Comprehensive Guide to Protein Stability Assays
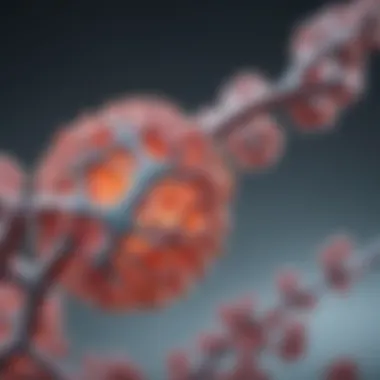
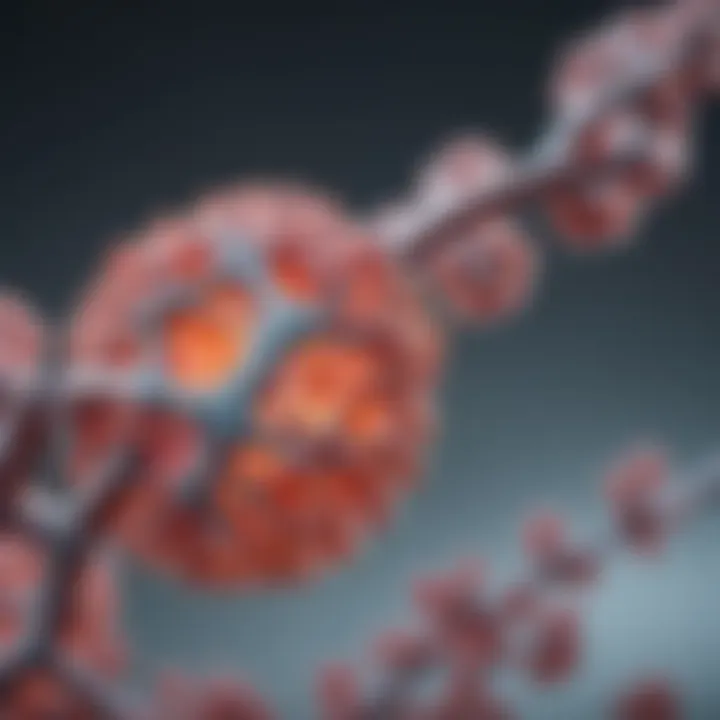
Intro
Protein stability assays play a crucial role in both biochemistry and molecular biology by offering insights into how proteins behave under various conditions. Understanding protein stability is vital for numerous applications, including drug development, protein engineering, and therapeutic interventions. When proteins are stable, they perform their functions effectively. Thus, assessing their stability helps researchers design better drugs and optimize therapeutic proteins.
In this article, we aim to delve into the diverse techniques and applications associated with protein stability assays. We will explore the fundamental principles of these assays, the methodologies commonly employed, and their significance in advancing scientific research. This exploration will not only broaden the understanding of protein stability but also highlight its impact on real-world applications.
Research Highlights
Overview of Key Findings
The key findings from various studies indicate that different techniques for assessing protein stability yield unique insights, which can be applied to numerous fields. For example, methods such as differential scanning fluorimetry, circular dichroism, and thermal shift assays have shown varied results depending on the proteins tested and the conditions applied.
Overall, these techniques have established crucial benchmarks for evaluating protein behavior, and they have been instrumental in the understanding of how proteins interact with potential drug candidates.
Significance of the Research
Assessing protein stability is not merely an academic exercise. The implications extend to drug design, where stability tests can predict how a drug will perform in vivo. If a drug's target protein is unstable, it may lead to ineffective therapeutic responses. Therefore, protein stability assays can assist researchers in making informed choices during the development phases.
Original Research Articles
Summary of the Article
While this article summarizes various methods related to protein stability, each original research piece expands on the intricacies tied to specific protocols, results, and applications within the broader spectrum of stability assays.
Academic literature demonstrates how variations in environmental conditions impact protein architecture and function. The assessment of stability often leads to discovering novel compounds that enhance or inhibit protein function, which has vast implications in pharmacology.
