Plasmid Isolation Techniques and Their Applications
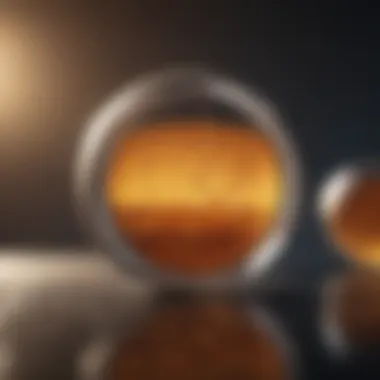
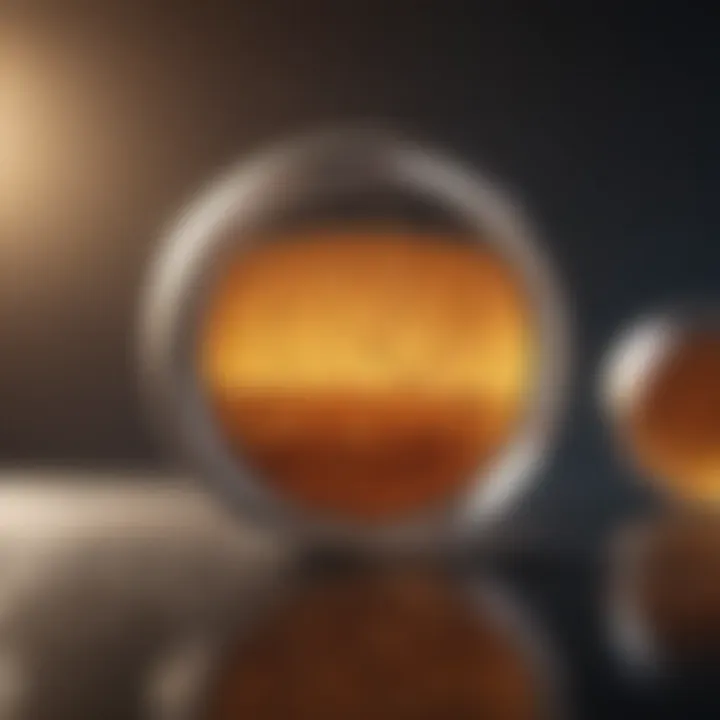
Intro
Bacterial plasmids, those small, double-stranded DNA molecules, are more than just genetic fluff hanging around in microbial cells. They play a crucial role in the genetics of bacteria. Understanding how to isolate these plasmids is vital for many fields, including biotechnology and genetic research. Various techniques exist for plasmid isolation, with each having its strengths and weaknesses. Knowing the right method for your needs can make a significant difference, especially when the stakes are high.
Think of plasmids as the little black dress of molecular genetics—flexible, adaptable, and often essential for creating novel functions in bacterial strains. Whether it’s for developing new antibiotics, engineering modified organisms, or studying gene therapy applications, plasmid extraction is indispensable.
In this article, we’ll dissect the techniques for isolating plasmids, examine their applications in research, and highlight the implications of these genetic elements in the broader scope of microbial genetics.
Research Highlights
Overview of Key Findings
- Plasmid Significance: Plasmids contribute actively to the adaptability of bacteria, particularly in environments rich in selective pressures like antibiotic presence.
- Isolation Techniques: The two most widely used methods—alkaline lysis and centrifugation—are detailed, shedding light on their operational processes and practical implications.
- Applications: The implications of plasmid isolation stretch into the realms of antibiotic resistance research and the development of gene therapy techniques.
Significance of the Research
Bacterial plasmids serve as vectors for understanding genetic engineering and synthetic biology. Their isolation not only paves the way for more efficient research methodologies but also enhances the practical utility of genetically engineered bacteria in various industries.
A granular analysis of complex interactions between plasmids and bacterial genomes reveals a lot about microbial adaptability and resistance mechanisms, making this field of study crucial for informed medical, agricultural, and environmental practices.
"Understanding how plasmids function equips researchers with the necessary tools to innovate in areas like antibiotic development and genetic modifications."
Original Research Articles
Summary of the Article
By dissecting the methods employed in plasmid isolation, we gain insights into the diverse approaches researchers and labs have taken. The article highlights major techniques and evaluates them based on efficiency, reproducibility, and cost.
Author Contributions
Various authors contributed their expertise, bringing different perspectives from fields like microbiology, genetics, and biotechnology. Their collective efforts help crystallize a broad understanding of plasmid isolation and its applications across multiple domains.
Research in this area remains dynamic, continuously evolving as new techniques emerge and existing ones are refined, highlighting the importance of keeping abreast of the latest methodologies.
Prolusion to Plasmids
In the realm of molecular biology, plasmids have carved out a significant niche, serving as more than just random bits of DNA. They play essential roles in a multitude of biological processes and applications, particularly in genetic engineering and biotechnology. Understanding these small, circular DNA molecules can illuminate how bacteria adapt and survive in various environments, making their study crucial for both academic and practical pursuits.
Plasmids are often used as vectors in gene cloning and synthetic biology. They facilitate the transfer of genetic material, thereby enabling scientists to manipulate genes for research and therapeutic purposes. This adaptability makes plasmids indispensable tools in laboratories, driving forward the fields of microbiology, pharmacology, and agricultural biotechnology.
