Comprehensive Guide to Particle Size Distribution Methods
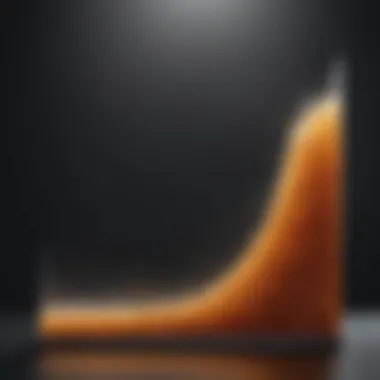
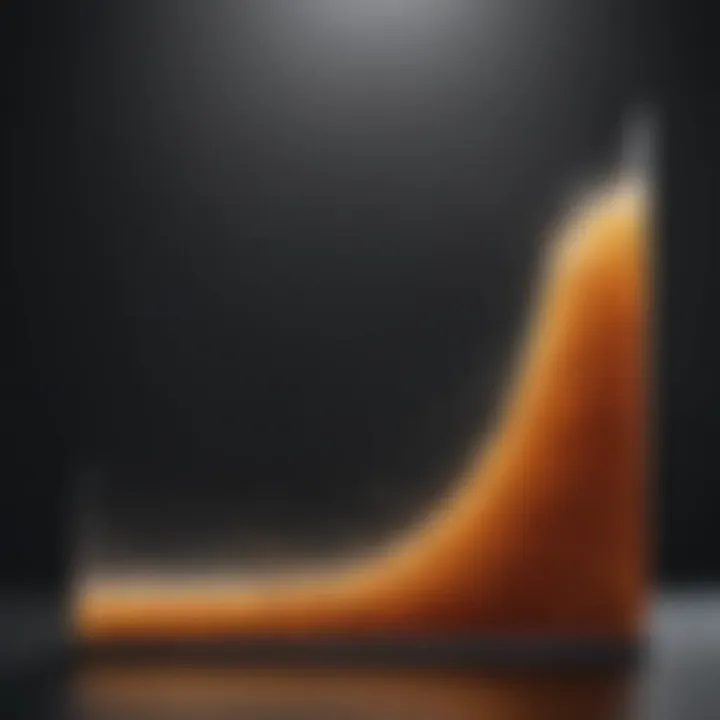
Intro
Particle size distribution (PSD) is a crucial aspect of material characterization across multiple scientific fields. Understanding the size and distribution of particles can have significant implications for various processes, from pharmaceuticals to environmental sciences. This article takes an informed approach to investigate various methods of PSD analysis, providing insights that are both rich and informative.
The attraction of particle size lies in its influence on physical and chemical properties. Factors such as reactivity, solubility, and flowability are often linked to particle size. Therefore, an analytical perspective on PSD is paramount for researchers, professionals, and educators.
We will delve into traditional methods like sieving and sedimentation, as well as modern techniques such as laser diffraction and dynamic light scattering. This exploration not only highlights the depth and variety of methodologies available but also addresses their respective advantages and limitations. Across the sections ahead, we will synthesize the existing knowledge and examine the relevance of effective PSD analysis in scientific advancements and applications.
As we embark on this journey, our aim is to equip you with a thorough understanding, catering to those who seek to enhance their analytical repertoire in particle size distribution.
Prelude to Particle Size Distribution
In the realm of material characterization, the analysis of particle size distribution (PSD) is pivotal. It affects the behavior, performance, and processing of materials across diverse applications. Understanding PSD is not merely an academic exercise; it has real-world implications in fields such as pharmaceuticals, material science, and environmental studies.
The significance of PSD derives from its multifaceted impact. For instance, in pharmaceuticals, drugs' efficacy often hinges on their particle sizes. Smaller particles may enhance absorption rates in the body, influencing bioavailability. Thus, a thorough grasp of PSD is essential for scientists and engineers alike.
Moreover, the methods used to assess PSD can be varied. They can range from simple techniques like sieving to more sophisticated procedures like laser diffraction. Each method comes with its own set of benefits and considerations, which will be further explored in subsequent sections of this article. This comprehensive exploration of PSD will provide professionals and enthusiasts in the scientific community with invaluable insights into the methodologies and applications relevant to their fields.
