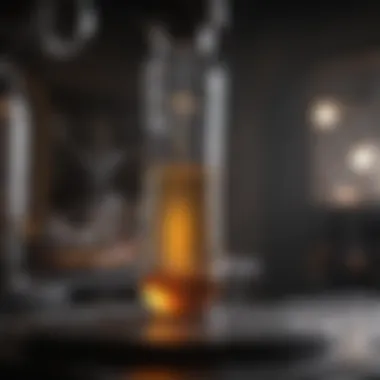
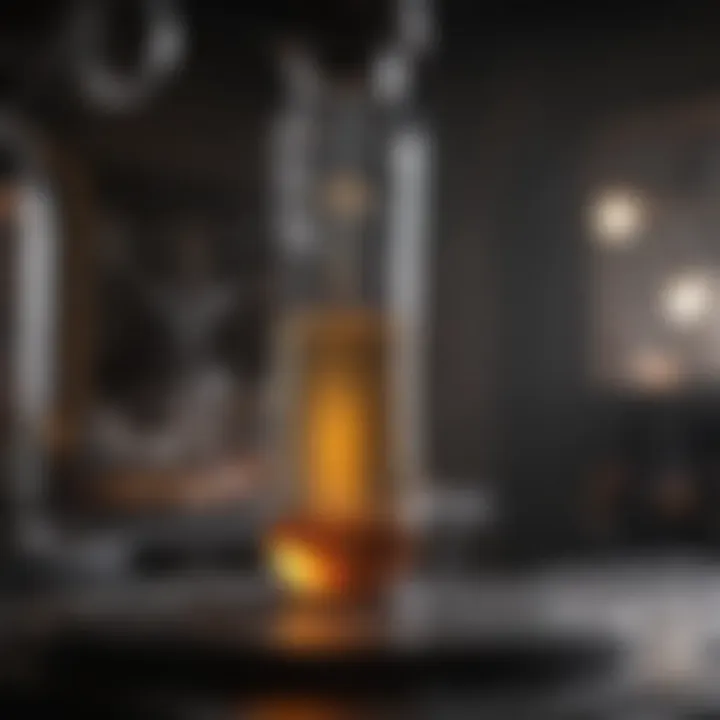
Intro
Surface Plasmon Resonance (SPR) is a cutting-edge technique, widely utilized in biochemical research. This approach provides real-time insights into protein interactions, which are fundamental to understanding various biological processes. With the continuous advancement in research methods, SPR has emerged as a significant tool that bridges theoretical concepts with practical applications in molecular biology.
Understanding protein interactions is critical for unraveling cellular mechanisms. These interactions dictate many biological functions, from signal transduction to immune responses. The importance of studying these interactions in real-time cannot be overstated, as it allows researchers to observe dynamic changes as they happen.
This article will outline the core principles of SPR, illustrate its diverse applications within various disciplines, and articulate the environmental factors that may influence experimental outcomes. Furthermore, we will delve into the methodological aspects necessary for achieving reliable protein interaction analyses. By synthesizing this knowledge, we aim to present a comprehensive examination of the significance of SPR in advancing biochemistry and molecular biology.
Research Highlights
Overview of Key Findings
One major finding highlighted in this article is how SPR enables the detection of subtle changes in refractive index caused by protein binding events. This real-time monitoring allows scientists to obtain kinetic parameters, such as binding affinity and rates of association and dissociation. Such parameters are essential for characterizing the strength and stability of biomolecular interactions.
Moreover, SPR facilitates the analysis of protein interactions in complex environments, including in vivo conditions. This feature is incredibly beneficial for understanding biological systems in their native states.
Significance of the Research
The implications of SPR go beyond individual research projects. The ability to observe protein interactions real-time holds promise for a wide range of applications, from drug discovery to diagnostics. For instance, pharmaceutical companies leverage SPR to identify potential drug candidates that can effectively bind to their target proteins.
The increased accuracy and resolution of data obtained from SPR studies can inform future research directions. As our understanding of protein behavior deepens, researchers can innovate therapeutic strategies and improve clinical outcomes for various diseases. By exploring the nuances of protein interactions through SPR, we contribute valuable information to the broader field of biochemistry and molecular biology, providing insights that can lead to groundbreaking advances in both research and clinical practice.
"The advancement of techniques like Surface Plasmon Resonance is essential for bridging the gap between experimental observations and theoretical understanding of protein interactions."
Original Research Articles
Summary of the Article
In this article, we explore the foundations and advancements surrounding Surface Plasmon Resonance. The discussion highlights methodology, empirical findings, and the contextual relevance of SPR in contemporary biological research. By addressing both the theoretical and practical aspects of SPR, we provide a streamlined view on its significance.
