Nucleic Acid Tests vs PCR: Key Differences Explained
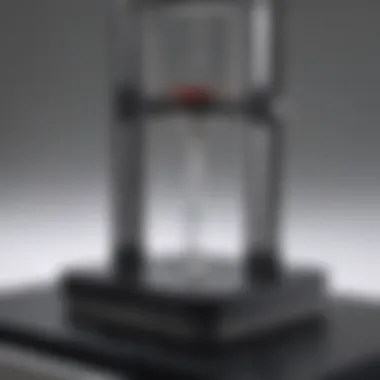
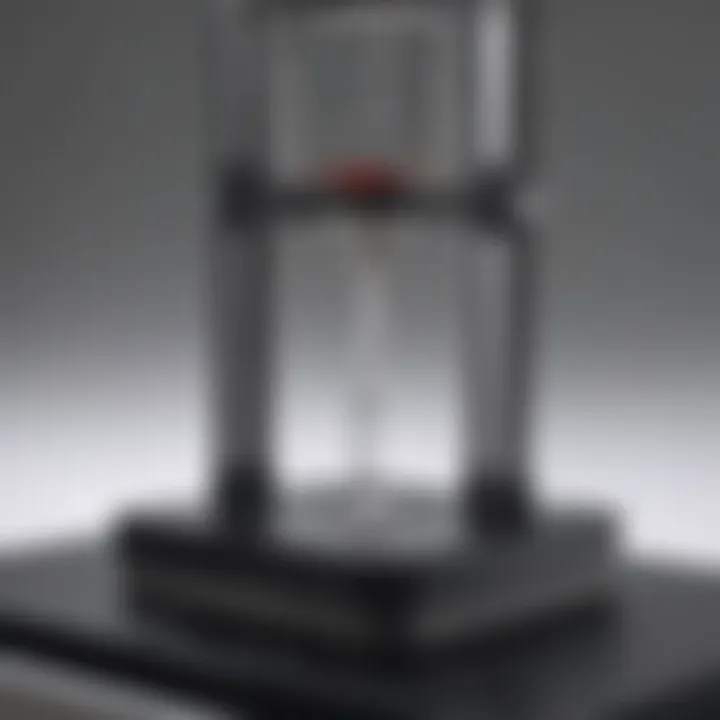
Intro
In the realm of molecular diagnostics, nucleic acid tests and polymerase chain reaction (PCR) systems play pivotal roles. These techniques are essential in detecting and identifying pathogens, thereby making them fundamental in the study and management of infectious diseases. This article intends to provide a thorough exploration of these two methodologies.
Understanding the distinctions between nucleic acid tests and PCR requires a closer examination of their operational processes, applications in various sectors, and how they perform in real-world scenarios. This analysis will not only clarify their unique attributes but will also highlight their respective significances in the scientific community.
As we navigate through this discussion, expect to gain insights into how these tests are conducted, where they excel, and what limitations they may present. The intent is to furnish researchers, students, and industry professionals with a clear perspective on these methodologies, fostering informed choices in related fields.
Research Highlights
Overview of Key Findings
Both nucleic acid tests and PCR are crucial for diagnosing infectious diseases, yet their methodologies differ significantly. PCR amplifies DNA or RNA sequences to enable detection, commonly used for viruses such as SARS-CoV-2. On the other hand, nucleic acid tests can range from PCR-based methods to other approaches such as hybridization techniques, providing flexibility in design.
- Nucleic Acid Tests: These can be subdivided into areas like reverse transcription quantitative PCR (RT-qPCR), which has seen extensive use in the COVID-19 pandemic.
- PCR Methods: Variants include real-time PCR, digital PCR, and multiplex PCR, allowing multiple targets to be examined simultaneously.
Significance of the Research
The implications of these testing methods extend beyond academic inquiry. In a clinical environment, the ability to accurately diagnose conditions leads to timely interventions. As health challenges become more complex and intertwined with genetics, understanding these testing frameworks is vital. Furthermore, discussions around these methodologies inform policy-making and clinical guidelines that impact public health.
This understanding not only enriches academic discourse but also reinforces the applied aspects of nucleic acid testing and PCR in real-world diagnostics.
Culmination
Navigating the landscape of nucleic acid tests and PCR unveils critical insights that transcends laboratory work, weaving into the fabric of public health and global efforts to combat disease. This article strives to delineate these methodologies, evaluating their strengths and weaknesses while articulating their relevance in contemporary diagnostics.