Author Contributions
Contributors to this field span a range of disciplines, including biochemistry, molecular biology, and pharmaceutical sciences. Their collaborative efforts have led to significant advancements in understanding the multifaceted nature of protein stability assessment. Each researcher brings unique perspectives, influencing methodologies or applications of findings in real-world scenarios.
The potential for protein stability assays to bridge gaps between basic research and practical applications cannot be overstated. Enhancing our grasp of these techniques will empower scientists to enhance drug development and therapeutic efficacy.
Prelude to Protein Stability Assays
The study of protein stability assays is pivotal in understanding how proteins behave under various conditions. Proteins serve as essential components in biochemistry and molecular biology, influencing a multitude of biological processes. Protein stability refers to the ability of a protein to maintain its functional conformation under changing environmental conditions, which is crucial for its activity. The significance of protein stability assays lies in their capacity to provide insights into the stability and functionality of proteins, which ultimately informs research in drug development, protein engineering, and therapeutic applications.
Understanding these assays supports optimal design and application, giving rise to more effective biomolecules. As the field of biopharmaceuticals continues to expand, the accuracy of stability assessments becomes even more vital. A well-conducted stability assay not only informs about the robustness of protein formulations but also helps predict the interaction of these proteins when exposed to physiological settings.
In this exploration, we will elucidate various aspects related to stability assays, including their definitions, historical developments, and significance in scientific research. We will delve into methodologies, data interpretation, and broader implications in drug development and engineering. By comprehensively analyzing protein stability assays, we aim to enhance understanding and expertise amongst scholars and industry professionals alike.
Definition and Importance
A protein stability assay is a laboratory technique used to measure the stability of a protein under different environmental conditions, such as temperature, pH, and concentration. The primary goal is to ascertain how these factors impact a protein's structure and function.
The importance of these assays extends beyond mere academic curiosity. In practical terms, protein stability can determine the efficacy and safety of therapeutics, which emphasizes the need for rigorous testing. For example, in the context of biologics, stability assays play a significant role in characterizing product quality and shelf life, critical factors that affect market acceptance.
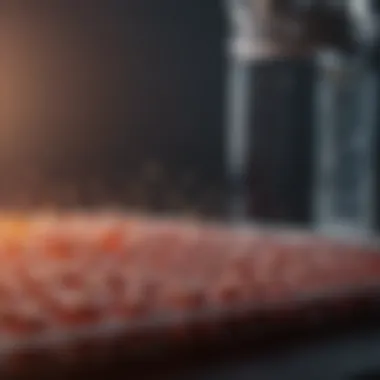
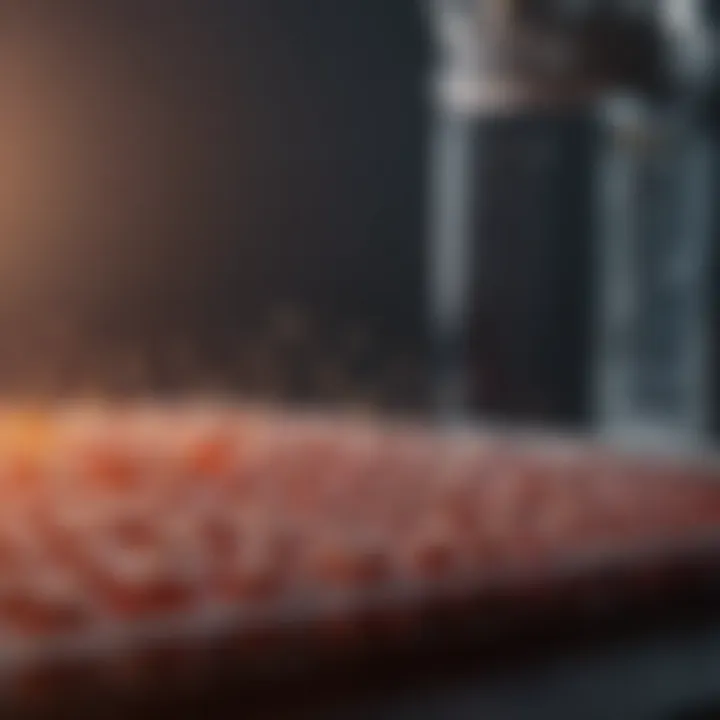
Moreover, insights derived from stability assays can guide modifications in protein design to enhance stability and performance. This is especially relevant for therapeutic proteins, where structural integrity is often linked to therapeutic effectiveness.