As we dive further into this exploration of plasmids, we will unpack their definitions, types, and overall impact on bacterial functioning. By shedding light on these genetic elements, we can appreciate their vital contributions to advancements in science and technology.
Definition of Plasmids
Plasmids are extrachromosomal DNA molecules found primarily in bacteria, although some eukaryotic organisms harbor them as well. These entities can replicate independently of the chromosomal DNA, allowing them to be passed on during cell division. Generally, plasmids carry genes that may benefit the host organism, contributing to its survival in challenging environments. For instance, genes that offer antibiotic resistance or confer metabolic advantages are common in plasmids.
In essence, plasmids represent a remarkable aspect of microbial genetics, showcasing how nature leverages the flexibility of DNA to engineer evolutionary fitness.
Types of Plasmids
When delving into plasmids, one encounters various types, each with distinct functions and characteristics.
Conjugative Plasmids
Conjugative plasmids are like the social butterflies of the plasmid world, capable of transferring themselves from one bacterium to another through a process known as conjugation. This feature is instrumental in the spread of beneficial traits, such as antibiotic resistance, across bacterial populations. Their ability to facilitate horizontal gene transfer makes them critical in the dynamics of microbial evolution.
The key characteristic of conjugative plasmids is their tra (transfer) locus, a specific genetic sequence that encodes the proteins necessary for the conjugation process. A notable example is the F plasmid in Escherichia coli, which allows the bacteria to form a bridge-like connection with another cell, effectively sharing genetic information.
Advantages of conjugative plasmids include their role in rapidly disseminating advantageous genes among populations, while the potential downside is that this can also lead to the rapid spread of antibiotic resistance, posing challenges for public health.
Non-Conjugative Plasmids
In contrast, non-conjugative plasmids rely on other mechanisms for replication and transfer. They lack the necessary machinery to initiate conjugation on their own, often depending on the host's natural cellular processes. However, this doesn’t diminish their importance; non-conjugative plasmids can still play vital roles in the genetic landscape of bacteria.
A key feature of non-conjugative plasmids is their ability to replicate independently within the host cells, utilizing the host's replication machinery. Commonly employed in genetic engineering, these plasmids can serve as vectors for cloning genes or expressing proteins without the complexities of gene transfer between cells.
While non-conjugative plasmids serve essential research functions, their limitation lies in the lack of autonomous spreading mechanisms, potentially leading to a narrower reach in genetic dissemination.
R Plasmids
R plasmids, or resistance plasmids, are particularly noteworthy due to their key role in conferring antibiotic resistance. These plasmids harbor genes that can break down or inhibit the action of various antibiotics, allowing bacteria to thrive in hostile environments. The presence of R plasmids is a significant concern in clinical settings, as they can give rise to multi-drug-resistant bacterial strains.
A notable characteristic of R plasmids is the presence of multiple resistance genes, which can confer resistance to an array of antibiotics. Their capability to move between different bacteria highlights their contribution to the growing issue of antibiotic resistance in pathogens.
While R plasmids facilitate bacterial survival against antibiotics, their prevalence amplifies the challenge of treating bacterial infections, making this type of plasmid not only fascinating but critical to public health and medical research.
Col Plasmids
Col plasmids are specialized types that primarily encode genes for bacteriocins, substances that can kill other bacteria. This feature aids in competition among microbial communities, allowing the host bacterium to establish dominance in its environment.
The unique feature of col plasmids is their ability to produce proteins that specifically target and lyse closely related bacterial strains. This competitive advantage can foster survival in nutrient-limited settings or within host organisms. Col plasmids serve a dual purpose; not only can they provide a competitive edge, but they also hold potential applications in developing new antimicrobial therapies.
However, while col plasmids offer benefits in terms of competition, their specific targeting can sometimes restrict their overall efficacy against a broad spectrum of bacteria.
Role of Plasmids in Bacteria
Plasmids are not just passive entities; they actively engage in the life of their bacterial hosts. One primary role is their involvement in the adaptation and survival of bacteria in various environments. For instance, under duress, plasmids can enable bacteria to switch on stress response genes, enhancing their defense mechanisms.
Furthermore, plasmids foster genetic diversity within bacterial populations by facilitating horizontal gene transfer. This promotes rapid evolutionary changes, allowing bacteria to adapt to new challenges such as antibiotic exposure or environmental stressors.
Overall, the role of plasmids in bacteria underscores their importance in the broader tapestry of microbial ecology and evolution. As we continue to explore plasmid isolation techniques, it becomes evident that harnessing these entities holds endless potentials for applications ranging from medicine to agriculture.