Author Contributions
The authors of this article have collectively contributed their expertise in biochemistry and molecular biology. Their insights stem from years of collaborative experience in protein interaction studies using SPR technology, ensuring a rich and informed narrative for the readership.
Prolusion to Surface Plasmon Resonance
Surface Plasmon Resonance (SPR) represents a pivotal advancement in our ability to study molecular interactions, particularly those involving proteins. Given the dynamic nature of biological systems, understanding these interactions is crucial. This section aims to provide a foundational overview of SPR, laying the groundwork for appreciating its significance in protein interaction studies.
Definition and Historical Background
Surface Plasmon Resonance is a sensitive optical technique used to detect changes in the refractive index near a sensor surface, usually at the interface between a metal film and the surrounding medium. The development of SPR began in the 1980s at the interface of physics and biology. Researchers recognized the potential for real-time analysis of biomolecular interactions without the need for labeling, which has traditionally posed a challenge in biological assays. The seminal contributions by researchers such as H. W. Hill and colleagues established the groundwork for SPR technology. This technique enables scientists to analyze how proteins bind and interact, which is essential for drug development and understanding disease mechanisms.
The Principle of Surface Plasmon Resonance
The working principle of SPR hinges on the phenomenon of surface plasmonsβcoherent electron oscillations at a metal-dielectric interface. When polarized light hits a metal film at a particular angle, it can excite these plasmons. This interaction causes a measurable change in the refractive index near the surface, which is correlated to the binding events between molecules.
During SPR analysis, a sample containing the analyte flows over a sensor surface coated with a corresponding binding partner, typically a ligand. As molecules interact, they alter the density of the surface layer, leading to a shift in the SPR angle or intensity. This shift is quantifiable and allows for the determination of binding kinetics, affinity, and concentration.
SPR's real-time monitoring capability and label-free detection provide distinct advantages over traditional methods, making it an invaluable tool in biochemistry and molecular biology. By providing insights into protein interactions, SPR contributes significantly to advancements in therapeutic development and our understanding of cellular mechanisms.
"SPR offers a revolutionary approach in analyzing biomolecular interactions, eliminating the need for complex labeling techniques while providing real-time data."
In summary, the introduction of SPR has transformed how researchers approach protein interaction studies. It combines historical advances in technology with an essential need in modern biochemistry to deliver insights that were previously difficult to obtain.
Understanding Protein-Protein Interactions
Understanding protein-protein interactions is fundamental in biochemistry and molecular biology. Proteins do not act alone; they often function within complexes or networks, where their interactions are critical for maintaining cellular processes. Their relevance extends to drug discovery, disease research, and many other areas. Given this, studying these interactions provides a deeper insight into how biological systems operate. Knowing how proteins interact enables researchers to develop new therapeutic strategies while also allowing for better disease understanding.
Types of Protein Interactions
Protein interactions can be categorized into several types, reflecting their diverse nature:
- Transient interactions occur for a brief period, often involved in signaling pathways. These interactions are crucial for processes like receptor-ligand binding.
- Permanent interactions are more stable and can lead to the formation of multi-protein complexes, like in structural proteins.
- Homotypic interactions involve the same protein interacting with itself, while heterotypic interactions involve different proteins, expanding the complexity of biological systems.
- Weak interactions such as van der Waals forces or hydrogen bonds can lead to significant physiological effects. These weak interactions are important in the assembly of larger protein complexes, where numerous weak bonds add up to provide stability.
These categories are not exhaustive, but they illustrate the complexity of protein interactions. Knowing what type of interactions are significant in a particular context is essential for researchers aiming for precise interventions in cellular mechanisms.