Historical Context
The evolution of protein stability assays can be traced back to early biochemistry studies, where researchers began to explore protein denaturation and refolding. Initial techniques were rudimentary but laid the groundwork for modern methodologies. Over the decades, advancements in spectroscopy and calorimetry technology revolutionized the way researchers assess protein stability.
As practices became more sophisticated, the advent of differential scanning calorimetry in the 1970s marked a turning point, providing high-resolution data about protein transitions. This period also saw the integration of circular dichroism and fluorescence spectroscopy as essential tools for stability measurement.
Today, protein stability assays are integral to numerous fields, with ongoing research continually refining analytical techniques, thereby improving the reliability and applicability of results. Understanding the historical context highlights how methods have evolved and underscores the continuing importance of these assays in scientific advancements.
Underlying Principles of Protein Stability
Understanding the underlying principles of protein stability is essential for anyone engaged in biochemistry and molecular biology. These principles shape how proteins behave under different conditions and influence various applications, from drug development to protein engineering. By examining these foundational concepts, researchers can better predict the behavior of proteins, enabling more effective manipulation and utilization in laboratory environments.
Factors Affecting Protein Stability
Protein stability is influenced by several factors. These factors can be broadly categorized into environmental and intrinsic variables.
- pH Levels: The pH of the surrounding environment can affect the charge and, consequently, the interactions between amino acids within the protein. Deviations from the optimal pH can lead to denaturation.
- Temperature: Elevated temperatures may increase molecular motion, potentially leading to structural destabilization. Each protein has a specific range of temperatures within which it maintains its stable conformation.
- Concentration of Salts: Salts can have a stabilizing or destabilizing effect. They can promote proper folding or facilitate aggregation depending on their concentration and type.
- Additives: Specific chemicals, like glycerol or certain detergents, can protect proteins from unfolding. Understanding how these additives work can enhance the stability of proteins in various applications.
These factors provide critical insights into the manipulative approaches researchers can adopt to enhance protein stability in experimental settings.
Thermodynamics of Stability
The thermodynamics of stability delves into energy considerations associated with protein conformations. Stability can be characterized by the difference in Gibbs free energy between the folded and unfolded states of a protein.
The key concepts include:
- Free Energy: The lower the free energy of a protein in its folded state compared to its unfolded state, the more stable the protein is. This thermodynamic perspective is crucial for understanding folding pathways and stability thresholds.
- Enthalpy and Entropy: These two thermodynamic parameters play pivotal roles in protein stability. Enthalpic contributions arise from attractive forces between atoms and the overall structural integrity. Entropy relates to the disorder in the system; higher entropy often denotes lower stability.
- Stability Measurements: Techniques such as differential scanning calorimetry can assess the calorimetric stability, providing quantitative data on the unfolding and refolding processes.
In summary, grasping these thermodynamic principles equips researchers with the knowledge necessary to predict stability outcomes, tailor experimental conditions, and advance the study of protein behaviors in multifaceted biological environments.
Common Methodologies of Protein Stability Assays
Understanding the common methodologies of protein stability assays is crucial in biochemistry research. These methods not only evaluate the stability of proteins but also provide insights into their practical applications in various fields like drug development. Each technique comes with its own benefits and considerations that researchers must take into account to choose the best method for their needs.
Differential Scanning Calorimetry
Differential Scanning Calorimetry (DSC) is a prominent technique for assessing protein stability. It measures the heat changes associated with transitions in physical state, such as folding and unfolding of proteins. The resulting data provides valuable kinetic and thermodynamic parameters, such as the melting temperature and enthalpy changes. These metrics are essential for understanding how environmental factors can influence protein stability.