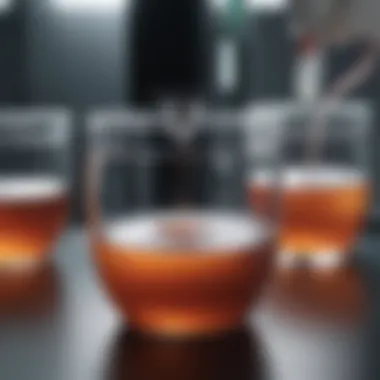
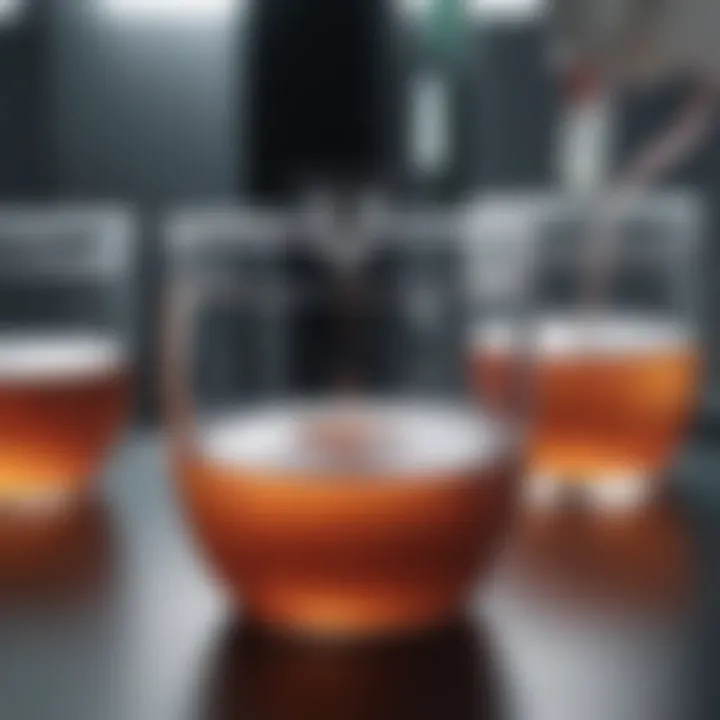
Importance of Plasmid Isolation
Plasmid isolation is a key process in microbiology and biotechnology that cannot be understated. Understanding the significance of this technique informs a multitude of scientific and practical applications. This part of the article breaks down how isolating plasmids can be a game-changer in various fields, emphasizing its relevance to genetic manipulation, ecological studies, and medicinal advancements.
In the realm of genetic engineering, plasmid isolation is of paramount importance. It serves as the bridge that connects the theoretical aspects of genetics with practical applications, particularly in the creation of genetically modified organisms. This process not only simplifies the task of manipulating genetic material but also enhances the precision with which genetic changes can be made.
Significance in Genetic Engineering
Cloning Applications
Cloning applications stand as one of the cornerstone aspects of genetic engineering, and the isolation of plasmids plays a critical role here. Through plasmid vectors, scientists can insert genes of interest into host cells for duplication and study. The common practice of using plasmids for cloning is especially beneficial in creating multiple copies of a DNA segment, which can then be utilized in various research projects or for protein production.
What sets cloning apart is its adaptability. Researchers can modify plasmids to include specific gene sequences, which is crucial when attempting to clone a wide variety of genes. Additionally, plasmids are relatively uncomplicated to manipulate. However, they also come with their unique challenges; not all plasmids ensure stable replication in every host organism, which can lead to unexpected outcomes in cloning.
Gene Therapy
Turning towards gene therapy, this area also greatly benefits from plasmid isolation. Harnessing plasmids to deliver therapeutic genes to patients opens new doors in treating genetic diseases. Essentially, these vectors can be engineered to carry corrected genes into the patient's cells, providing a potential cure for ailments ranging from cystic fibrosis to certain types of cancer.
The beauty of utilizing plasmids in gene therapy lies in their ability to express therapeutic proteins post-insertion. Additionally, they can be designed to target specific tissues, increasing the precision of treatment. Nonetheless, gene therapy using plasmids isn't without hurdles. Issues such as immune responses and insertional mutagenesis can arise, complicating treatment and outcomes.
Contributions to Microbial Genetics
Plasmid isolation doesn't just pave the way for advancements in genetic engineering; it also enriches the field of microbial genetics. By isolating and studying plasmids, scientists can unravel the complex relationships between different species of bacteria. This reveals valuable insights into the mechanisms of gene transfer, horizontal gene transfer, and the evolution of microbial populations.
Moreover, investigating plasmids leads to a better understanding of antibiotic resistance. Resistance genes are often found on plasmids, which can be shared between bacteria, facilitating the spread of resistance traits. Knowledge gained from plasmid studies can inform public health strategies and contribute to the development of new antimicrobial agents.
Implications for Antibiotic Resistance Research
The implications of plasmid isolation extend into the urgent area of antibiotic resistance. As mentioned, plasmids are vehicles for transmitting resistance genes among bacteria. This means that by isolating and studying plasmids, researchers can trace the origins of resistance traits, track their dissemination, and understand the contributing factors behind the rise of resistant strains.
Tracking resistant plasmids is essential in clinical settings, especially when devising strategies to combat infections caused by resistant bacteria. Insights gained through plasmid studies can also lead to the development of novel antibiotics or alternative treatment modalities aimed at overcoming resistance. In this regard, plasmid isolation serves not just as an academic exercise, but a vital tool in public health and therapeutic developments.
"Understanding plasmid isolation is akin to wielding a key that unlocks numerous avenues in biological research and medicine."