Intro to Nucleic Acid Testing
Nucleic acid testing (NAT) represents a pivotal aspect in the realm of molecular diagnostics. With advancements in technology and an increasing demand for precise and rapid diagnostic methods, understanding nucleic acid testing becomes crucial. This section explores the definition and significance of these tests, providing a framework for their role in modern medicine. The importance of nucleic acid testing lies in its ability to detect specific genetic materials, paving the way for earlier diagnoses of diseases and enhancement of treatment strategies.
Definition and Overview
Nucleic acid testing is a diagnostic technique aimed at identifying and quantifying nucleic acids—DNA and RNA—within various biological samples. The term encompasses a range of methods, each designed to analyze genetic material due to the rising need in sectors like infectious disease management and genetics.
The core principle of these tests revolves around amplifying the target nucleic acid segments for easier detection. By focusing on the genetic material of pathogens or specific genes linked to diseases, nucleic acid tests can reveal information that traditional methods may overlook. This inherent specificity elevates their utility in diagnosing conditions such as viral infections, genetic disorders, and cancers.
Significance in Modern Medicine
In recent years, the significance of nucleic acid testing in modern medicine has expanded dramatically. Its adoption has been instrumental in revolutionizing diagnostic practices. The capability to detect infections at an early stage or identify genetic predispositions strongly affects patient management.
Key benefits of nucleic acid tests in modern medicine include:
- Rapid results: Many nucleic acid tests can deliver results in a matter of hours, which is crucial for timely intervention in infectious diseases.
- High specificity: These tests minimize false positives, making them reliable for precise diagnosis.
- Broader applicability: NAT can be applied to various fields, including oncology, infectious disease tracking, and hereditary genetic testing.
Furthermore, the evolution of nucleic acid testing technologies has implications beyond individual patient care. Public health responses have also benefited through improved surveillance of disease outbreaks, enabling health officials to track and control the spread of infectious agents effectively.
"Nucleic acid tests provide a bridge between basic research and clinical applications, facilitating the translation of molecular biology insights into practical diagnostic tools."
As we delve deeper into specific methodologies and applications of nucleic acid testing, it becomes clear that these diagnostic methods hold potential for shaping the future of pathology and personalized medicine.
Foreword to PCR
Polymerase Chain Reaction, or PCR, is a crucial technique in molecular biology. It revolutionized the field by allowing scientists to amplify small segments of DNA. This amplification process is essential for various applications, including medical diagnostics and forensic science. Understanding PCR is vital because it serves as a foundational tool in many research and diagnostic settings. The ability to replicate DNA with precision enables quicker turnaround for results, making PCR an important method in both clinical and laboratory environments.
Basic Principles of PCR
The process of PCR relies on a few key principles that make it effective. First, the technique involves repeated cycles of heating and cooling. This is necessary for denaturation, annealing, and extension of DNA.
- Denaturation: The double-stranded DNA is heated to around 94-98°C. This separates the strands, creating single-stranded DNA.
- Annealing: The temperature is lowered to about 50-65°C. During this phase, primers, which are short DNA sequences, bind to specific locations on the single-stranded DNA.
- Extension: The temperature is raised again to about 72°C. At this point, a DNA polymerase enzyme synthesizes the new DNA strands by adding nucleotides complementary to the template strand.
These three steps repeat, generally for 25-35 cycles. Each cycle doubles the amount of target DNA, leading to exponential amplification. The specificity and efficiency of PCR come from the precise conditions under which these reactions occur, including temperature, time, and reagents used.
Historical Context and Development
PCR was developed in 1983 by Kary Mullis. His invention opened new doors in genetic research and diagnostics. Before PCR, DNA amplification was a complex and time-consuming endeavor. The efficiency of PCR brought about a paradigm shift in how scientists approach genetic analysis.
Initially met with skepticism, the method gained acceptance as its applications became evident. PCR's impact on forensics, medicine, and research cannot be overstated. Case studies of criminal investigations have showcased how PCR can analyze minuscule amounts of DNA, establishing a person's presence at a crime scene.
Over time, advancements have led to various modifications of the PCR technique, resulting in faster and more efficient protocols. Real-time PCR, for example, allows quantification of DNA in real-time, which has further expanded PCR's utility in different scientific fields.