Definition of Particle Size Distribution
Particle Size Distribution refers to the measurement and quantification of the sizes of particles within a particular sample. It offers critical insights into the characteristics and behavior of materials. The distribution is often expressed in terms of various parameters, including the mean, median, and standard deviation of particle sizes.
Fundamentally, PSD can be depicted in graphical form, typically as a cumulative distribution curve or a histogram. This representation allows for a visual interpretation of the particle size spectrum, which is vital for understanding how the physical properties of a material will behave.
Importance of Particle Size in Various Fields
In numerous disciplines, the role of particle size is profound. For instance:
- Material Science: The properties of composite materials often rely on the distribution of particle sizes. Uniformity in size can increase material strength and stability.
- Pharmaceuticals: The efficacy of drug formulations is tightly linked to particle size. Smaller particles can improve dissolution rates and absorption, making them more effective in medical applications.
- Environmental Studies: In areas such as soil analysis or air quality testing, the size of particulate matter directly relates to health and environmental impact. Understanding the distribution helps in assessing contamination and its control measures.
"Particle size can drastically influence both performance and functionality across various fields. A deviation in size can lead to significant inconsistencies in results."
In summary, mastering PSD analysis is critical to ensuring optimal performance across various industries. The subsequent sections will delve deeper into the techniques used for measuring particle size, their accuracy, and their application across different fields.
Fundamental Concepts in Particle Size Analysis
Understanding the fundamental concepts of particle size analysis is vital for advancing both research and practical applications across various disciplines. This understanding lays the groundwork for accurately assessing materials, which is essential for ensuring quality and performance in products ranging from pharmaceuticals to advanced materials. It informs selection of appropriate measurement techniques, consideration of statistical representations, and interpretation of results. Moreover, grasping these principles aids in addressing challenges that arise in diverse fields, allowing for informed decision-making that can enhance both innovation and efficiency.
Measurement Techniques Overview
Particle size analysis employs various measurement techniques, each with its unique principles and operational protocols. The selection of a technique can deeply affect the outcomes of the analysis, making it crucial to understand the strengths and limitations of each method. Common techniques include:
- Sieving: This is one of the oldest and simplest methods, where particles are classified by passing them through a series of sieves with different pore sizes. It is effective for larger particles but less appropriate for very fine materials.
- Sedimentation: This method relies on the gravitational settling of particles in a liquid, applying Stokes' Law to calculate the size based on the rate of settling. It is useful for an understanding of size distribution in slurries or colloidal solutions but may be limited by particle shape and density variations.
- Laser Diffraction: In this advanced method, light scattering from particles in a laser beam is analyzed to determine size distribution. It is quick and can handle a wide range of sizes, but may require calibration and periodic instrument checks to maintain accuracy.
- Dynamic Light Scattering (DLS): This technique measures the Brownian motion of particles to determine their size. It is particularly effective for nanoparticles and emulsions, however, factors such as concentration and sample purity can influence accuracy.
Each of these techniques has its distinct applications and is chosen based on the specific requirements of the study being conducted.
Statistical Representation of Particle Size
Statistical representation is a fundamental aspect of particle size analysis, allowing for meaningful interpretation of data from various measurement techniques. The representation methods can include:
- Mean Size: A simple average that provides a quick overview of the particle size but can be misleading if the distribution is skewed due to outliers.
- Median Size: Represents the middle value in the data set, offering a more robust measure that minimizes the influence of extreme values.
- Standard Deviation: This gives insight into the variability of particle sizes, indicating how consistent or dispersed the particles are around the average size.
- Size Distribution Curve: Visual representation of particle sizes, typically displayed as a histogram or cumulative distribution function, allowing for a clearer understanding of the distribution pattern.
Accurate statistical representation is key for determining the impact of particle size on material properties, and it plays a major role in quality control and product effectiveness.