Methods for Plasmid Isolation
Plasmid isolation is a cornerstone of molecular biology. It serves as the gateway for researchers to access these important genetic elements. Understanding the methods for isolating plasmids from bacteria is essential for anyone stepping into the field of biotechnology, as these techniques can influence the outcomes of various applications, from cloning to gene therapy. Each method carries its own nuances, pros and cons, making a solid grasp of them pivotal.
Overview of Isolation Techniques
There’s a whole smorgasbord of techniques available for plasmid isolation. Broadly, these approaches can be categorized based on the underlying principles they exploit. Here’s a rundown of the main isolation methods that are popular in labs today:
- Alkaline Lysis Method: This technique harnesses pH to break open bacterial cells. It’s pretty much the bread and butter of plasmid isolation.
- Centrifugation Techniques: Methods like isopycnic and density gradient centrifugation utilize centrifugal force to separate plasmids from cellular components based on density.
- Instant Extraction Techniques: Heat shock and microwave-assisted extraction are quicker options that speed up the isolation process, tapping into drastic temperature changes.
- Use of Commercial Kits: Ready-to-use kits offer convenience and standardized protocols, but there's a trade-off when it comes to cost.
Alkaline Lysis Method
Principle
The alkaline lysis method hinges on the idea of disrupting the bacterial cell membrane using an alkaline solution. When the pH is raised, cellular components like chromosomal DNA and proteins are denatured, allowing plasmids to remain intact. One major characteristic of this principle is its simplicity and effectiveness, which has made it a leading choice in molecular labs.
Using alkaline lysis allows for a relatively quick and efficient extraction of plasmids, minimizing the handling of samples, which is a prime factor in reducing contamination risks. However, this method isn’t perfect; over-alkalization can lead to loss of plasmids by denaturing them alongside other DNA types, adding an element of caution.
Step-by-Step Procedure
The alkaline lysis procedure is methodical and can be broken down into a few key steps:
- Cell Lysis: Bacterial cells are resuspended and treated with an alkaline buffer solution.
- Neutralization: A neutralization buffer is added, prompting precipitation of the cell debris while keeping plasmids in solution.
- Centrifugation: The mix is spun to collect the cellular debris, leaving the plasmids in the supernatant.
- Purification: Lastly, isopropanol or ethanol can be used to precipitate the plasmids out of solution.
This straightforward procedure ranks high on efficiency. However, care must be taken during the neutralization stage, as rapid changes in pH can result in reduced yields if not done cautiously.
Advantages and Disadvantages
The alkaline lysis method is lauded for its:
- Time Efficiency: It typically takes less than an hour from start to finish.
- High Yield: It can yield a good amount of plasmid DNA, especially when structured properly.
Nevertheless, drawbacks do exist:
- Sensitivity to Conditions: Variables like pH and temperature can impact the quality and yield.
- Potential Contamination: If not executed under sterile conditions, there’s a chance of contaminating the plasmid sample, which can jeopardize downstream applications.
Centrifugation Techniques
Isopycnic Centrifugation
Isopycnic centrifugation is an intriguing method that relies on the principle of buoyancy. It separates biomolecules based on their density rather than size or shape. In this technique, plasmids are purified when placed in a density gradient medium such as cesium chloride. The key characteristic of isopycnic centrifugation is its meticulous nature; it can achieve a high degree of purity.
While this precision is advantageous, the process can be quite lengthy and requires a fair bit of expertise, not to mention the need for specific lab equipment. Thus, it is not the go-to choice for all labs.
Density Gradient Centrifugation
As a sister technique to isopycnic centrifugation, density gradient centrifugation functions in much the same way but often tends to use a wider range of materials for achieving density separation. This method can yield highly purified plasmid DNA, and it’s especially useful in the case of larger plasmids or those found in difficult bacterial strains.
However, the methodology can be complex, requiring careful preparation of gradient solutions. Its specialized nature strikes a balance between efficacy and practicality, often making it a second-choice for many teams aiming for rapid results.
Instant Extraction Techniques
Heat Shock Method
The heat shock method is a quick and simple strategy to create competent cells. By shocking bacteria with sudden temperature changes—usually a shift from 0 degrees Celsius to 42 degrees Celsius—plasmids are often taken up more efficiently. This quick turnaround is what makes it a favorite among many labs for instant plasmid extraction.
But like most rapid methods, there’s a reliance on optimal timing and conditions. Improper execution can result in lower efficiencies, which could deter researchers from relying solely on this method.