Importance of Studying Protein Interactions
Studying protein interactions is invaluable for several reasons:
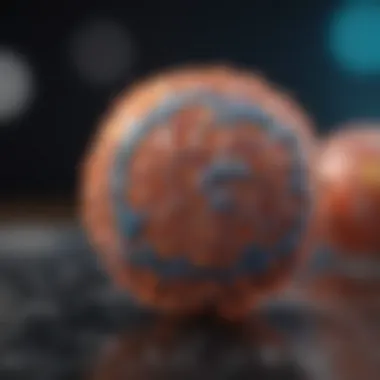
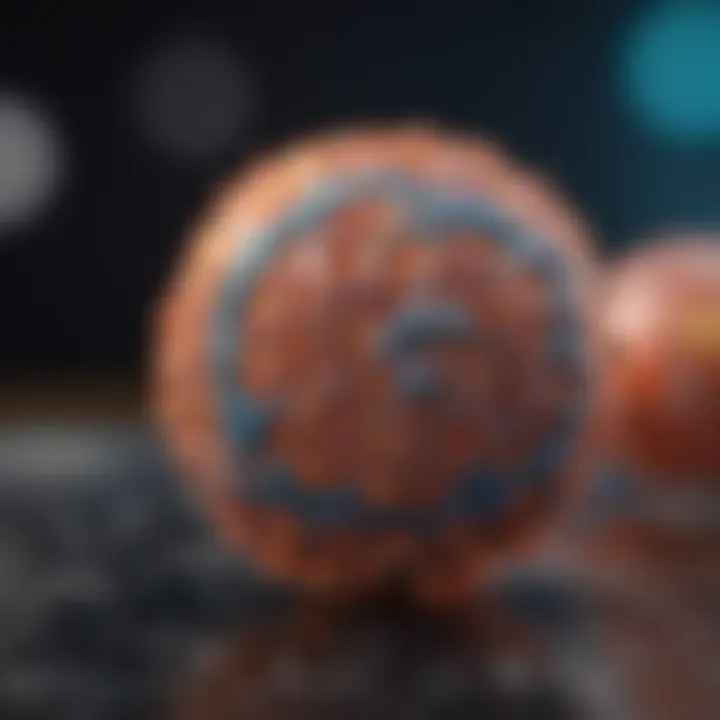
- Understanding Cellular Mechanisms: Many diseases, including cancer, are linked to the malfunction of protein interactions. By elucidating these interactions, researchers can better understand the molecular basis of diseases.
- Drug Development: Targeting specific protein interactions offers a way to create more effective therapeutic strategies. Identifying the interaction pathway can lead to the design of small molecules that disrupt these interactions, providing a potential avenue for treatment.
- Biomarker Discovery: Knowing how proteins interact can also assist in discovering new biomarkers for diseases. Changes in interaction patterns can indicate disease states, making them crucial for early diagnosis.
Research on protein-protein interactions leads to transformative insights in both basic and applied science, bridging gaps in our knowledge and presenting new avenues for innovation.
In summary, understanding the intricacies of protein-protein interactions is essential in the context of biological research. Their implications stretch beyond theri immediate interactions into wider applications in healthcare, drug discovery, and our fundamental understanding of life processes.
Theoretical Framework of SPR
The theoretical framework of Surface Plasmon Resonance (SPR) is fundamental for understanding how this technique accurately measures protein interactions. It involves principles from physics, mathematics, and chemistry that combine to create a platform for analyzing biosensor responses in real-time. Recognizing the theory behind SPR is crucial not only for operating the equipment but also for interpreting the results effectively. Each aspect of the theoretical framework contributes significantly to the reliability and efficiency of SPR in biological research.
Mathematical Principles of SPR
The mathematical principles governing SPR lie primarily in the behavior of surface plasmons and how they interact with light. At its core, SPR relies on the conditions under which electrons at the metal-dielectric interface resonate, manifesting a dip in reflectivity. The key formulas involved in SPR typically revolve around the Fresnel equations and the conditions for resonance.
- Resonance Condition: The resonance condition can be described by the equation:
[ k_i = k_t + q ]
where ( k_i ) is the wave vector in the dielectric, ( k_t ) is the wave vector in the metal, and ( q ) represents the momentum transfer to the surface plasmons. This equation is essential to understand how the binding events change when a ligand interacts with a surface-bound analyte. - Sensitivity and Response: The sensitivity of SPR is directly related to the change in refractive index near the sensor surface. Therefore, quantifying the refractive index change offers insights into the interaction kinetics of the proteins being studied. Mathematical models, such as Langmuir adsorption isotherm, enable researchers to dissect the binding responses further and derive kinetic parameters.
Understanding these mathematical principles allows researchers to calibrate their setups and interpret the resultant data more accurately. By manipulating these equations, one can predict how varying concentrations of a target molecule will influence the SPR signal.
Instrumentation and Setup
The instrumentation involved in SPR plays a vital role in its application for protein interaction studies. The setup typically includes a light source, a prism, a detector, and a sensor chip. Each component must work harmoniously to ensure valid readings and reproducibility of results.
- Light Source: A laser or a broadband light source is used to shine light onto the sensor chip. The choice of light directly influences the quality of the signal, where a fundamental role is to ensure that the wavelength used is suitable for creating surface plasmons at the metal interface.
- Sensor Chip: The sensor chip is often made of gold or silver due to their properties supporting surface plasmon formation. Coating the chip with an appropriate ligand provides a surface that interacts specifically with the analytes under investigation. Consideration of surface chemistry is crucial for optimizing binding sites and minimizing nonspecific interactions.
- Detection System: The detection system captures changes in reflectance as analytes bind to the sensor surface. Electronic readouts must be calibrated properly to ensure reproducibility. Moreover, software associated with the detection system aids in analyzing the kinetic and equilibrium constants of binding events.