- Importance: DSC offers high sensitivity and precision, making it suitable for relatively small protein samples.
- Considerations:Sample concentration and buffer composition must be carefully controlled because they can significantly affect results. Also, the presence of buffers or additives can perturb protein stability, which might complicate data interpretation.
Circular Dichroism Spectroscopy
Circular Dichroism (CD) Spectroscopy is another widely utilized method for analyzing protein stability. By examining the absorption of circularly polarized light, CD can provide information on the secondary structure of proteins. This technique is particularly useful for detecting structural changes that occur during thermal or chemical denaturation.
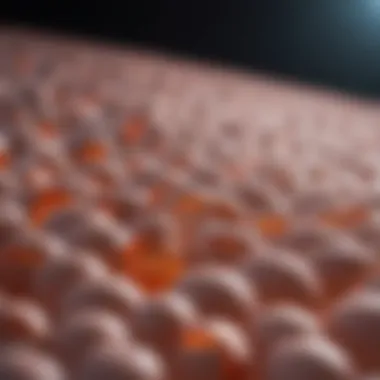
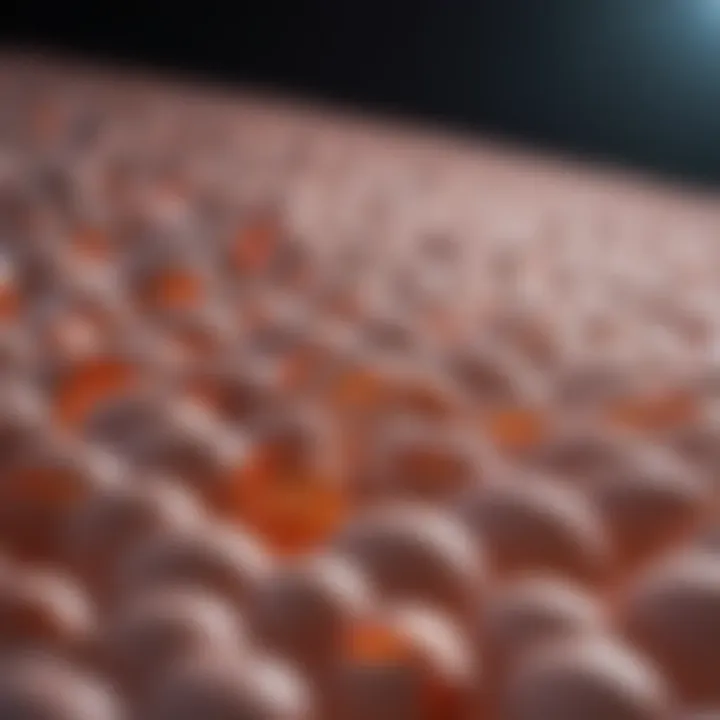
- Benefits: CD is relatively quick and requires minimal sample preparation compared to other methods. It is non-destructive, allowing the same sample to be used for subsequent tests.
- Considerations: The interpretation of CD spectra can be complex, requiring calibration against standard proteins. Additionally, it may not provide detailed information about tertiary or quaternary structures, limiting its application in some contexts.
Fluorescence Spectroscopy
Fluorescence Spectroscopy is employed to monitor protein stability via changes in the fluorescence intensity or emission wavelength. This technique relies on the intrinsic fluorescence of aromatic amino acids or the use of fluorescent probes. Changes in these fluorescence properties can indicate conformational changes in the protein structure, particularly during unfolding.
- Significance: This method allows for real-time observation and can detect subtle changes in protein stability under different conditions.
- Limitations: The requirement for pure protein samples and potential interference from external factors, such as pH and ionic strength, can complicate results. Probes must also be chosen carefully to avoid issues with specific interactions.
Thermal Shift Assays
Thermal Shift Assays (TSA) are increasingly popular for studying protein stability. This method assesses the stability of proteins based on their melting temperature in the presence of various ligands or denaturants. As the temperature rises, the protein denatures, and this shift can be monitored using fluorescent dyes that bind to unfolded proteins.