Microwave-Assisted Extraction
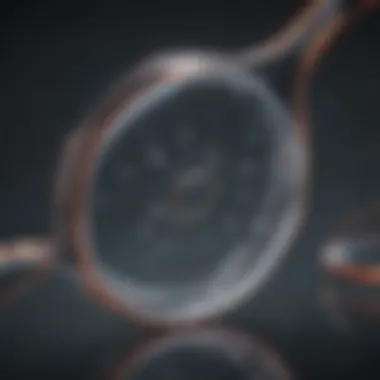
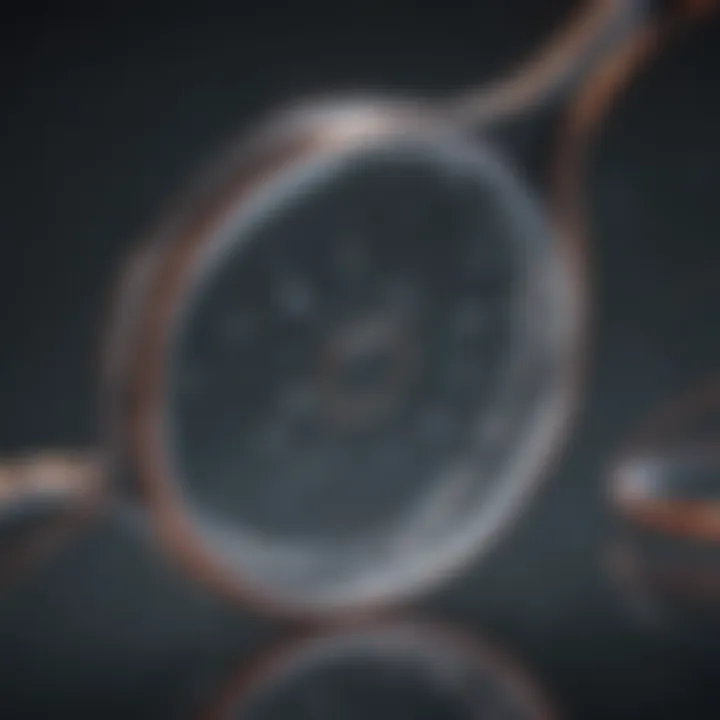
A fairly novel approach, microwave-assisted extraction shortens the plasmid isolation time significantly. Here, microwave energy is used to disrupt the cell membrane, allowing plasmids to be released more efficiently.
This technique offers a unique twist to traditional methods, reducing the processing time and making it particularly useful for high-throughput situations. Yet, it may require careful calibration of microwave exposure to avoid degrading sensitive plasmid DNA, creating its own challenges.
Use of Commercial Kits
Advantages
Commercial kits often promise a user-friendly experience. With pre-packaged reagents and detailed protocols, they take the guesswork out of the process, making them ideal for non-expert users or those who may lack specialized tools.
- Standardized Protocols: These kits come with tried-and-true methods that can enhance reproducibility.
- Time-Saving: They significantly reduce hands-on time compared to traditional methods.
However, cost can be prohibitive for some users, particularly in budget-restricted environments, limiting their accessibility.
Limitations
While commercial kits can be quite beneficial, they are not without issues:
- High Cost: They can be expensive, particularly for labs on a tight budget.
- Limited Flexibility: Users may find that protocols aren’t easily adaptable to specific needs, thereby constraining innovation or exploration in novel areas.
Despite their limitations, the convenience that commercial kits bring can often outweigh drawbacks, especially for those just starting out or needing reliable consistent results.
Quality Control in Plasmid Isolation
Quality control plays a crucial role in plasmid isolation, ensuring that the retrieved plasmids maintain the integrity and functionality necessary for downstream applications. Without stringent quality control measures, the isolation process can lead to compromised yield and purity, which can undermine the findings of experiments or the efficacy of biotechnological applications. Thus, it becomes essential to assess both the purity and the yield of isolated plasmids, as these factors are directly linked to the success of genetic manipulation and analysis.
Assessing Purity and Yield
Assessing the purity and yield of isolated plasmids involves meticulous checks at various stages of the isolation process. High purity is essential because contaminants, such as proteins or genomic DNA, can interfere with subsequent manipulations, such as transformation into host cells or restriction digestion. The yield of plasmid DNA is equally important; if the yield is subpar, it may not suffice for its intended application. A common benchmark for high-purity plasmid DNA is an A260/A280 ratio of around 1.8, indicating minimal contamination from proteins.
Practical steps for assessing purity and yield:
- Spectrophotometric Analysis: This is a standard method to measure the concentration of plasmid DNA and assess its purity based on the absorbance at specific wavelengths.
- Fluorometric Methods: More accurate than spectrophotometry, these methods utilize fluorescent dyes that bind specifically to nucleic acids, providing a more reliable quantification of DNA.
Techniques for Verification
Verification techniques confirm that the isolated plasmids meet the required quality standards. The two prevalent verification methods include agarose gel electrophoresis and restriction enzyme digestion. Each technique offers unique insights into the plasmid quality and ensures that the right processes have been followed.
Agarose Gel Electrophoresis
Agarose gel electrophoresis is a staple in molecular biology, allowing researchers to separate DNA molecules based on size. Its contribution to quality control in plasmid isolation is invaluable, as it can visually demonstrate the integrity of plasmid DNA. The technique is straightforward: plasmid samples are loaded into wells in an agarose gel and subjected to an electric current.
Key Characteristics:
- Resolution: Capable of resolving DNA fragments ranging from hundreds to thousands of base pairs.
- Visualization: After electrophoresis, ethidium bromide staining allows for visualization under UV light.
Agarose gel electrophoresis stands out because it can differentiate between supercoiled, linear, and relax forms of plasmids. Supercoiled plasmids are preferred as they are more likely to be efficiently transformed into bacteria. While the method is popular for its effectiveness, a downside is that it requires additional steps for quantification, as the gel does not directly provide concentration values.