The choice of statistical methods should be based on the goals of the analysis and the nature of the data, ensuring that relevant features of particle size are captured effectively. Ultimately, this foundational understanding of measurement techniques and statistical representation informs more sophisticated applications and interpretations in various fields.
Traditional Methods of Analysis
Traditional methods of particle size distribution analysis hold a foundational role in the field. These techniques provide essential background and comparatives against modern methodologies. Understanding traditional techniques helps to appreciate their evolution and the contexts in which they are still applicable today.
The investigation of particle size distribution remains significant, notably because many industries still rely on these methods. By examining their benefits and considerations, we contribute to a nuanced understanding of the advancements in this area.
Sieving Techniques
Sieving is one of the oldest methods of particle size analysis and continues to be relevant today. It operates by passing particles through a series of screens with different mesh sizes. Each layer retains particles that exceed the mesh size, allowing for a straightforward classification process. This method is recognized for its simplicity and cost-effectiveness.
Types of Sieves
Sieves can come in various forms, including standard sieves, test sieves, and sonic sieves. Each type serves particular needs, depending on the granularity of the materials being analyzed.
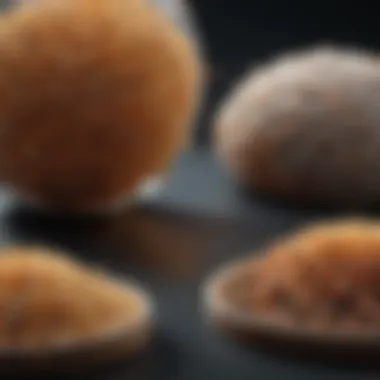
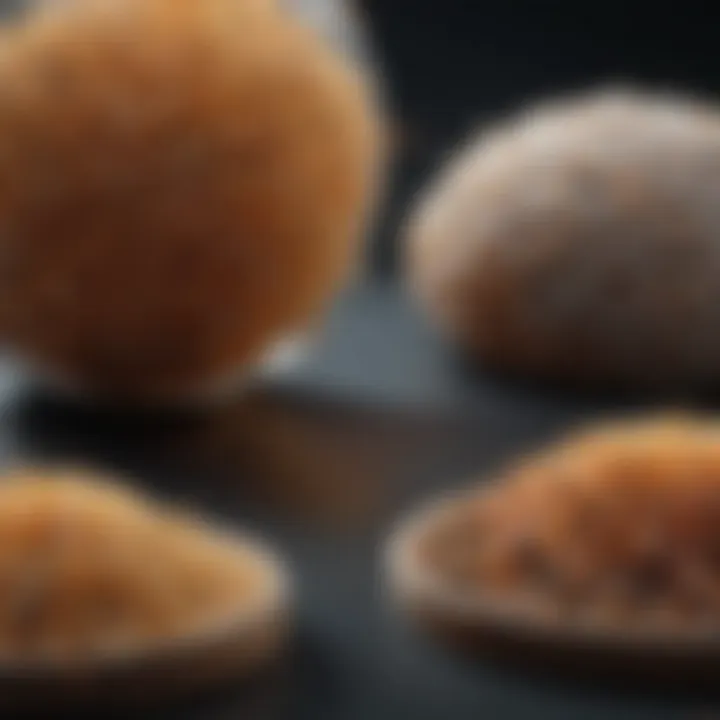
A key characteristic of sieves is that they facilitate the physical separation of particles without altering their structure. For instance, standard sieves made of woven wire mesh are widely used in laboratories due to their robustness. However, one unique feature of sonic sieves employs sound waves to assist in the separation process. This technology enhances the efficiency of particle classification. Despite their benefits, sieves may not be suitable for assessing extremely fine particles, limiting their application scope in certain scenarios.
Advantages of Sieving Techniques:
- Cost-effective: Traditional sieving techniques require minimal investment in equipment.
- Simple operation: Anyone with basic training can perform sieving.
- Immediate results: The method offers real-time classification.
However, non-desirable effects may occur, such as particle agglomeration or loss of fines.
Applications of Sieving
Applications of sieving are vast, ranging from construction to pharmaceuticals. In construction, sieving determines the appropriate mix of aggregates for concrete.