- Advantages: TSAs are straightforward and can be performed in high-throughput formats, making them cost-effective for screening large libraries of compounds.
- Challenges: Accurate interpretation of thermal shifts can be affected by dye choice and sample conditions. Furthermore, the assay may not fully capture kinetic aspects, making it less informative for dynamic stability assessments.
Overall, selecting the proper methodology for protein stability assays depends on multiple factors including research objectives, sample characteristics, and the specific information sought. Each technique has its own strengths and weaknesses that must be recognized to ensure reliable results.
Interpreting Protein Stability Assay Results
Interpreting the results of protein stability assays is crucial for understanding how a particular protein behaves under various conditions. This analysis provides insights not only into the inherent properties of the protein itself but also into its potential applications in fields such as drug development and protein engineering. Proper interpretation can lead to significant advancements in these domains by guiding researchers in optimizing conditions for protein stability and functionality.
Data Analysis Techniques
Data analysis techniques utilized in interpreting protein stability assay results are diverse. They range from simple graphical representations to complex statistical models. Below are some notable methods commonly employed:
- Thermal denaturation profiles: Provides a graphical representation of unfolding temperatures, which indicates thermal stability. Plots can be generated to visualize melting temperatures and other thermodynamic parameters.
- Kinetic analysis: Involves measuring the rate of protein unfolding under various stress conditions. This technique can yield valuable information about the stability of the protein.
- Software tools: Programs such as GraphPad Prism and MATLAB can be used for fitting models to experimental data. These are helpful for performing detailed kinetic analyses, allowing for extrapolation of stability information.
- Comparative analysis: Comparing results across different conditions or formulations can help identify optimal stability conditions for a specific protein. This method can highlight effects of pH, temperature, or the presence of ligands.
Accurate application of these techniques leads to more robust conclusions about protein behavior. It becomes essential to select the appropriate method based on the specific protein characteristics and experimental conditions.
Common Pitfalls
Interpreting protein stability assay results is complex, and researchers often encounter common pitfalls. Awareness of these can prevent misinterpretations or incorrect conclusions. Some issues include:
- Overlooking variability: Protein stability assays can yield variable results based on slight changes in experimental conditions. It is critical to analyze replicate data and account for variability in interpretations.
- Inadequate controls: Lacking controls can lead to faulty conclusions about the stability of the target protein. Using appropriate controls is vital to differentiate between experimental effects and inherent variability.
- Misapplication of analysis tools: Applying inappropriate statistical tools can result in misleading outcomes. Ensure the chosen method is suitable for the data type and research question.
- Ignoring environmental factors: Factors such as temperature and concentration can significantly impact protein behavior, yet researchers may overlook this aspect & discard important environmental considerations.
Consider a meticulous approach to your data analysis and keep potential pitfalls in check. This ensures insights drawn from protein stability assays are accurate and reliable.
Applications of Protein Stability Assays
Protein stability assays play a crucial role in modern biochemistry and biotechnology. Their application spans several fields, impacting the development of new drugs, the refinement of protein engineering techniques, and the advancement of biologics and therapeutics. Understanding these applications helps elucidate the broader significance of protein stability in both research and industry.
Drug Development
In drug development, protein stability assays are vital for determining the feasibility and formulation of biologic drugs. The efficacy of a drug often hinges on the stability of the protein it contains, which can be affected by factors such as temperature, pH, and concentration. By employing techniques such as differential scanning calorimetry or thermal shift assays, researchers can ascertain how well a protein will perform under physiological conditions. This can lead to more effective drug formulations with improved shelf life and reduced adverse effects.
Moreover, stability assays aid in the identification of best candidates for drug development. During the screening process, proteins that show greater stability are often prioritized. This added layer of scrutiny can save time and resources, enhancing the overall efficiency of the drug development pipeline.
