Exploring Q Column Ion Exchange Mechanisms and Uses
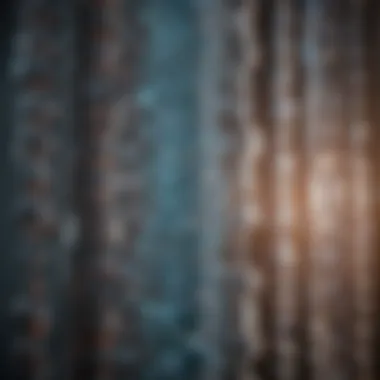
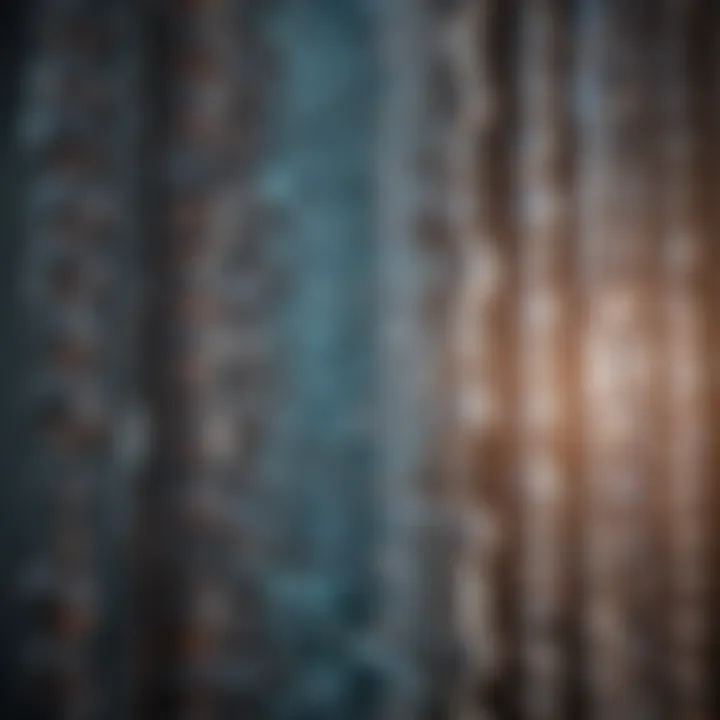
Intro
In the world of biochemical research, efficiency and precision are paramount. Q column ion exchange technology emerges as a frontrunner in the realm of purification processes. This method hinges on the interaction between charged ions, allowing for the selective isolation of molecules. Whether in a laboratory setting or a large-scale industrial application, the ability to separate specific components from a mixture can drastically influence the success of research outcomes and product quality.
The underlying mechanics of Q column ion exchange are rooted in electrostatic interactions and the properties of various ionic species. By manipulating these factors, researchers can achieve highly targeted separations. Understanding these intricate dynamics reveals the significance of Q column ion exchange not just as a laboratory tool but as a critical mechanism across various scientific disciplines.
As we delve deeper into this article, we'll explore the operational principles of Q column ion exchange, review key applications across industries, and discuss how this technology is shaping future advancements in biochemical research. The aim is to provide a comprehensive overview that caters to the intellectual pursuits of students, researchers, educators, and professionals engaging with biochemical sciences.
Intro to Q Column Ion Exchange
Q column ion exchange technology stands as a crucial player in the world of biochemical research and industrial practices. By enabling the targeted purification and separation of molecules, it enhances both efficiency and specificity in diverse applications. The significance of this technology extends beyond mere laboratory use; it filters through various sectors, from pharmaceuticals to environmental science, illustrating its versatility and profound impact.
Understanding the mechanics behind Q column ion exchange is foundational for anyone interested in advanced biochemistry. This section will delve into the essence of this technology, focusing on its definition, historical significance, and operational insights. The benefits of employing Q column ion exchange are manifold, especially when you consider how it tackles the challenges faced in purification processes. Key aspects include its capacity to isolate proteins, small molecules, or larger complexes with fine precision, thus translating into higher purity levels and better yields in research and production settings.