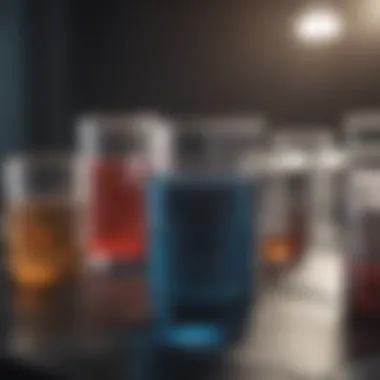
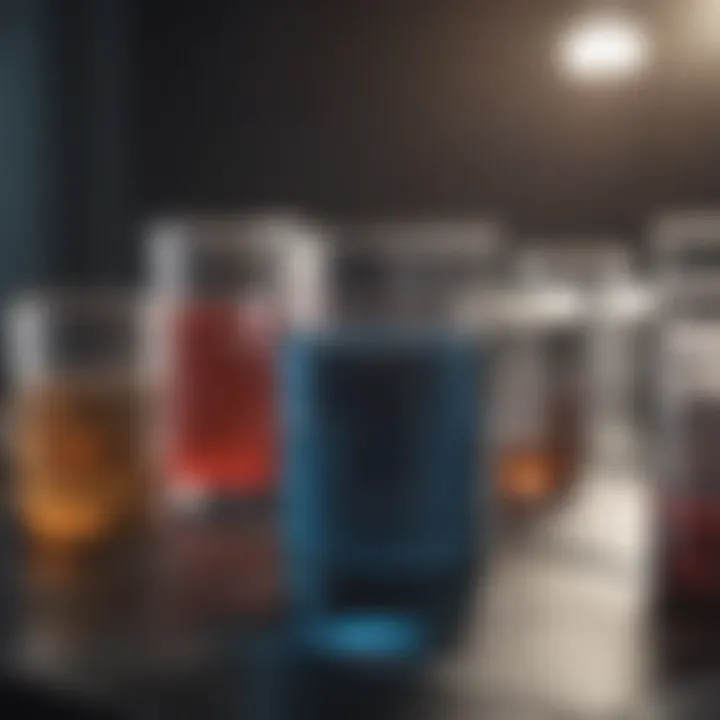
In summary, PCR is a foundational molecular biology technique that has transformed the landscape of DNA analysis since its inception. Understanding both its basic principles and historical context is essential for anyone involved in the life sciences.
Methodology of Nucleic Acid Tests
The methodology of nucleic acid tests plays a critical role in modern diagnostics. These techniques allow for the detection and quantification of nucleic acids, such as DNA or RNA, providing valuable insights into various biological processes. Their significance is particularly pronounced in infectious disease diagnostics, genetic testing, and research applications.
Types of Nucleic Acid Tests
In the realm of nucleic acid testing, several methods have gained prominence. These include Real-time PCR, LAMP, and Hybridization-Based Tests. Each of these methods has specific characteristics and applications.
Real-time PCR
Real-time PCR, also known as quantitative PCR, enables the simultaneous amplification and quantification of DNA. This technique is widely used because it provides real-time results, which is essential in situations where rapid diagnosis is crucial. Unlike traditional PCR, where the results are obtained after the completion of amplification, Real-time PCR monitors the reaction as it occurs.
The key characteristic of Real-time PCR is its ability to measure the amount of DNA in a sample while amplification is happening. This feature allows researchers to determine the initial quantity of target nucleic acid. However, the complexity of the assay setup and the need for specialized equipment can be considered disadvantages.
LAMP
Loop-mediated isothermal amplification (LAMP) is a technique that amplifies nucleic acids at a constant temperature. This method is notable for its simplicity and speed. It does not require sophisticated thermal cycling devices, making it accessible for various settings, including field testing.
LAMP's key characteristic is its high specificity and sensitivity, which enable detection of low levels of nucleic acids. This is particularly beneficial in resource-limited settings. However, LAMP can be influenced by the quality of the sample, which is a limitation to consider.
Hybridization-Based Tests
Hybridization-based tests include methods like microarrays and Southern blotting. These techniques are designed to measure the presence and quantity of specific nucleic acid sequences. They are beneficial in applications where a comprehensive view of genetic markers is required.
The unique feature of hybridization-based tests is their ability to analyze multiple targets simultaneously. This multi-target capability is significant in complex diagnostics, such as those for infectious diseases. Nevertheless, these tests often require more time and technical expertise compared to other nucleic acid methods.
Sample Collection and Preparation
Sample collection and preparation are crucial steps in nucleic acid testing. The reliability of test results largely depends on the quality and integrity of the sample. Proper procedures must be followed to avoid contamination and ensure accurate analysis. Common sample types include blood, tissues, and swabs, each requiring specific handling protocols. Attention to detail in these procedures can greatly enhance the effectiveness of the testing methodologies.
Methodology of PCR
The methodology of polymerase chain reaction (PCR) holds significant importance in modern molecular biology and diagnostic applications. This section delves into the systematic approach that defines the PCR process, highlighting its steps, the equipment needed, and the reagents involved. Understanding these elements is crucial for scientists who aim to leverage PCR for precise DNA amplification, and it serves as a guide for optimizing the process in various applications, such as medical diagnostics and forensic analysis.