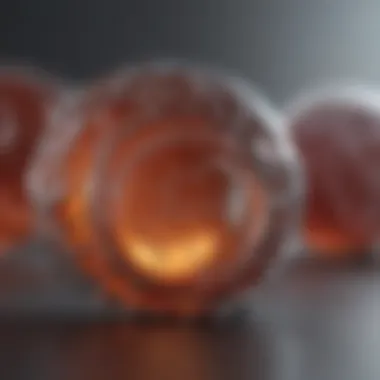
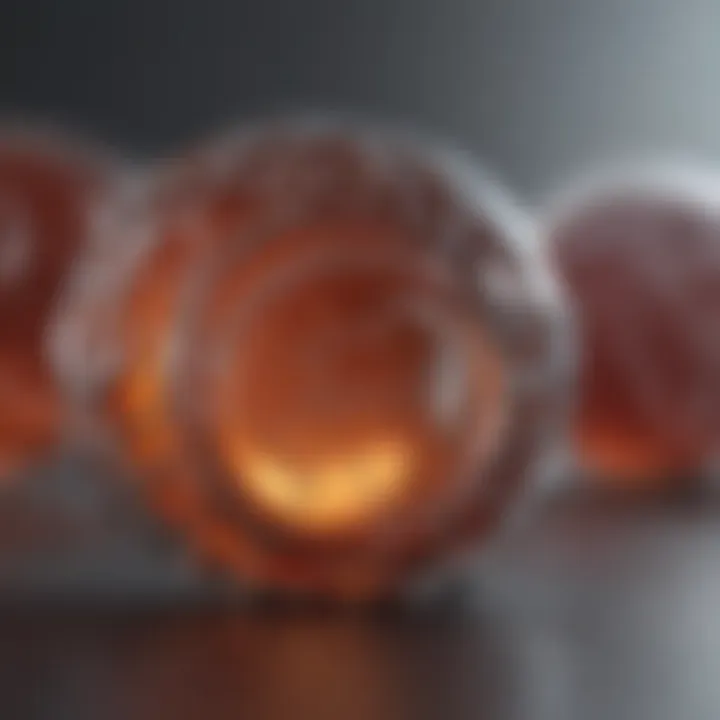
Protein Engineering
Protein engineering is another domain where stability assays prove indispensable. When designing proteins with novel functions, it is important to ensure that these engineered forms maintain their stability over time. Stability assays provide insights into how modifications affect the folding, activity, and longevity of the proteins. For example, amino acid substitutions can be systematically evaluated for their impact on stability using techniques like circular dichroism spectroscopy.
Additionally, these assays inform the design process by helping select mutations that optimize functionality while maintaining overall stability. By understanding stability profiles, researchers can create proteins tailored for specific applications, which significantly expands the potential of protein engineering.
Biologics and Therapeutics
The realm of biologics and therapeutics has greatly benefitted from advances in protein stability assays. Many modern therapeutics are based on recombinant proteins, monoclonal antibodies, or other biologics. The stability of these products is not only critical for their effectiveness but also impacts their safety and efficacy.
Stability assays help predict how these biologics will behave during storage and transportation, which is essential for compliance with stringent regulatory standards. Additionally, understanding protein stability allows researchers to formulate biologics that remain stable over a range of conditions, reducing the risk of degradation that can lead to loss of efficacy.
"Failure to assess protein stability can lead to significant setbacks in drug approval processes and patient treatment outcomes."
Furthermore, stability profiling can guide post-approval changes or formulation adjustments, ensuring that the integrity of the therapeutics is maintained throughout their lifecycle. Overall, stability assays provide a framework for optimizing biologic therapies, ensuring that they are both effective and safe for patient use.
Challenges in Protein Stability Assays
Protein stability assays play a crucial role in understanding protein behavior under various conditions. However, significant challenges exist that can hinder the effectiveness of these assays. It is essential to address these challenges to improve assay outcomes and enhance our comprehension of protein stability.
Limitations of Current Techniques
While several methodologies for protein stability assessment are available, they all carry inherent limitations.
- Sensitivity: Some techniques may not be sensitive enough to detect subtle changes in protein stability. For instance, methods like circular dichroism can provide insight into secondary structure changes, yet they may not reveal detailed tertiary structural alterations.