A key characteristic of applications is their adaptability across diverse industries. For example, the pharmaceutical sector uses sieving to ensure uniformity in tablet formulations.
Unique features of applications include the ability to combine sieving with other methods, enhancing analysis precision. Despite this, some issues can arise, such as limited accuracy in sizing for certain materials, which may be a concern, especially with very fine powders.
Sedimentation Techniques
Sedimentation is another classical technique that evaluates particle size based on the settling velocity of particles in a fluid medium. The principle here is that smaller particles settle more slowly than larger ones under the influence of gravity. This method is tied closely to fluid mechanics, providing a reliable overview of size distribution.
Stokes' Law Application
Stokes' Law applies to this technique by describing the motion of a sphere through a viscous fluid. It states that the settling velocity relates to the square of the radius of the particle, making it a vital equation for determining particle sizes.
Key characteristic of Stokes' Law is the direct proportionality of particle size and settling rate, allowing researchers to predict sizes based on known variables. It's a beneficial choice for layered analysis when examining sedimentation behavior in controlled environments.
However, the law assumes spherical particles and lacks accuracy for non-spherical or irregularly shaped particles.
Limitations of Sedimentation
While sedimentation is valuable, it possesses limitations. For example, particles with similar sizes can have vastly different settling velocities based on their shape or density.
A key characteristic of these limitations is that they may affect the clarity of results and lead to misrepresentation of size distribution.
Moreover, the method is influenced by external factors such as temperature and viscosity of the fluid, which could skew the findings. The sedimentation process often takes time, leading to delays in obtaining results.
Overall, traditional methods such as sieving and sedimentation have stood the test of time despite evolving technology. Their role in particle size distribution ensures that even as newer methods emerge, these techniques provide fundamental insights into the realm of particle analysis. This continued relevance invites further examination and refinement in various applications.
Advanced Analysis Techniques
Advanced particle size distribution analysis methods have become increasingly relevant in modern scientific research. These methods provide higher precision and can analyze a wider range of particle sizes than traditional techniques. Their importance lies primarily in their ability to deliver rapid, reproducible results and their adaptability across various fields from material science to pharmaceuticals.
Laser Diffraction
Principle of Operation
Laser diffraction is a widely accepted technique for measuring particle size distribution. This method operates on the principle that particles scatter light at angles that depend on their size. Smaller particles scatter light at larger angles, while larger particles scatter at smaller angles. The data collected from this scattering is analyzed using sophisticated algorithms which translate the scattering pattern into particle size distribution information.
One key characteristic of laser diffraction is its speed in data collection, typically offering results within minutes. This immediacy makes it a beneficial choice for labs that require fast turnaround times. Moreover, laser diffraction is capable of analyzing a broad size range, from submicron to several millimeters, which is essential in various applications.
However, one distinctive feature of laser diffraction is its dependency on assumptions regarding particle shape and refractive index. This can lead to inaccuracies in cases where particles deviate significantly from spherical shapes.
Applications and Accuracy
The applications of laser diffraction span numerous fields. It is used for characterizing powders in pharmaceuticals, ensuring consistency in active ingredients. In material science, it aids in designing composites by determining the proper particle sizes for optimal material performance.
The accuracy of laser diffraction is another key highlight. It provides high-resolution data that can be crucial for quality control and product development in many industries. Nonetheless, results can vary based on factors like the dispersion medium used and particle agglomeration. This requires careful sample preparation to maintain accuracy, which can sometimes pose a challenge in practical scenarios.
Dynamic Light Scattering (DLS)
Mechanism of DLS
Dynamic Light Scattering presents a different methodology for the analysis of particle sizes, primarily focusing on smaller particles in suspension. It operates on the principle of Brownian motion where particles in a fluid are subjected to random thermal motion. By shining a laser through a sample, DLS measures the fluctuations in intensity caused by the movement of these particles. This data allows the calculation of the size of the particles based on how quickly they move in the medium.
A key characteristic of DLS is its ability to provide detailed information on nanoparticles, which is particularly valuable in nanotechnology and pharmaceuticals.