Steps in the PCR Process
The PCR process is divided into three fundamental steps: Denaturation, Annealing, and Extension. Each step plays a distinct role in the overall amplification of DNA.
Denaturation
Denaturation is the first step of the PCR process. This phase involves heating the reaction mixture to a temperature around 94-98°C. The heat causes the double-stranded DNA to unwind and separate into two single strands. This is a key characteristic of denaturation, allowing access to the DNA sequence of interest. This step is beneficial because it ensures that all targeted sequences are available for copying in subsequent stages. However, a notable disadvantage is that excessive heat can lead to degradation of sensitive components, thus it's crucial to optimize the temperature appropriately for the specific DNA being worked with.
Annealing
The second step is Annealing, where the temperature is lowered to 50-65°C. During this step, specific short DNA sequences, known as primers, bind to complementary sequences on the single-stranded DNA. The primer’s specificity is vital as it determines the exact location where amplification will initiate. A unique feature of annealing is its reliance on the precise matching of primer sequences to the target DNA, which makes it a powerful step in enhancing the specificity of the PCR process. However, if the temperature is too low, non-specific binding may occur, leading to the unintended amplification of non-target sequences.
Extension
The final step of PCR is Extension, typically occurring at about 72°C. In this phase, the enzyme Taq polymerase, which is heat-stable, adds nucleotides to the growing DNA strand, synthesizing a new strand complementary to the template. The unique characteristic of extension is the efficiency with which Taq polymerase operates, allowing for rapid amplification of the target DNA sequence. While this step has considerable advantages in terms of speed and effectiveness, it is vital to ensure the enzyme's activity is not hampered by inhibitors present in the sample, as this can significantly impact the yield of the PCR.
Equipment and Reagents
The successful execution of PCR requires specific equipment and reagents. Essential equipment typically includes a thermocycler, which provides the precise temperature control needed for each step of the PCR cycle. The reagents essential for PCR include DNA, primers, Taq polymerase, nucleotides, and buffer solutions.
The combination of these components is critical.
- Thermocycler: Controls the temperature changes during PCR cycles.
- Taq Polymerase: This enzyme is essential for synthesizing DNA.
- Primers: The short sequences that initiate replication.
- Buffer Solutions: Maintain the optimal pH and ionic conditions of the reaction.
Understanding the methodology of PCR is essential for accurate and effective molecular diagnostics.
This detailed overview lays a strong foundation for understanding PCR processes and their practical applications, setting the stage for future explorations in subsequent sections.
Applications of Nucleic Acid Testing
Nucleic acid testing plays a critical role in various fields of medicine and research, providing depth and accuracy in diagnostics. It is particularly important due to its ability to detect the genetic material of pathogens, thus offering insights that conventional methods cannot. As we explore the applications of nucleic acid testing, it is important to recognize the specific elements and benefits characterized by two main areas: infectious disease diagnostics and genetic testing.
Infectious Disease Diagnostics
One of the most prominent applications of nucleic acid testing is in diagnosing infectious diseases. Nucleic acid tests (NATs) such as polymerase chain reaction (PCR) have revolutionized how clinicians approach diagnoses of pathogens like viruses and bacteria.
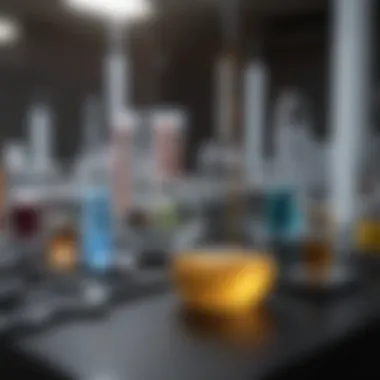
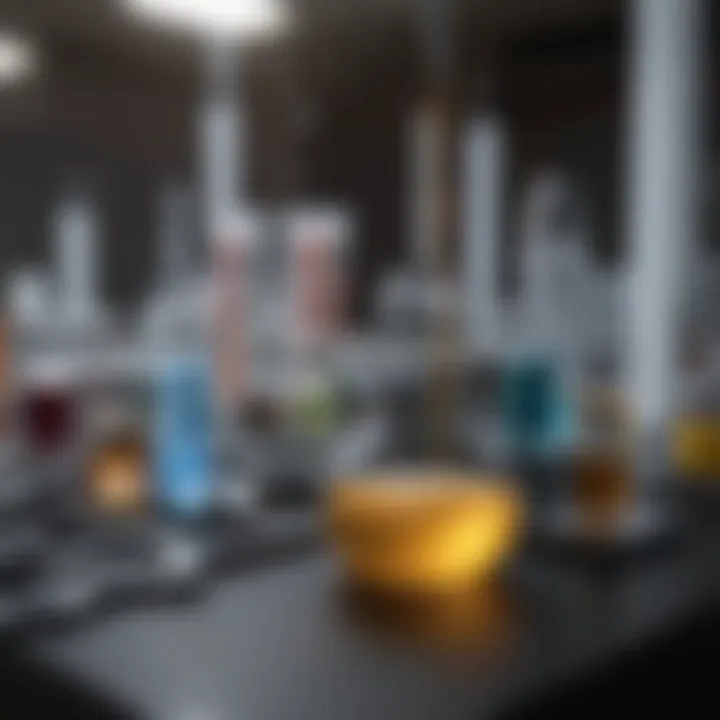
Key aspects include:
- Early detection: Nucleic acid tests can identify infections at an early stage by detecting the genetic material of the pathogen even before the body produces antibodies. This early intervention can lead to timely treatment, reducing disease spread and improving patient outcomes.