Definition and Overview
The concept of Q column ion exchange revolves around the principle of electrostatic interaction between charged ions. Simply put, it employs a stationary phase that has a positive charge, allowing for the capture of negatively charged ions from the mobile or liquid phase. This mechanism forms the backbone of Q column ion exchange and exemplifies how charge dynamics can be harnessed for separation processes.
In practical terms, this process works effectively for varying molecular weights and sizes, making it particularly useful in protein purification, enzyme isolation, and more. Its flexibility allows it to tackle complex samples that may vary greatly between runs, a feature not often available with simplified chromatography methods.
Historical Evolution
The history of ion exchange technology dates back a few decades, but the journey has been quite substantial. Initially developed in the early 20th century, ion exchange began to pave the way for more intricate biochemical applications post World War II, particularly when demand increased for efficient methods of purifying biomolecules.
Early iterations were rudimentary and often faced limitations in resolution and selectivity, which hampered broader usability. However, the late 20th century saw rapid advancements in polymer chemistry and support material development. This period was marked by profound innovations that allowed scientists to fine-tune the properties of resins and columns, shedding light on novel applications across various fields.
As techniques evolved, researchers began to recognize the immense potential for Q columns in complex bioprocessing tasks. This laid the groundwork for its integration into pharmaceutical developments and diagnostic labs, where purified proteins and biomolecules are vital. Today, Q column ion exchange remains a cornerstone of biochemistry and biotechnology, continually adapting to meet the demands of modern science.
"Technology, in its most impactful form, not only solves problems; it redefines the boundaries of what is possible."
The interplay of historical advancements and continuous innovations underlines the relevance of Q column ion exchange technology in today's scientific landscape. With this backdrop, one can appreciate its importance in various applications, making it a focal point for study and exploration.
Fundamentals of Ion Exchange Theory
Understanding the fundamentals of ion exchange theory is crucial when diving into the world of Q column ion exchange. This theory lays the groundwork for how substances interact at a molecular level, making it possible to separate and purify biomolecules. Ion exchange is essentially the process by which ions from a solution are exchanged for other ions of a similar charge, typically occurring in a medium that retains these ions. Whether in a lab setting or in larger industrial applications, comprehending these basics enables researchers and practitioners to optimize their processes for better results.
One major benefit of mastering ion exchange theory is the ability to select and customize the right type of resin and column setup. Factors such as ionic strength, pH, and temperature all play a significant role in the efficiency of ion exchange. Being aware of these parameters can lead to the avoidance of common pitfalls, like poor resolution during separation processes. Thus, the theory doesn't just inform choices; it shapes the very frame of success in biochemical experiments.
Basics of Ion Exchange Mechanisms
At its core, ion exchange relies on the principles of charge balance and electrostatics. When molecules dissolve in a solvent, they can become charged. In Q column ion exchange, positively charged ions tend to bind to negatively charged resin and vice versa. This creates an equilibrium where the fixed site on the resin can either hold or release specific ions, depending on various conditions.
For instance, think of a magnet attracting metal filings; similarly, charged molecules attract or repel each other based on their charges and the surrounding environment. The ability of resin to selectively bind certain ions means that when a mixture flows through the column, the desired molecules chart a distinct pathway compared to the others. This selectivity is key in applications ranging from protein purification to vaccine development.
Types of Ion Exchange Columns
There are two primary categories of ion exchange: anionic and cationic exchange. Each type has its unique features and is suited for different applications.
Anionic vs Cationic Exchange
In anionic exchange, negatively charged ions are attracted to positively charged sites on the resin. This is particularly useful when dealing with biomolecules that are often anionic, like nucleic acids. Conversely, cationic exchange involves positively charged ions binding to negatively charged resin sites. This type of exchange is beneficial for proteins, which usually have a net positive charge under certain conditions.