However, a unique aspect of DLS is its limitation to relatively small particles, usually below one micron. While this restriction is a disadvantage for broader applications, it makes DLS highly effective where precision with small sizes is critical.
Advantages Over Other Techniques
DLS is known for its accessibility and ease of use compared to other particle sizing methods. It requires minimal sample preparation, making it suitable for laboratory environments with limited resources. This is a practical advantage over techniques that necessitate more complex setups.

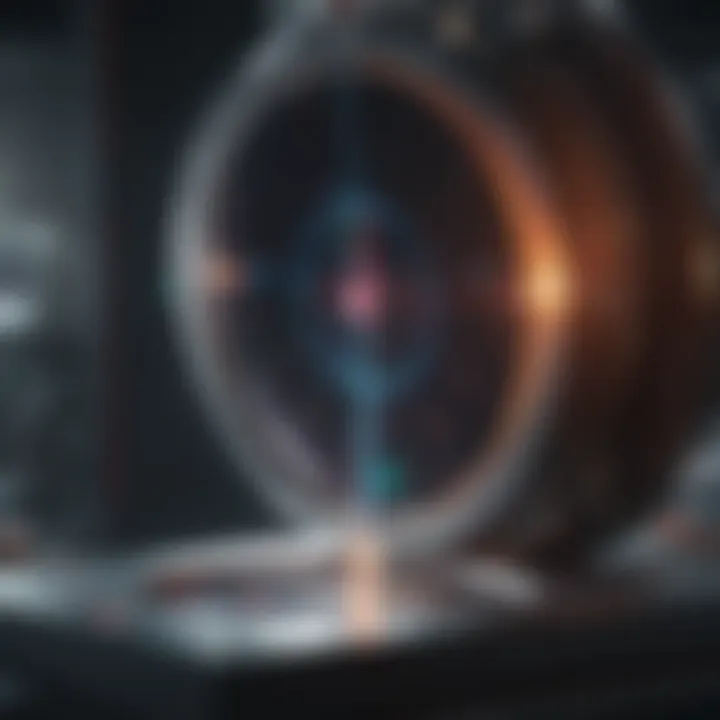
The ability of DLS to analyze very low concentrations of particles makes it a preferred choice for many industries. Yet, it also has its drawbacks. For instance, larger particles and aggregates can give misleading results due to their influence on scattering patterns. This means proper calibration and interpretation are vital to avoid inaccuracies in particle size assessment.
Comparison of Methods
In particle size distribution analysis, the comparison of methods is crucial. With a variety of techniques available, selecting the right one is essential for obtaining reliable results. Different methods can yield different particle size distributions, making this comparison vital for ensuring accuracy and relevance in research outcomes.
When choosing a method, researchers must consider several elements:
- Data requirements: Some methods may require more detailed input or information than others.
- Sample characteristics: The nature of the sample can influence what method is most appropriate.
- Measurement range: Different techniques may offer varied ranges of particle size detection.
Overall, the importance of comparing these methods cannot be overstated. It allows professionals to weigh the benefits and limitations of each approach in relation to their specific needs.
Accuracy and Precision
Accuracy and precision are critical in particle size distribution analysis. Accuracy refers to how close a measurement is to the true value, while precision measures the repeatability of the method, or how consistently it yields the same results under unchanged conditions.
Different methods exhibit varied levels of accuracy and precision. For instance, laser diffraction is recognized for high precision in a range of particle sizes, while sedimentation techniques might be less precise due to external influencing factors like temperature or sample viscosity. Recognizing these differences is vital for interpreting data correctly.
Factors affecting accuracy include:
- Quality of instrumentation used
- Calibration procedures followed
- Environmental conditions during analysis
Thus, when assessing methods, accuracy and precision should be major considerations. Ensuring both are prioritized will ultimately lead to more reliable and interpretable results.
Cost and Accessibility
Cost and accessibility of measurement techniques are important considerations. Some advanced methods like laser diffraction are costly and may not be accessible to smaller labs or educational institutions. Conversely, traditional methods such as sieving and sedimentation tend to be more affordable and easier to implement.