- Specificity and Sensitivity: These tests provide high levels of specificity and sensitivity. This means that they can accurately identify the target nucleic acids while minimizing false positives and negatives, which is essential in clinical settings.
- Application in Outbreaks: During outbreaks, NATs quickly provide the required information to control and manage the situation. For instance, the COVID-19 pandemic highlighted how nucleic acid testing enabled swift identification of infection cases and guided public health responses.
Genetic Testing and Research
Nucleic acid testing extends beyond clinical diagnostics into genetic testing and research, offering insights into hereditary diseases and genetic predispositions.
Several important points are:
- Understanding Genetic Disorders: Nucleic acid tests can identify mutations or variations in DNA that are responsible for genetic disorders. This allows for better diagnosis and risk assessment in patients, enhancing personalized medicine.
- Research and Development: In research, nucleic acid testing contributes to the understanding of genetic functions and interactions. It supports the development of new therapies, including gene therapy, which targets genetic defects directly.
- Biobanking: Nucleic acid integrity is crucial for biobanking studies. These studies rely on the quality of DNA and RNA extracted from biological samples to ensure that future research remains valid and reliable.
In summary, the implications of nucleic acid testing in genetics and research are profound. It not only aids in understanding individual genetic profiles but also accelerates advancements in medical science.
Nucleic acid tests establish a connection between genetic research and clinical applications, forming a bridge for better healthcare delivery.
Through these applications, nucleic acid testing proves essential for unlocking new understanding in both infectious diseases and genetic conditions, highlighting its importance in our evolving healthcare landscape.
Applications of PCR
Polymerase Chain Reaction (PCR) has revolutionized the field of molecular biology, offering diverse applications across multiple domains. Its significance lies in the ability to amplify minute quantities of DNA into millions of copies, thus enabling a range of analytical techniques. This amplification is crucial for various applications such as medical diagnostics and forensic analysis, each contributing substantially to advancements in their respective fields.
Medical Diagnostics
PCR's role in medical diagnostics is pivotal. It enables the detection of various pathogenic organisms, including bacteria and viruses, with high sensitivity and specificity. This is particularly vital in the diagnosis of infectious diseases. For instance, PCR is extensively used for identifying pathogens in respiratory infections, such as those caused by SARS-CoV-2 or Mycobacterium tuberculosis.
- Rapid Results: One of the significant benefits of PCR in medical diagnostics is the quick turnaround time. Patients receive diagnoses within hours rather than days, which can be crucial for timely treatment.
- Sensitivity: PCR can detect very low levels of a pathogen, often allowing clinicians to identify infections that might not be detectable through culture methods. This heightened sensitivity can significantly influence clinical management and patient outcomes.
- Application in Genetic Testing: PCR is also fundamental in genetic testing. It can analyze specific genes or mutations, making it a powerful tool in prenatal screening, cancer diagnostics, and identifying genetic disorders.
"PCR has transformed the landscape of diagnostics by enabling the detection and quantification of nucleic acids in real-time, ensuring efficient patient care and management."
Forensic Analysis
Forensic applications of PCR are equally transformative. The technique has changed how forensic scientists analyze crime scene evidence, as trace amounts of biological samples can now yield sufficient DNA for analysis.
- DNA Profiling: PCR is regularly used in creating DNA profiles from blood, hair, skin, or other biological samples. In criminal investigations, these profiles can provide critical leads, link suspects to evidence, or exonerate the innocent.
- Cold Cases: With advancements in PCR techniques, cold cases can be re-evaluated using DNA evidence that was previously too degraded for analysis. This capability can bring justice even decades after a crime.
- Family Relationship Testing: PCR is also employed in paternity testing and other familial relationship examinations, providing necessary evidence in legal cases involving family law.