Restriction Enzyme Digestion
Restriction enzyme digestion offers another layer of verification by cutting the plasmid at specific sequences. By analyzing the resultant fragments, researchers can ascertain the plasmid's identity and confirm whether it matches the expected pattern.
Key Characteristics:
- Specificity: Each restriction enzyme recognizes a particular DNA sequence, ensuring targeted analysis.
- Fragment Analysis: The size and number of resulting fragments can indicate if the construction of the plasmid was successful.
This method is particularly beneficial as it can not only verify the presence of the plasmid but can also ensure the correctness of the inserted gene sequences. On the downside, if a plasmid has multiple recognition sites for a given enzyme, this could lead to multiple fragments, complicating the interpretation.
By adhering to rigorous quality control standards and employing verification techniques like agarose gel electrophoresis and restriction enzyme digestion, researchers can assure the reliability of their plasmid isolation processes, thus enabling precise and effective applications in genetic engineering and biotechnology.
Applications of Isolated Plasmids
Understanding the applications of isolated plasmids is paramount in our journey through microbial genetics and biotechnology. These small, circular DNA molecules serve as tools that enable scientists to manipulate and explore the functions of genes within various organisms. Their role spans multiple disciplines, turning fundamental research into practical applications, thus underscoring the true impact of plasmid isolation.
In Synthetic Biology
Synthetic biology has emerged as a cutting-edge field that combines biology, engineering, and computational science to build and design biological systems. Isolated plasmids play a pivotal role in this discipline by functioning as vectors for gene transfer. This process supports the creation of novel organisms that can produce biofuels, pharmaceuticals, or even biodegradable plastics.
Plasmids allow researchers to harness genetic components from various sources, enabling the development of tailored organisms with specific functions. For instance, plasmids can be engineered to contain sequences from other organisms, giving rise to genetically modified strains that express desirable traits, such as increased disease resistance or enhanced metabolic capabilities. The flexibility to modify plasmids is what makes them an invaluable asset in synthetic biology, ultimately blurring the lines between natural systems and engineered solutions.
In Agricultural Biotechnology
Agricultural biotechnology represents another significant domain where isolated plasmids find critical application. Here, they are utilized to enhance crop traits, resist pests, and increase yield. By introducing plasmids containing specific genes into plant genomes, scientists bridge the gap between traditional breeding and modern genetic engineering.
Genetically Modified Organisms
The heart of agricultural biotechnology often lies in genetically modified organisms (GMOs). These are crops developed through the introduction of new genetic material via plasmids, granting them enhanced characteristics. For example, Bt corn is a common genetically modified organism engineered to express a protein from the bacterium Bacillus thuringiensis, which provides resistance against certain pests.
The key characteristic of GMOs is their ability to withstand environmental stressors while reducing the need for chemical pesticides. This not only leads to economic benefits for farmers but also contributes to greater sustainability by minimizing chemical usage. However, it is important to consider public perception and regulatory challenges surrounding GMOs, as many still harbor fears about their safety and ecological consequences.
Biopesticides
Biopesticides represent another fascinating facet of agricultural biotechnology, developed using plasmids that can deliver plant-protecting genes. These biologically-derived products offer an alternative to traditional chemical pesticides. For instance, some plasmids can be engineered to include genes coding for proteins toxic to specific pests while being harmless to beneficial organisms.
The standout aspect of biopesticides is their environmentally friendly profile. They are often biodegradable and target only particular pests, thus preserving beneficial insect populations and reducing inadvertent damage to the ecosystem. However, scalability and consistency in efficacy remain challenges that the development of biopesticides continues to grapple with.
In Pharmaceutical Development
Another significant area where isolated plasmids find their home is in pharmaceutical development. They serve as reservoirs for genes encoding therapeutic proteins and vaccines, allowing for large-scale production and innovative treatments.
Vaccine Production
Plasmids are prominent players in vaccine production, particularly in the formulation of DNA vaccines. These vaccines consist of plasmid DNA that encodes antigens corresponding to pathogens, eliciting an immune response without using live pathogens. The key characteristic of DNA vaccines is their ability to stimulate both humoral and cellular immunity.