Yet, while cost may be a limiting factor, it should not limit the choice of methodology if accuracy and appropriateness are at stake. Cost-effective options exist that deliver reliable results, especially for basic particle size analysis. It's crucial to find a balance between cost, accuracy, and the specific needs of the research being conducted.
Applications of Particle Size Distribution Analysis
The analysis of particle size distribution has a critical role in various industries. Understanding how particles are distributed in size can influence the selection of materials, the effectiveness of products, and the overall quality of outcomes in research and applications. Techniques for measuring particle size can help engineers create better materials, chemists to formulate effective drugs, and environmental scientists to evaluate soil and air quality. This section reviews several fulfilling applications across key fields: material science, pharmaceuticals, and environmental studies.
Material Science
Nanoparticle Characterization
Nanoparticle characterization is essential in nanotechnology. Here, the size and distribution of nanoparticles play a pivotal role in determining their physical and chemical behaviors. Smaller particles often exhibit different properties than their larger counterparts, which can affect their reactivity and stability. One prominent reason for the importance of nanoparticle characterization is its direct effect on performance in applications such as catalysis, drug delivery, and electronics.
The key characteristic of this analysis is its ability to provide insight into the shape and surface area of nanoparticles. Such details help researchers understand the interaction of nanoparticles with their environment. A major advantage of focusing on nanoparticle size is that it allows for tailoring properties for specific applications, leading to more effective and safer products.
However, challenges also exist in nanoparticle characterization. The methods may require significant preparation and optimization of samples to ensure that the readings accurately reflect the typical particle size of the material being tested.
Composite Materials
Composite materials often consist of two or more constituents to achieve desired mechanical properties. Particle size distribution analysis reveals how different sizes of particles in composites interact with each other. For instance, the distribution can influence the strength, toughness, and durability of materials used in construction and manufacturing.
A beneficial characteristic of studying composite materials is that researchers can predict performance based on the size distribution of the components. This helps in optimizing formulations and achieving better results in applications, such as aerospace and automotive industries. Its unique feature is how the right mixture and sizes can greatly enhance the overall performance of the end product.
Nonetheless, different particle sizes may lead to challenges in homogeneity in composites. Achieving a consistent particle size distribution can require careful control during the manufacturing process.
Pharmaceuticals
Drug Formulations
In pharmaceuticals, drug formulations depend heavily on particle size distribution to optimize quality and effectiveness. The size of active pharmaceutical ingredients (APIs) can impact solubility, stability, and absorption rates in the body. This can make particle size analysis crucial for effective drug development.
The key trait of drug formulations is their tailored release profiles, which can be influenced by particle size. For example, smaller particles can dissolve faster, improving bioavailability and ensuring that drugs work faster in the body. This feature makes particle size determination a popular choice for formulating effective medications.
On the downside, too small a size can lead to aggregation and stability issues, impacting the overall performance and storage of drug products.
Bioavailability Factors
Bioavailability factors relate to the proportion of a drug that enters circulation when introduced into the body. The size and distribution of particles affect how drugs are absorbed. Small particles often lead to higher bioavailability, as they have larger surface areas in contact with bodily fluids, which consequently leads to faster dissolving.
Highlighting this key characteristic is how understanding size and distribution can lead to formulations that maximize therapeutic effects with minimum doses, benefiting patient outcomes. However, particular formulations can encounter manufacturing errors due to sensitive methods of size distribution control.