The configuration and integration of these instruments determine the clarity and accuracy of the experimental outcomes. Mastery of instrumentation is paramount for both novice users and experienced researchers, as it impacts not only data quality but also the speed at which experiments can be performed.
Key Point: A well-understood theoretical framework allows for effective optimization of experimental setups and enhances the validity of the conclusions drawn from SPR experiments.
By diving into the theoretical underpinnings and honing instrumentation skills, researchers can leverage the full potential of SPR in their investigations of protein interactions.
Experimental Design in SPR Analysis
Experimental design in Surface Plasmon Resonance (SPR) analysis is fundamental to obtaining reliable and accurate results. This section discusses how proper planning and thoughtful experimental setups can enhance the study of protein interactions. A rigorous experimental design ensures that variables are controlled, making the interpretations of interactions both feasible and meaningful.
One of the benefits of a well-structured experimental design is the ability to systematically evaluate how different factors affect protein interactions. For instance, variations in concentrations, surface chemistry, and environmental conditions can significantly impact binding kinetics. By carefully designing experiments that isolate these variables, researchers can derive clearer insights into underlying mechanisms of protein interactions.
Sample Preparation and Considerations
Sample preparation is a crucial element of the SPR experimental design. High-quality samples ensure that the data collected is not confounded by impurities or unexpected variables. Key considerations in sample preparation include:
- Purity of Proteins: Ensuring that proteins are adequately purified minimizes background noise in SPR measurements.
- Concentration Determination: Accurate determination of concentrations is vital, as these can affect binding affinities and kinetics observed in the analysis.
- Buffer Selection: The selection of the right buffer is also important. Buffers should maintain stability and mimic physiological conditions to ensure relevant data.
Improper sample handling can lead to poor reproducibility and inaccurate results, reducing the utility of SPR as a tool for analyzing protein interactions.
Control Experiments and Validation
Control experiments are essential for validating the results obtained from SPR analysis. These controls help distinguish specific interactions from non-specific binding. Effective strategies for incorporating control experiments include:
- Using Blank Surfaces: This helps in understanding the background signal and allows the differentiation of specific from non-specific binding.
- Incorporating Known Interactions: Testing proteins with known binding affinities can help calibrate the system, providing benchmarks for interpreting unknown interactions.
- Replicates: Running multiple replicates of the same experiment adds to the reliability of the data, allowing researchers to assess variability and significance.
Control experiments are not just a formality; they are integral to ensuring the integrity of SPR data.
Through thoughtful design and rigorous validation methods, researchers can maximize the potential of SPR to yield insightful data on protein interactions. This approach not only boosts the credibility of the findings but also fortifies the understanding of molecular behavior in biological systems.
Factors Influencing SPR Results
Understanding the factors influencing Surface Plasmon Resonance (SPR) results is crucial for obtaining accurate and reliable data concerning protein interactions. Various elements can significantly impact the responses measured during SPR experiments. These factors include surface chemistry, ligand density, and environmental conditions such as pH, temperature, and ionic strength. Each aspect must be carefully considered and optimized to ensure meaningful interpretations in the context of biochemical research.
Surface Chemistry and Ligand Density
Surface chemistry plays a vital role in SPR experiments. The quality of the surface immobilization directly affects the analyte binding kinetics, completeness of immobilization, and binding orientation. Furthermore, ligand density must be optimized to avoid steric hindrance that can arise with a densely packed surface. An appropriate balance between surface interaction and analyte accessibility must be established. Often, a compromise between high ligand density for sensitivity and lower density to facilitate interaction is ideal.
Additionally, the nature of the surface chemistry can influence the stability of the protein layer. For instance, using chloro-silane surface modifications can yield better results for certain protein types compared to passive adsorption methods. The choice of surface functionalities will dictate how easily analytes can approach and bind to targeted molecules.
Environmental Conditions
pH
The pH of the environment is a critical factor influencing protein stability and interaction. Proteins possess specific isoelectric points, at which the net charge is zero. Deviating from this pH can lead to different charges on the protein, thus affecting binding affinities during SPR experiments. Maintaining an optimal pH can enhance the accuracy of interaction measurements. It is also crucial since many biochemical assays are sensitive to pH changes. The use of buffers can stabilize the pH, providing a consistent environment for reactions to occur.