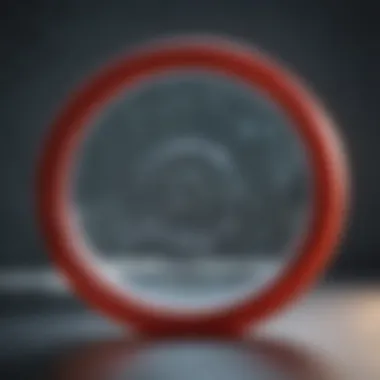
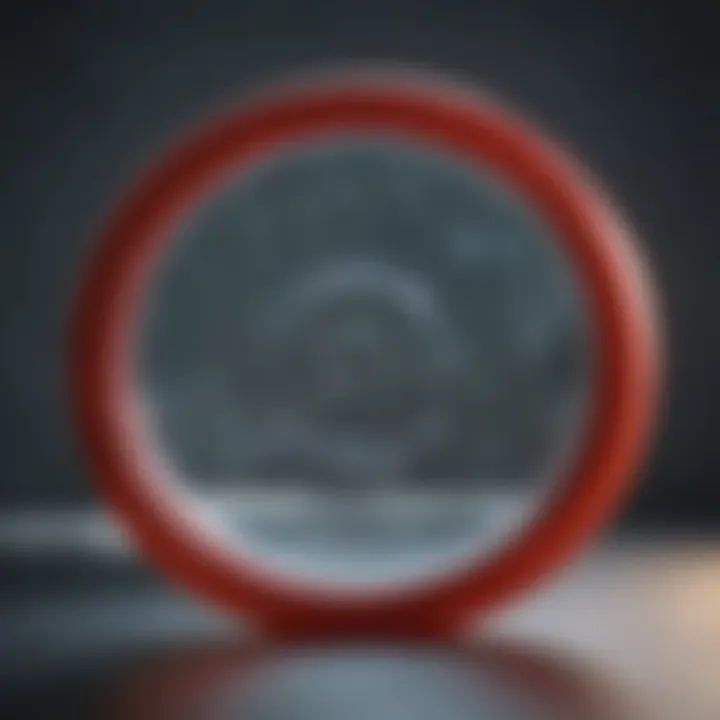
The appealing benefit of using plasmids in vaccine production lies in their stability and ease of manipulation. This ease allows rapid development in response to emerging health threats, as seen during the recent pandemic where plasmid-based platforms were utilized for vaccine design. However, challenges remain, including ensuring effective delivery and long-lasting immune responses in vaccinated individuals.
Therapeutic Protein Synthesis
Lastly, the synthesis of therapeutic proteins is heavily reliant on plasmids. They enable the production of proteins such as insulin, monoclonal antibodies, and enzymes, which are crucial for treating various conditions.
Plasmid vectors used in therapeutic protein synthesis often contain strong promoters and elements that facilitate high expression levels in host cells. This means that minimal quantities of plasmids can lead to significant yields of therapeutic proteins. Nonetheless, factors like post-translational modifications and the purification process can introduce complexities that require attention.
The versatility of plasmids not only demonstrates their intrinsic scientific significance but also underscores their power as instruments of change across multiple sectors, notably within synthetic biology, agricultural biotech, and pharmaceutical advancements.
Challenges in Plasmid Isolation
The process of isolating plasmids from bacteria is not without its hurdles. Understanding these challenges is paramount, not just for ensuring a successful extraction but also for advancing research in genetic engineering and microbial genetics. Every method for plasmid isolation comes with its own set of problems that can affect not only the yield of plasmids obtained but also their quality. Here, we delve into three significant challenges: contamination issues, low yield factors, and variability in plasmid profiles.
Contamination Issues
Contamination during plasmid isolation can be a thorn in the side of researchers. Plasmid preparations risk being tainted by genomic DNA, proteins, or other cellular components, which may compromise their subsequent applications. This is particularly critical in experiments where purity is essential, such as those utilizing plasmids for cloning or gene therapy.
Inadequate cell lysis, improper handling, or subpar reagents can all lead to such contamination. Take, for example, the alkaline lysis method: if the cell lysis isn't fully effective, it can leave behind unwanted genomic DNA or cellular debris in the solution. Researchers often see undesirable results weeks down the line, realizing that the plasmid they were working with contained contaminants that biased their experiments.
To mitigate these issues, rigorous purification steps like phenol-chloroform extraction or the use of spin columns are often employed, which adds time and complexity to the procedure. The need for careful handling and validated reagents emphasizes the importance of maintaining a sterile environment throughout the process.
Low Yield Factors
A frequent concern in plasmid isolation is the yield. Low yield can stem from various factors, including suboptimal growth conditions of the bacterial culture or inefficient extraction techniques. For instance, if the bacterial strain isn't cultured under conditions where plasmid replication is robust, the resultant plasmid quantity can be disappointingly low.
Another contributor to low yields can be the bacterial strain being used for isolation. Some strains may be genetically predisposed to harbor less plasmid content or could be less amenable to the techniques used for extraction. A classic example is using a plasmid that confers antibiotic resistance; if the plasmid does not give a selective advantage under the growth conditions, the bacterial population may not maintain high levels of plasmids.
To counteract low yields, researchers often explore optimizing culture conditions or using more robust techniques tailored to the specific plasmids being isolated. However, this can require a fair amount of trial and error, which isn’t always feasible.
Variability in Plasmid Profiles
Variability in plasmid profiles adds another layer of complexity to plasmid isolation. Different bacterial strains, whether through natural mutations or through selective pressure during culturing, can result in diverse plasmid profiles. This variability can lead to inconsistent behaviors in subsequent applications, which is especially problematic in industrial settings where reliability is key.
For instance, researchers might find that even when isolating plasmids from the same strain and under similar conditions, the plasmids can differ in copy number or in the presence of mutations. Such profile changes can affect downstream applications, such as cloning efficiency or protein expression levels.
To address this variability, many researchers employ techniques like plasmid fingerprinting or sequencing. These methods help confirm the integrity and consistency of plasmids, ensuring that the isolated plasmids provide the desired functionality for experimental needs.
The reliability of results in plasmid-based research is heavily reliant on overcoming contamination, yield, and variability issues. Strategies to address these challenges are essential for successful applications.