In summary, the applications of PCR in both medical diagnostics and forensic analysis underscore its importance as an indispensable tool in modern science. Its versatility not only aids in diagnosing diseases but also plays a critical role in the justice system, demonstrating the far-reaching impacts of this technique in various areas of research and practice.
Comparative Analysis: Nucleic Acid Tests vs PCR
The comparative analysis of nucleic acid tests and polymerase chain reaction (PCR) methods is crucial for understanding their respective roles within the field of molecular diagnostics. Each method presents unique strengths and limitations that impact their use in various applications, particularly in the diagnostics of infectious diseases and genetic conditions. An in-depth exploration of these aspects not only clarifies their importance but also helps professionals make informed decisions based on specific needs and contexts.
In this section, we will closely examine two key elements: efficacy and accuracy, as well as turnaround time and cost. This detailed analysis will provide insights into how these methods can be best utilized for optimal diagnostic outcomes.
Efficacy and Accuracy
Efficacy and accuracy are fundamental to establishing the reliability of any diagnostic test. Nucleic acid tests offer a high level of sensitivity, allowing for the detection of minute quantities of nucleic acids from pathogens or genetic material. This high sensitivity is especially important for identifying organisms that may be present in low numbers, such as in early stages of infection.
On the other hand, PCR’s robustness against contamination and its ability to amplify trace amounts of DNA make it widely regarded as a gold standard in many laboratories. The specificity of PCR is enhanced through the use of specific primers that bind only to target sequences. However, this method is not without its challenges. Factors such as incorrect primer design or sample handling can lead to significant false-positive and false-negative results, which undermines accuracy.
"The reliability of a test is directly correlated to the efficacy and the accuracy achieved in real-world applications."
In comparison, nucleic acid tests such as LAMP (Loop-Mediated Isothermal Amplification) have gained attention for their ability to produce results without the need for sophisticated thermal cycling equipment. This method can be equally effective while being less prone to temperature fluctuations, hence potentially increasing accuracy in some settings.
Turnaround Time and Cost
Turnaround time and cost are essential considerations in choosing between nucleic acid testing methods and PCR. PCR, while highly sensitive and accurate, often requires extensive preparation time due to its multi-step process. This includes sample collection, nucleic acid extraction, and the amplification steps. Consequently, the entire process may take several hours to days before results are reported.
Nucleic acid tests can offer a more rapid turnaround. Tests like rapid antigen tests can return results within minutes. However, their efficacy can sometimes be compromised. The balance between speed and sensitivity is often a deciding factor in various clinical scenarios.
Cost is another layer that often plays a critical role in the decision-making process. The reagents and equipment for PCR can be expensive, requiring notable investment from laboratories. Meanwhile, certain nucleic acid tests are designed to be more cost-effective, making them accessible in settings where budget constraints are a significant concern.
Limitations of Nucleic Acid Tests
Nucleic acid tests (NATs) play a pivotal role in modern diagnostics, particularly in disease detection and management. However, despite their significant benefits, these tests also come with limitations that cannot be overlooked. Understanding the constraints of nucleic acid tests is crucial not only for researchers but also for healthcare professionals who rely on these methods for accurate diagnostics. By examining these limitations, we can better assess the appropriateness of NATs for specific diagnostic scenarios, leading to more effective and informed healthcare decisions.
Sensitivity and Specificity Issues
The effectiveness of any diagnostic test largely hinges on its sensitivity and specificity. Sensitivity refers to the test's ability to correctly identify those with the disease, while specificity indicates its capability to correctly identify those without the disease. Nucleic acid tests, while often highly sensitive, can sometimes yield false negatives, particularly in the early stages of infection.