Temperature
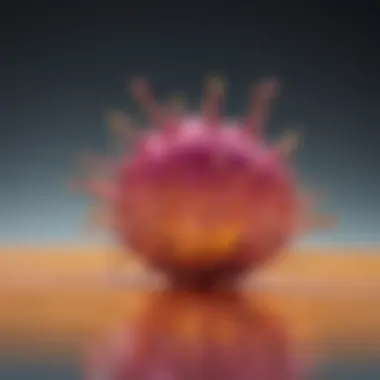
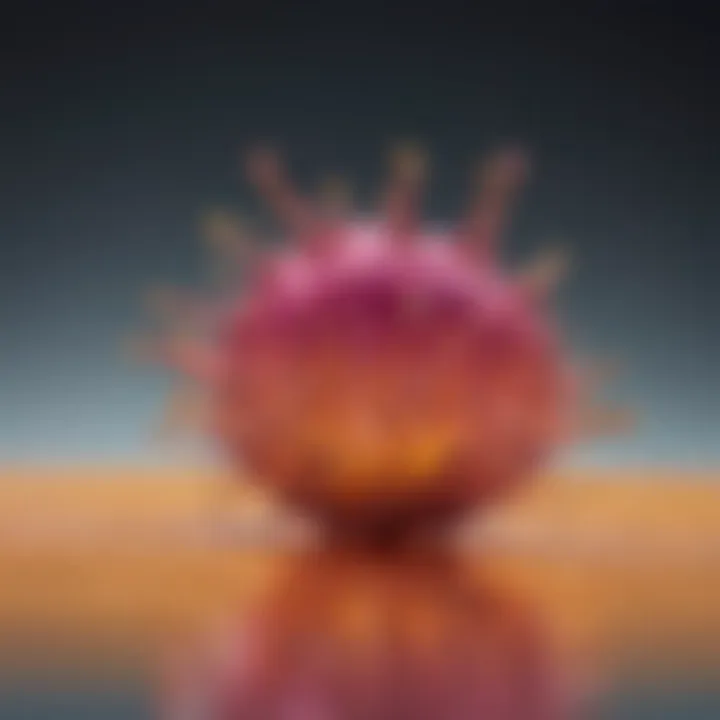
Temperature is another important element that affects molecular interactions. Increased temperatures typically enhance kinetic activity, which may lead to more rapid interactions between proteins and ligands. However, it also raises the risk of denaturation and loss of functionality for temperature-sensitive proteins. Therefore, careful calibration is needed to find a balance that maximizes interaction rates without compromising protein integrity.
Ionic Strength
Ionic strength affects electrostatic interactions between charged molecules. High ionic strength can screen charges, reducing the electrostatic repulsion or attraction between proteins and surfaces. This can be advantageous in cases where nonspecific binding may interfere with measurements. However, too high ionic strength may lead to protein precipitation or reduced binding efficiencies. Thus, careful optimization of ionic strength is important for maintaining effective interactions in SPR experiments.
It is essential to acknowledge the influence of various environmental parameters to ensure accurate and reproducible SPR results in protein interaction studies.
Data Analysis in SPR Experiments
Data analysis is a critical component of Surface Plasmon Resonance (SPR) experiments. It transforms raw experimental data into meaningful conclusions about protein interactions. Effective data analysis can yield insights into binding kinetics, affinity measurements, and overall interaction dynamics. Without sound data analysis, the potential of capturing real-time interactions may not be realized.
Kinetic and Equilibrium Analysis
Kinetic analysis involves studying the rates at which proteins bind to and dissociate from each other. This is fundamental to understanding the dynamics of protein interactions. For instance, the association rate constant (k_on) reveals how quickly a protein binds, while the dissociation rate constant (k_off) indicates how fast it unbinds.
To compute these constants, the sensorgram, which is a plot of the signal change over time, is analyzed. The shapes of these curves provide insights into the interaction mechanisms. A simple model assumes a one-to-one interaction, which simplifies calculations but may not apply in all cases.
Equilibrium analysis, on the other hand, focuses on the equilibrium constant (K_d), which represents the ratio of dissociation to association rates at equilibrium. The equilibrium constant can provide crucial information about the strength of the interaction, impacting potential applications in drug discovery and molecular biology.
In summary, kinetic and equilibrium analysis offers valuable metrics that enhance our understanding of how proteins interact in different environments.
Software and Computational Tools
The analysis of SPR data requires specialized software and computational tools. These tools aid in modeling, simulating, and interpreting the binding events. Many researchers rely on dedicated SPR software such as BIAevaluation, Scrubber, or TraceDrawer, which can streamline the analysis process significantly.
Using such software allows for various fitting models to be applied, from simple to complex mass action models. For example, the choice of model affects the calculated kinetic parameters, which can lead to varying conclusions if not selected wisely.
Some tools facilitate real-time monitoring and enable batch processing of multiple sensorgrams, which is especially useful for high-throughput studies. In addition to established software solutions, programming languages like R and Python are also increasingly used for custom analyses, offering flexibility in data processing and visualization.
Applications of SPR in Biological Research
Surface Plasmon Resonance (SPR) serves as a pivotal tool in biological research. It provides quantitative data about protein interactions, enabling researchers to identify binding affinities and kinetic rates. This technique supports various applications crucial for advancements in biochemistry and molecular biology, particularly in understanding complex biological systems.