Through understanding these challenges—contamination issues, low yield factors, and variability in plasmid profiles—researchers can better navigate the complexities of plasmid isolation. While the path may be rocky, addressing these challenges head-on ensures that plasmid isolation continues to serve as a cornerstone of genetic research.
Future Trends in Plasmid Research
The field of plasmid research is on the cusp of transformation, thanks to a myriad of technological breakthroughs and evolving scientific perspectives. Understanding these future trends is essential not only for foundational research but also for applied sciences such as medicine, agriculture, and environmental stewardship. The importance of exploring these trends lies in their potential to vastly improve the efficiency and efficacy of plasmid isolation, while also broadening their applications in gene therapy, synthetic biology, and beyond.
Advancements in Isolation Techniques
Isolation techniques continually evolve, reflecting advancements in biochemistry and molecular biology. One notable area is the development of more efficient and gentle extraction processes. Traditional methods like alkaline lysis, while reliable, often compromise plasmid integrity due to harsh conditions.
Current research is focusing on techniques that employ less harsh conditions, such as the use of non-ionic detergents and enzymatic approaches that minimize DNA degradation. Moreover, methods utilizing nanotechnology are gaining traction. These methods leverage nanoscale materials that bind selectively to plasmid DNA, thus streamlining the isolation process.
- Advantages of Advanced Techniques:
- Higher purity and yield.
- Reduced risk of enzymatic damage.
- More straightforward and faster procedures.
Integration of Automation in Procedures
As the realm of molecular biology progresses, automation is becoming a cornerstone of plasmid isolation processes. Robotics and automated liquid handling systems are now being used to improve both accuracy and throughput in laboratories. By automating routine steps, researchers can let machines handle the more tedious tasks—thus freeing them for more strategic endeavors.
This integration not only leads to increased efficiency but also reduces human error—a common pitfall in manual procedures. Furthermore, automation allows for the consistent handling of reproducibility, a critical factor in research.
- Benefits of Automation:
- Increased throughput for plasmid extraction.
- Consistency and precision in procedures.
- Reduced labor costs in the long run.
Enhanced Applications in Gene Editing
The interplay between plasmid research and gene editing is becoming increasingly important. As technologies like CRISPR continue to revolutionize genetics, the need for high-quality plasmid supplies is paramount. Plasmids serve as vectors for delivering gene-editing tools into target cells, making their effective isolation crucial for successful interventions.
In the coming years, we can expect more sophisticated applications of plasmid technology in clinical settings. For instance, researchers are aiming to engineer plasmids that can be customized on-the-fly based on the specific genetic modifications required for individual patients in gene therapy applications.
- Future Directions in Gene Editing:
- Customized plasmids for personalized medicine.
- Improved precision in gene delivery mechanisms.
- Enhanced efficacy of therapeutic interventions.
"The fusion of advanced plasmid isolation techniques with cutting-edge automation and gene editing opens new avenues for exploring the genetic landscape of life forms, potentially altering treatment paradigms in various fields."
In summary, the future of plasmid research is not just a matter of refining techniques but about rethinking how these genetic elements are utilized. As technology continues to advance, so will the potential applications and relevance of plasmid research across multiple disciplines.
Ending
In this final section, it’s crucial to reflect on the significance of plasmid isolation from bacteria. This topic doesn't just scratch the surface of biotechnology; it digs deep into the DNA of nature itself, showcasing the intricate relationships between genetic engineering and microbial biology.
Summary of Key Points
The essence of this article can be drawn from various pivotal discussions:
- The Role of Plasmids: These circular DNA entities are key players in bacterial adaptability and resistance. Their ability to transfer genes between organisms enables rapid evolution, particularly in response to environmental pressures like antibiotic treatments.
- Isolation Techniques: From alkaline lysis to centrifugation, each method carries its own set of pros and cons. They can affect purity and yield, which is a major consideration for researchers.
- Applications and Future Implications: Isolated plasmids empower genetic engineering, enhance agricultural biotechnology, and contribute to pharmaceutical innovations, laying the groundwork for breakthroughs in gene therapy and vaccine development.
The Evolving Landscape of Plasmid Research
As we step into the future, the field of plasmid research appears dynamic and promising. Technological advancements are continually paving the way for novel isolation methods. There’s a marked trend towards automation, aiming to not only streamline the extraction process but also to minimize human error, boosting reproducibility in experimental scenarios. Moreover, with the rise of CRISPR technology and other gene-editing tools, the applications of plasmids are expanding into realms that were once thought to be purely theoretical. The potential to edit specific genomic regions heralds a new era for treatments and crop improvements that could be game-changers.
The depth of understanding surrounding plasmids is crucial for empowering future research and influencing practical applications in fields ranging from medicine to environmental sciences. As researchers continue to engage with these genetic elements, one can expect a trail of discoveries that reshapes our understanding of genetics and its applications in everyday life.
"Plasmids serve as a gateway to the genetic toolkit of nature, holding the key to innovative solutions and advancements across multiple disciplines."
Indeed, as this article unfolds, it becomes clear that the future of plasmid research is not just a scientific endeavor but a multidimensional pathway toward solutions for some of society's pressing challenges.