Several factors influence the sensitivity of NATs:
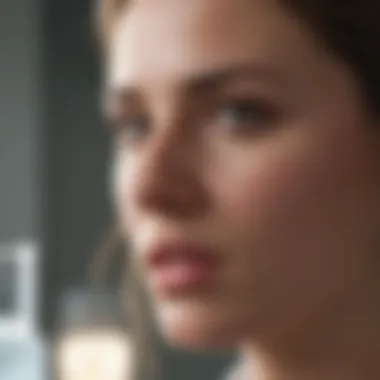
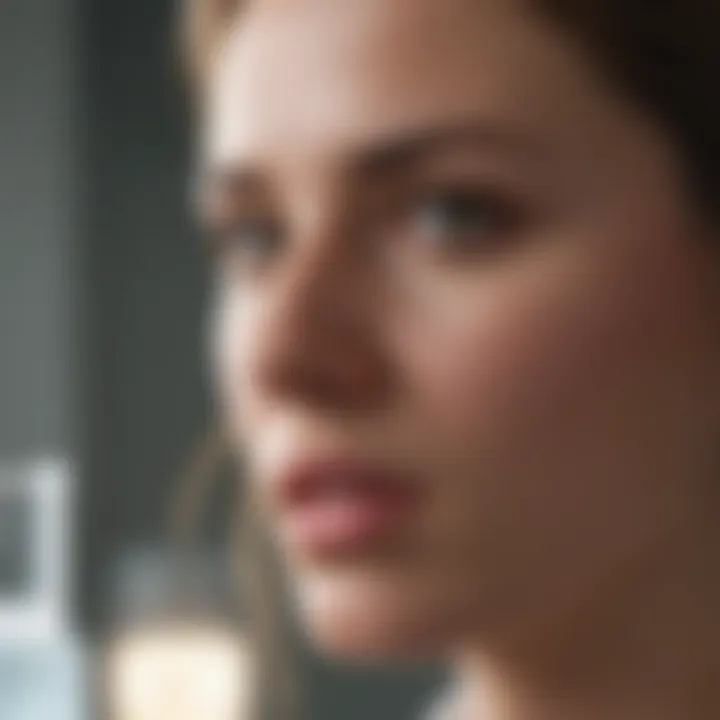
- Timing of Sample Collection: Testing too soon after exposure can result in negative results, even if the pathogen is present. This is particularly common in viral infections like HIV.
- Quality of the Sample: Poor sample quality may hinder detection of nucleic acids, leading to inaccuracies.
- Assay Variability: Different NATs have varying performance characteristics. It is essential to select the right test for the specific pathogen in question.
On the other hand, specificity can also be impacted. Contamination in the laboratory or during sample collection can lead to false positives. This can mislead diagnosis and treatment, undermining the credibility of nucleic acid tests in critical situations.
Sample Contamination Risks
Sample contamination is another critical limitation of nucleic acid testing. Given that these tests rely on amplifying specific genetic material, even trace amounts of extraneous nucleic acids can interfere with results. Contamination may occur at various stages, including:
- Collection: Inadequate sterile techniques during sample collection can introduce foreign DNA or RNA.
- Transportation and Handling: Samples that are not properly sealed or maintained can be exposed to environmental nucleic acids, leading to contamination.
- Laboratory Procedures: The processes involved in extracting and amplifying nucleic acids are sensitive to contamination risks. Cross-contamination between samples is a well-documented issue that can compromise test integrity.
Regular audits of laboratory protocols and strict adherence to contamination prevention measures are critical in minimizing these risks.
To mitigate these issues, laboratories often implement rigorous quality control measures and utilize advanced techniques for detection and quantification, such as using strict cleaning protocols and physical barriers.
Recognizing and addressing these limitations improves the reliability of nucleic acid tests in various diagnostic settings. As the landscape of molecular diagnostics continues to evolve, it is imperative to refine these tests to ensure their robustness and accuracy.
Limitations of PCR
Polymerase Chain Reaction (PCR) is a revolutionary technique in molecular biology that allows for the amplification of specific DNA sequences. Despite its many applications and successes, it also has several limitations that researchers and practitioners must consider. Understanding these limitations is crucial for accurate data interpretation and making informed decisions in both research and clinical settings.
DNA Quality Concerns
One of the key limitations of PCR is its reliance on the quality of the DNA sample. High-quality DNA is essential for effective amplification. If the DNA is degraded or contaminated, it can lead to suboptimal results. For instance, samples that have been improperly stored or exposed to extreme conditions may contain fragments rather than intact DNA molecules. This degradation affects the specificity and efficiency of the amplification process, often resulting in incomplete or non-specific products.
The initial quantity of target DNA also plays a significant role. In cases where the target is present in low quantities, it may be difficult to achieve successful amplification simply due to insufficient starting material. Therefore, ensuring the integrity of the sample and assessing its quality before starting the PCR process is vital.
False Positives and Negatives
Another significant concern with PCR is the occurrence of false positives and false negatives. A false positive occurs when the test indicates that a target sequence is present when it is not. This can happen due to contamination during any stage, such as sample collection or handling, especially if proper sterile techniques are not utilized. Cross-contamination between samples can lead to erroneous results, which can severely impact clinical decisions.
Conversely, a false negative result indicates that a target sequence is absent when it is actually present. This situation can arise when the amplification conditions are not optimal or if the PCR protocol is not adequately sensitive. Additionally, inhibitors present in the sample can interfere with the PCR reaction, leading to failure in amplifying the desired DNA sequence.
Understanding these limitations is essential for healthcare professionals and researchers. Awareness of these factors can guide necessary adjustments to procedures and improve the reliability of PCR results.