By employing SPR, researchers can observe real-time interactions between biomolecules without the need for labels. This offers significant advantages, such as preserving the native structure of proteins during analysis. Moreover, the sensitivity of SPR allows for the measurement of low-affinity interactions that are often critical in biological pathways. The importance of SPR encompasses several facets, including its role in drug discovery and investigating disease mechanisms.
Drug Discovery and Development
In the domain of drug discovery, SPR plays a vital role in the identification and optimization of drug candidates. It allows for high-throughput screening of interactions between potential drugs and target proteins, leading to more efficient discovery processes.
Some key aspects of using SPR in drug development include:
- Real-time Monitoring: SPR enables the tracking of binding events as they occur, providing insights into kinetics and affinity. This data helps in optimizing lead compounds.
- Target Validation: SPR assists in confirming that proposed targets interact with their drug candidates. Validating targets early in the discovery process minimizes time and resources spent on unproductive leads.
- Assessment of Drug Dynamics: Understanding the binding dynamics between drugs and their targets can inform structure-activity relationships, facilitating better compound design.
Using SPR in drug discovery ultimately aims to shorten development timelines, reduce costs, and improve the overall success rate of new therapeutics.
Investigating Disease Mechanisms
The application of SPR extends beyond drug development; it also significantly enhances the understanding of disease mechanisms. By studying protein interactions implicated in various diseases, researchers can uncover pathways that contribute to disease pathology.
Key benefits of utilizing SPR in this context include:
- Mapping Interaction Networks: SPR can help delineate complex interaction networks of proteins involved in specific diseases. This is crucial for identifying potential biomarkers.
- Understanding Pathogenic Mechanisms: Investigating how different proteins interact in pathological conditions can reveal mechanisms of disease progression.
- Evaluating Therapeutic Targets: SPR provides the means to evaluate how effectively potential therapeutic targets bind with disease-related proteins, guiding future research and development.
As a result, SPR serves as a fundamental technique in both understanding the intricacies of diseases and developing targeted therapies. Its versatility and precision solidify SPR's role in enriching our understanding of biological research.
Limitations and Challenges of SPR
In the field of biochemistry and molecular biology, Surface Plasmon Resonance (SPR) stands as a crucial tool for analyzing protein interactions. However, it is essential to address its limitations and challenges to fully appreciate its utility in research. Understanding these factors will help in making informed decisions during experimental design and data interpretation. Here, we explore significant concerns such as sensitivity and specificity issues, along with time and resource constraints.
Sensitivity and Specificity Issues
Sensitivity and specificity are critical metrics when evaluating SPR as a method for protein interaction studies. Sensitivity refers to the ability of the SPR system to detect low concentrations of analytes, while specificity pertains to its capability to discern relevant interactions amidst a backdrop of noise from non-specific bindings.
One notable challenge is the inherent limitations in detecting low-affinity interactions. Certain protein interactions, especially transient or weak ones, may fall below the detection threshold of conventional SPR systems. This can lead to the underrepresentation of biologically relevant interactions in experimental results. To overcome this issue, researchers can consider enhancing sensitivity by optimizing conditions such as increasing the surface density of ligands or utilizing more sensitive detection techniques, yet this may introduce other complexities.
Moreover, cross-reactivity is another concern. Non-specific binding can skew results and lead to false-positive signals. To counteract this, thorough experimental controls must be implemented. This might include running parallel experiments with a control protein that does not interact with the target, thereby providing a baseline for evaluating specificity. Overall, a careful balance must be struck between enhancing sensitivity while maintaining specificity to ensure robust and reliable data from SPR analyses.
Time and Resource Constraints
While SPR offers real-time interactions monitoring, it does not come without its demands regarding time and resources. The experimental setup for SPR analysis can be labor-intensive. Researchers need to dedicate significant time for instrument calibration, surface preparation, and optimization of binding conditions. When multiple proteins or conditions are tested, these requirements compound, leading to longer durations for complete analyses.