Environmental Studies
Soil Analysis
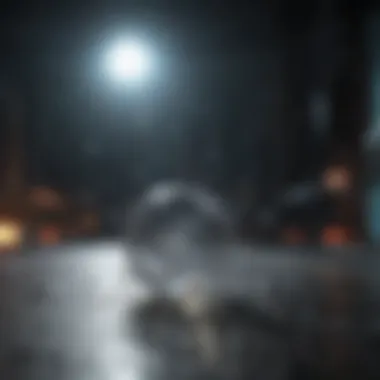
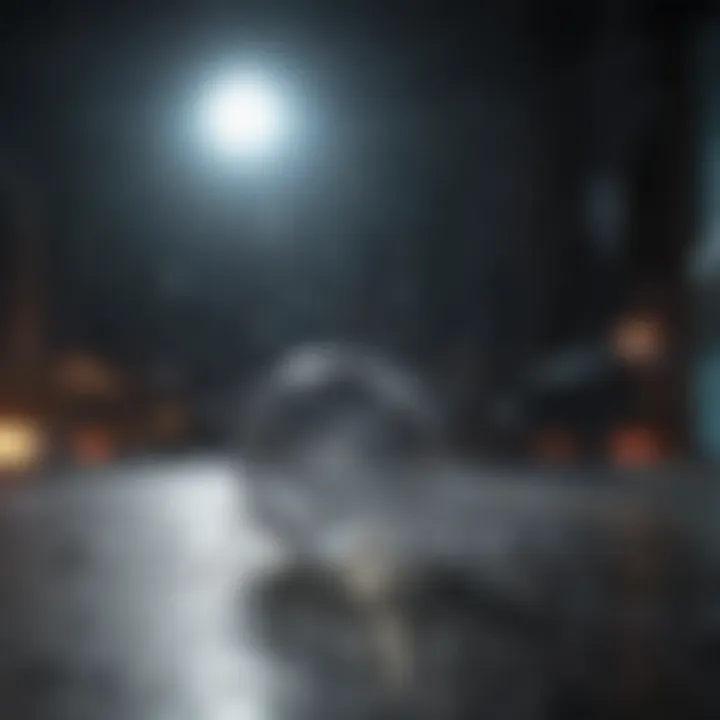
In environmental science, soil analysis focuses on understanding particle size regarding soil composition and behavior. It helps in assessing soil fertility, erosion potential, and water retention properties. The distribution of different particle sizes can indicate soil quality and its implications for agricultural practices.
A key aspect of soil analysis is its role in land management and conservation efforts. Accurate particle size distribution allows for better-informed decisions on soil treatment and crop selection. While advantageous, proper sampling methods are essential to ensure representative results, and that can complicate the analysis process.
Air Quality Assessment
Air quality assessment involves analyzing particulate matter in the environment. Particle size distribution helps identify sources of pollution and its potential health impacts. Smaller particles can penetrate deeper into the respiratory system, leading to severe health issues.
The unique feature of assessing particle size in air studies is its relevance for regulatory standards on pollution control. Monitoring particle size is crucial for compliance, yet limitations in real-time monitoring can pose challenges.
A comprehensive understanding of particle size distribution across applications contributes to significant advancements in material engineering, medicine, and environmental management.
Limitations and Challenges
Particle size distribution analysis methods play a key role in various scientific disciplines. However, these methods are not without limitations and challenges. Understanding these issues is crucial for achieving accurate results. This section explores the specific elements that limit the effectiveness of particle size analysis, focusing on sample preparation issues and reproducibility concerns.
Sample Preparation Issues
Sample preparation is often the first step in particle size analysis, and it significantly influences the outcome. Proper preparation aims to ensure that the sample accurately represents the whole batch. Poorly prepared samples can lead to skewed data, which can misguide research conclusions.
Several factors can complicate sample preparation:
- Contamination: Samples may become contaminated during collection, handling, or storage. This contamination can alter the measured particle size.
- Aggregation: Fine particles may agglomerate, leading to larger perceived sizes. This issue is particularly prevalent in wet processes.
- Environmental Conditions: Humidity and temperature can affect sample stability. If not controlled, these conditions can impact particle behavior.
All these variables highlight the need for stringent protocols in sample preparation. Researchers must be aware that even small errors in this stage can compromise the integrity of their analysis.
Reproducibility Concerns
Reproducibility is a cornerstone of scientific research. The ability to replicate results validates findings and strengthens confidence in methodologies. However, particle size distribution analysis faces several reproducibility challenges.
Factors affecting reproducibility include:
- Operator Variability: Different technicians may employ slightly different techniques, leading to variations in results.