"The effectiveness of PCR is intimately connected to the quality of the starting material and the precautions taken to avoid contamination."
Future Directions in Nucleic Acid Testing and PCR
The future of nucleic acid testing and polymerase chain reaction (PCR) is a subject of significant interest and importance. As the world leans more heavily into personalized medicine, understanding how these technologies can evolve to meet new challenges is essential. Advances in these methodologies will shape the landscape of diagnostics in the medical field, enabling quicker, more reliable, and more accurate results.
One important aspect to consider is the ongoing innovations in technology. Emerging methods such as CRISPR-based diagnostics are at the forefront, promising enhanced sensitivity and specificity. These innovations not only aim to improve laboratory efficiency but also expand possibilities for point-of-care testing. This shift could democratize access to diagnostics, especially in low-resource settings.
Innovations and Emerging Technologies
Innovations in nucleic acid testing and PCR are increasingly focused on several key areas:
- Miniaturization: Development of microfluidic devices allows for multiplexing and testing multiple pathogens from a single sample, significantly reducing time and cost.
- CRISPR Technology: This gene-editing tool is being integrated into diagnostic platforms, offering rapid and precise analyses. CRISPR-based tests can detect viral RNA within hours, showcasing potential in outbreak management.
- Next-Generation Sequencing (NGS): NGS enables comprehensive genomic profiling. Its integration with nucleic acid testing provides deeper insights into genetic diseases and infectious pathogens, opening pathways for targeted therapies.
- Digital PCR: This technology enhances accuracy through partitioning samples, allowing for the detection of low-abundance targets that traditional PCR methods might miss.
As more research continues, it is likely we will see partnership between academic institutions and industry, leading to even more breakthroughs in testing technologies.
Regulatory and Ethical Considerations
The rapid evolution of nucleic acid testing and PCR necessitates careful consideration of regulatory and ethical implications. Regulatory bodies must establish clear guidelines to ensure safety and efficacy in these novel testing methods. Some areas needing attention include:
- Quality Assurance: Establishing protocols for testing procedures will be critical in maintaining standards across laboratories, especially with the rise of rapid testing technologies.
- Data Privacy: As genetic testing becomes more widespread, safeguarding patient data will be paramount. Ethical frameworks must be implemented to protect sensitive information.
- Equity of Access: There is a risk that advanced technologies may only be available in more affluent regions. Addressing this disparity is vital for equitable healthcare.
- Informed Consent: Patients must fully understand the implications of nucleic acid testing. Ethical considerations regarding how results are communicated and used should form the basis of informed consent processes.
"The integration of emerging technologies into nucleic acid testing is revolutionizing diagnostics, but it must be guided by strict ethical and regulatory frameworks to ensure public trust and safety."
Finale
The conclusion serves as a crucial element of this article as it encapsulates the central themes and findings discussed throughout the text. The analysis of nucleic acid tests in comparison to polymerase chain reaction (PCR) methods contributes to a wider understanding of their respective roles in diagnostics, particularly within the realms of infectious diseases and genetic research. In light of recent advancements in molecular diagnostics, grasping the distinctions between these techniques is essential for healthcare practitioners, researchers, and students alike.
Summary of Key Findings
The examination highlights several key findings:
- Nucleic acid tests and PCR are both fundamental in molecular diagnostics, yet they function through distinct mechanisms.
- While nucleic acid tests offer diverse methodologies like real-time PCR and LAMP, PCR remains a gold standard for many diagnostic applications.
- Both techniques exhibit advantages in terms of sensitivity and speed but come with limitations related to sample quality and potential contamination.
These insights clarify not only how each method operates but also their relative efficacy in various contexts. By understanding the solo and combined strengths of these techniques, practitioners can better inform clinical decisions and enhance patient outcomes.
The Role of Molecular Diagnostics in Future Healthcare
Molecular diagnostics is set to play a pivotal role in shaping future healthcare strategies. The growth of personalized medicine, for instance, relies heavily on insights derived from both nucleic acid applications and PCR methodologies.
- Early Detection: Enhanced testing methods can allow for earlier detection of diseases, especially ones that are genetic or infectious in nature.
- Tailored Treatments: A clearer understanding of genetic profiles means treatments can be customized to individual needs, increasing their effectiveness.
- Public Health Initiatives: Nucleic acid testing can aid in monitoring outbreaks and developing rapid responses to emerging health threats.
Overall, the future landscape of healthcare is likely to be characterized by the integration of these tests into routine clinical practice, leading to improved diagnostic precision and patient care. Embracing these innovations is beneficial not just for practitioners, but for the healthcare system as a whole.