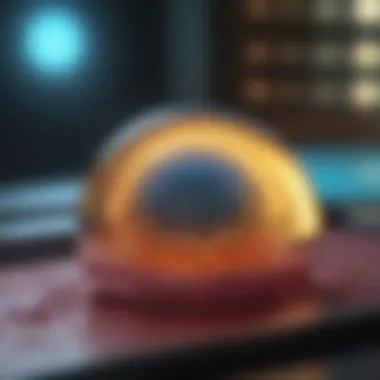
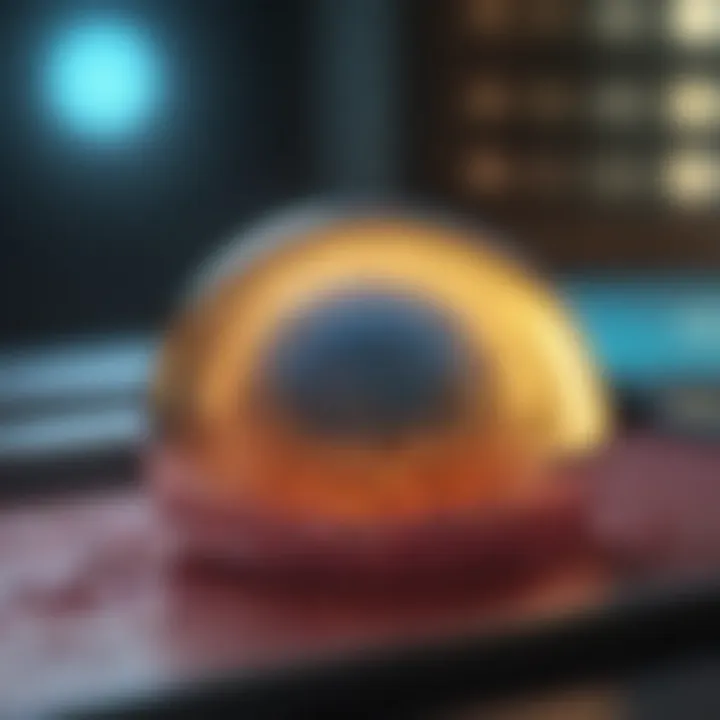
Additionally, the cost of SPR equipment and maintenance can be substantial. High-quality SPR devices like the Biacore systems can be an investment, often prohibiting access for smaller laboratories or institutions. The necessity for skilled personnel to operate such sophisticated instruments further contributes to resource constraints.
To mitigate these challenges, researchers should carefully plan their experiments, ensure efficient use of instrumentation, and consider integrating alternative methods or techniques when appropriate. Collaborations among institutions can also be beneficial by pooling resources and expertise, thereby extending the availability of SPR technology.
"Understanding the limitations of Surface Plasmon Resonance is key for optimizing its application in research. Addressing issues related to sensitivity, specificity, and resource constraints enables more accurate and efficient experimental design."
Neither sensitivity nor time constraints should deter the pursuit of utilizing SPR in research. Instead, acknowledging these challenges encourages innovation and the development of solutions that will improve the overall methodology.
Comparative Techniques to SPR
In the exploration of protein interactions, Surface Plasmon Resonance (SPR) stands as a prominent method due to its real-time analysis capabilities. However, the landscape of biophysical techniques includes various alternatives that researchers can leverage. Understanding these comparative techniques is crucial for contextualizing the applications of SPR, identifying its strengths, and recognizing its limitations. Each technique provides unique insights that can complement or serve as alternatives to SPR. The insights gained from utilizing various methods can enhance the depth of research and provide a more comprehensive understanding of protein interactions.
Surface Plasmon Resonance Imaging (SPRi)
Surface Plasmon Resonance Imaging (SPRi) represents an advanced adaptation of conventional SPR. This method offers the capability to observe multiple interactions simultaneously across a surface. It generates spatially-resolved images, allowing researchers to see where interactions take place. This is particularly significant in high-throughput settings or when screening numerous samples. The ability to gather data in a multiplexed manner enables a more efficient approach to analyzing protein interactions.
SPRi's unique feature is its high throughput and ability to provide interaction maps. This helps in screening large libraries of candidates rapidly. However, despite its advantages, there are certain challenges. The complexity of data interpretation may increase due to multiple interactions being analyzed simultaneously. Understanding the fine details of individual interactions can become more difficult.
Other Biophysical Methods
NMR
Nuclear Magnetic Resonance (NMR) spectroscopy is a powerful tool in studying biomolecular structures and dynamics. It allows researchers to view protein structures in solution, providing valuable information about the protein's environment and interactions. This characteristic of NMR makes it an integral method for understanding the nuances of protein-protein interactions in a native context.
Key to NMR's utility is its ability to provide dynamic information, which is crucial for analyzing interactions that may be transient. This is particularly useful when studying large proteins or complexes. However, one significant drawback is the concentration of samples required. High concentrations are often essential for NMR to yield reliable results, posing challenges when working with scarce or sensitive samples.
X-ray Crystallography
X-ray Crystallography is another foundational technique for elucidating protein structures. It relies on the diffraction of X-rays passing through protein crystals to reveal atomic-level details. This technique is invaluable for obtaining high-resolution structural information. Its key characteristic is the ability to visualize the precise arrangement of atoms within a protein complex, allowing for in-depth analysis.
While X-ray Crystallography offers exceptional detail, it comes with its unique challenges. Protein crystallization can be difficult and time-consuming, often requiring specialized conditions that may not represent physiological environments. Thus, the applicability of crystallographic data to dynamic, in vivo scenarios can be limited.