- Instrument Calibration: Equipment used for measurements must be properly calibrated. Even minor deviations can introduce errors.
- Method Sensitivity: Certain analysis techniques may be sensitive to environmental variations, such as vibrations or electromagnetic fields, possibly leading to inconsistent results.
Achieving reproducible results is essential for maintaining credibility in scientific research.
Therefore, addressing these challenges requires systematic approaches. Researchers should standardize methods and document their processes thoroughly to facilitate better replicability across studies. Careful attention to both sample preparation and method execution can ultimately enhance the reliability of particle size distribution analyses.
Future Directions in Particle Size Research
The field of particle size distribution analysis is rapidly evolving. As technology advances, new possibilities emerge that transform how we understand, measure, and analyze particle characteristics. To stay relevant and effective, researchers must be aware of these future directions in particle size research. Emerging technologies and innovative applications hold the potential to refine current methodologies and expand their utility across different sectors.
Emerging Technologies
Advanced imaging techniques are at the forefront of improving particle size analysis. Methods like scanning electron microscopy (SEM) and transmission electron microscopy (TEM) provide high-resolution images at the nanoscale. This accuracy allows for more precise measurements of particle size and morphology.
Additionally, machine-learning algorithms are becoming pivotal. They can analyze vast datasets and identify patterns that human analysts might overlook. For instance, researchers can now use these algorithms to predict the behavior of particles based on size, shape, and distribution. This predictive capability can save time and resources in laboratory settings.
Moreover, instruments like image-based analyzers are gaining traction. These devices can measure particle sizes quickly and accurately through digital imaging. They offer extensive data collection capabilities, which is beneficial for detailed statistical analysis.
Potential for Novel Applications
The importance of particle size distribution is not limited to traditional fields. Novel applications are emerging in areas like nanotechnology, where controlling particle size is crucial for developing new materials. For example, nanoparticles are being explored for targeted drug delivery in pharmaceuticals. Understanding their size distribution can determine how effectively they reach the intended site in the body and how they interact with biological systems.
In environmental science, studying the size distribution of particulate matter can aid in assessing air quality. Smaller particles are more likely to penetrate deep into the lungs and cause health issues. As regulations around air quality tighten, accurate particle size analysis will become even more critical.
The food industry is also taking interest in particle size distribution. In processes such as emulsification and milling, optimizing the size of food particles can improve product texture and shelf life. Addressing these challenges can lead to innovations that enhance safety and consumer satisfaction.
The evolution of particle size distribution analysis is crucial for diverse fields. As challenges arise, exploring these technologies and applications presents significant oppertunities and benefits to science and industry.
End
In the realm of particle size distribution analysis, the conclusion serves an essential purpose. It synthesizes the information presented throughout the article, tying together various themes and methods explored. This section plays a critical role in reinforcing the significance of understanding particle size distribution in multiple fields, such as material science, pharmaceuticals, and environmental studies.
Summary of Key Points
The key points discussed in this article are pivotal for anyone engaged in the study or application of particle size analysis. Key aspects include:
- Definition: Clear understanding of what particle size distribution is.
- Measurement Techniques: Overview of both traditional and advanced methods for analysis, including sieving, sedimentation, laser diffraction, and dynamic light scattering.
- Applications: Importance in diverse areas like material science, pharmaceuticals, and environmental monitoring.
- Limitations: Awareness of the challenges and limitations that exist within these methodologies, which can affect accuracy and reproducibility.
By summarizing these elements, readers can appreciate the breadth and depth of the topic.
Implications for Future Research
The implications for future research are vast and significant. Trends indicate that emerging technologies continue to advance particle size distribution analysis. Researchers should focus on:
- Integration of Artificial Intelligence: Utilizing AI to enhance data analysis and interpretation can lead to faster and more accurate particle size assessments.
- Innovative Techniques: Exploration of novel methodologies that could address existing limitations in traditional methods.
- Interdisciplinary Research: Encouragement of collaboration across fields, such as combining insights from nanotechnology and environmental science to improve measurement techniques.