- Time Efficiency: Many stability assays can be time-consuming. Techniques like differential scanning calorimetry, although accurate, often require extensive preparation and lengthy data collection. This can delay critical decision-making phases in drug development and protein engineering projects.
- Environmental Effects: Assays conducted in vitro may not accurately represent physiological conditions. The influence of cellular environments, including pH, temperature, and crowding effects, are often overlooked. This discrepancy raises questions about the relevance of laboratory findings to real-world scenarios.
- Sample Handling: Protein stability can vary significantly based on how samples are handled before and during the assay. Factors like light exposure, temperature fluctuations, and even buffer conditions can lead to artifacts in data.
These limitations highlight the need for continuous development in assay techniques, ensuring reliable and reproducible results that are critical in research and industry contexts.
Future Directions for Improvement
Overcoming the challenges associated with protein stability assays requires innovation and refinement of current methodologies. Researchers can focus on several key areas for improvement:
- Integration of Multi-Modal Approaches: Combining different stability techniques can provide a more comprehensive understanding of protein stability. For instance, integrating thermal shift assays with fluorescence spectroscopy may offer insights into both thermal stability and conformational changes.
- Adaptation to Physiological Conditions: Developing assays that better mimic in vivo conditions will enhance the relevance of data obtained. Techniques that consider ion strength, crowding, and cellular matrices could reveal more about protein behavior in a biological context.
- Automation and High-Throughput Screening: Increasing automation in protein stability assays can reduce time and labor demands. High-throughput screening methods will allow simultaneous testing of multiple protein variants, expediting the discovery process in drug development and protein engineering.
- Data Normalization and Standardization: Establishing standards for data interpretation across different methods can facilitate better comparisons and validations. It is important to develop universally accepted metrics and protocols to assess protein stability reliably.
In summary, by addressing existing limitations and embracing new methodologies, the field can make substantial progress. These improvements will not only enhance the reliability of protein stability assays but also deepen our understanding of protein behavior, ultimately benefiting research and therapeutic applications.
Finale and Future Perspectives
The examination of protein stability assays reveals their critical role in both research and industrial applications. Understanding how proteins respond under various conditions not only helps in fundamental biological studies but also informs therapeutic developments and protein engineering initiatives. The insights gained from these assays guide researchers in optimizing protein formulations and advancing new drugs.
Summary of Key Points
- Mechanisms of Protein Stability: The stability of proteins is influenced by multiple factors including temperature, pH, and the presence of stabilizing or destabilizing agents. Recognizing these factors is essential for accurate experimental design.
- Key Methodologies: Techniques such as Differential Scanning Calorimetry and Circular Dichroism Spectroscopy allow precise measurements of protein stability. Each method has its strengths, providing unique insights into protein behavior.
- Data Interpretation Challenges: Analysts must be cautious while interpreting stability assay results. Misinterpretations can lead to incorrect conclusions, affecting downstream applications in drug development or therapeutic design.
- Future Directions: As the field evolves, there’s a push towards more high-throughput stability assays. Innovations in technology are poised to enhance the sensitivity and specificity of these assays, potentially leading to breakthroughs in understanding protein dynamics.
Implications for Research and Industry
The ramifications of protein stability assays extend far beyond academic labs. In the pharmaceutical industry, knowledge of protein stability directly influences the formulation strategies for biologics. Stability assessments ensure that therapeutic proteins maintain their efficacy during storage and administration. They also assist in the characterization of protein-drug interactions.
Research initiatives benefit from improved accuracy in understanding protein behavior under physiological conditions. Such knowledge drives advancements in protein engineering, allowing for the design of proteins with better therapeutic properties. Furthermore, these assays contribute to regulatory compliance, ensuring product safety and efficacy.
As research and industry demands grow, new methodologies and technologies must be embraced. The collaborative efforts between academia and industry will facilitate the development of innovative solutions for existing challenges in protein stability assessments. Embracing these future perspectives ensures that the field remains dynamic and responsive to the evolving landscape of biochemical and biophysical research.