Mass Spectrometry
Mass Spectrometry (MS) is a technique that measures the mass-to-charge ratio of ions. It provides insights into protein identity, structure, and interactions. One of its key characteristics is sensitivity, allowing it to detect low-abundance proteins and post-translational modifications. This sensitivity makes MS a beneficial choice for analyzing complex biological samples.
A unique aspect of mass spectrometry is its capability to analyze complex mixtures without the need for labeling. This feature enhances its flexibility in various experimental setups. However, one cannot overlook the challenges associated with MS, such as the requirement for sample ionization and potential loss of non-covalent interactions during the analysis process.
Overall, understanding these comparative techniques to SPR not only enhances the interpretation of experimental results but also guides researchers in selecting the most appropriate methods for their specific studies.
Future Directions in SPR Research
The field of Surface Plasmon Resonance (SPR) continues to expand and evolve, making it essential to consider the future directions of this technique. As researchers deepen their understanding of protein interactions, the implications of advancements in SPR can significantly enhance both basic and applied sciences. The focus on technology and clinical applications reflects the increasing relevance of SPR in modern biochemistry.
Technological Innovations
Innovations in technology are driving the future of SPR research. These advancements focus on improving sensitivity, resolution, and user-friendliness. One notable trend is the development of ultra-sensitive SPR sensors. These sensors can detect minimal concentrations of biomolecules, which broadens the scope of applications in drug development, diagnostics, and environmental monitoring. Furthermore, miniaturization of SPR devices is becoming popular. Smaller, portable systems enable real-time analysis in various settings, including field studies and clinics.
Additionally, advances in data processing and analysis software have emerged. New algorithms allow for more precise kinetic modeling and interaction analysis. This integration of machine learning into data interpretation can reveal deeper insights from SPR experiments, thereby enhancing our understanding of complex biological systems. These innovations highlight the importance of interdisciplinary collaboration among physicists, biochemists, and computer scientists.
Expanding Applications in Clinical Research
Expanding the applications of SPR within clinical research is a critical aspect of future directions. SPR is poised to play a significant role in personalized medicine by enabling real-time monitoring of biomolecular interactions in the context of patient-specific therapies. For instance, the ability to measure how drugs interact with their targets in real-time allows for more tailored treatment regimens that could reduce side effects and enhance efficacy.
Moreover, SPR can aid in the early detection of diseases by enabling the identification of disease biomarkers. This aspect is particularly valuable in oncology, where the ability to detect cancer-related proteins could significantly impact early diagnosis and treatment strategies. The integration of SPR technology in clinical diagnostics can streamline workflows in laboratories, making tests faster and more efficient.
"The integration of SPR technology in clinical diagnostics can streamline workflows, enhancing the speed and efficiency of tests."
Overall, the future directions in SPR research suggest a strong potential for technological advancements and clinical applications. The focus on enhancing SPR techniques will likely facilitate more robust research outcomes, further cementing its role as a vital tool in understanding protein interactions at a molecular level.
Finale
In this article, the conclusion section encapsulates the critical insights gained from exploring surface plasmon resonance (SPR). The significance of understanding protein interactions through this technique cannot be overstated, particularly regarding its contributions to both basic and applied science. SPR offers a highly sensitive, real-time method to analyze molecular interactions, enhancing our appreciation of the complexities of biochemical systems.
Summary of Key Insights
Through this exploration, several key insights emerge:
- Real-Time Analysis: SPR allows researchers to observe protein interactions as they happen. This immediacy provides a dynamic view that traditional methods lack.
- Quantitative Data: The technique offers quantitative measures of affinity and kinetics, critical for understanding the strength and timing of interactions.
- Versatility: SPR is not limited to specific proteins or conditions, making it applicable across various fields, from drug discovery to diagnostics.
- Impact of Environmental Conditions: Factors like pH, temperature, and ionic strength can significantly influence SPR results, underscoring the necessity of careful experimental design.
Understanding these insights helps researchers employ SPR more effectively, ensuring data reliability and relevance in their studies.
The Role of SPR in Future Research
Moving forward, the role of SPR in research is poised for enhancement. The technological advances in instrumentation will likely improve sensitivity and reduce costs. As we uncover more complex biological processes, SPR's application in clinical research will expand. This technique can serve as a vital tool in identifying biomarkers, optimizing drug formulations, and elucidating disease mechanisms. As we enhance our methodologies and tools, the breadth of SPR's application in both academic and industrial research will certainly grow, aligning with the ultimate goal of unraveling the intricacies of protein interactions in biological systems.
In summation, the future of SPR in research looks promising, with the potential to revolutionize our understanding of molecular interactions and their implications in health and disease.