The key characteristic of this division is its specificity to the types of ions involved. Anionic systems are often preferred for isolating larger biomolecules like antibodies, while cationic systems excel in polishing smaller proteins. Moreover, this specificity translates into practical benefits—like yielding higher purity levels and better recovery rates.
Advantages and Disadvantages
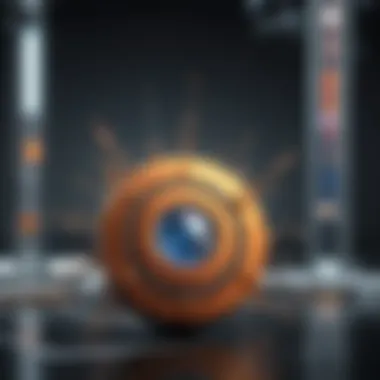
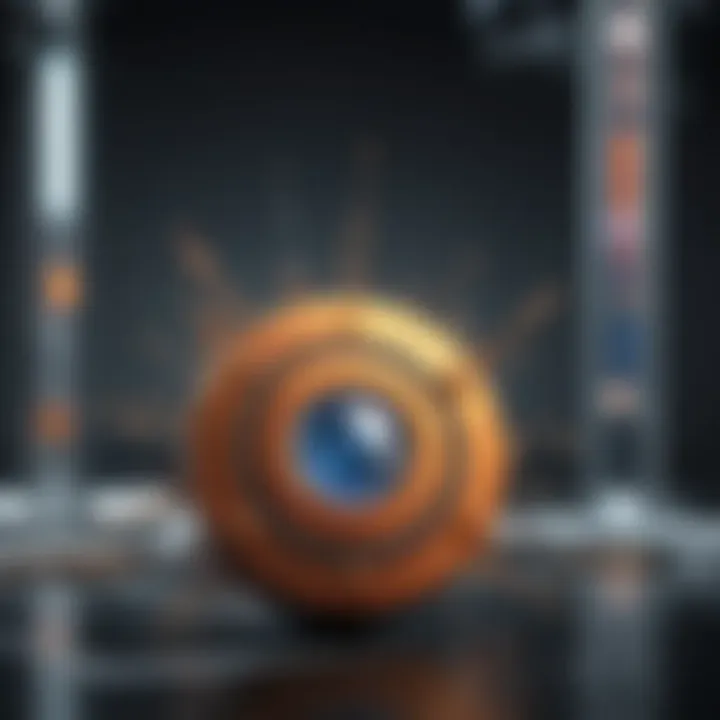
While both techniques carry distinct features, they also have unique challenges. Anionic exchanges can sometimes suffer from lower binding capacities due to the competitive binding of other anions present in the mixture. Meanwhile, cationic systems might require strict pH control to maximize their efficiency. Recognizing these factors can guide researchers in choosing the proper type of exchange for their specific needs.
Resins and Support Materials
Resins serve as the backbone of ion exchange columns. They come in various forms and compositions, each tailored for specific ion exchange processes. Understanding the types of resins available is paramount for successful applications in Q column ion exchange.
One key characteristic of modern resins is their functionalization. Many resins are chemically modified to enhance their interaction capabilities with target ions. This modification is what makes some resins particularly fit for high-throughput applications, while others are better suited for precision tasks.
Advantages and Disadvantages
In terms of unique features, many contemporary resins boast high stability and resistance to harsh chemical environments, therefore, broadening their applicability. However, careful selection is still necessary, as some resins may exhibit non-specific binding, leading to unwanted interactions that could compromise the integrity of the target molecules in the separation process. This balancing act of benefits against potential drawbacks is essential for anyone looking to delve into Q column ion exchange for practical or research purposes.
Understanding the fundamentals of ion exchange mechanisms and the specific types of columns available is key to harnessing the full power of Q column ion exchange technology, enabling the efficient separation and purification of complex biomolecules.
Operational Principles of Q Column Ion Exchange
Understanding the operational principles of Q column ion exchange is central to grasping its value in biochemical applications. This technology hinges on the interplay of charged particles, facilitating the separation and purification of biomolecules. The significance here is twofold: not only does it enhance the efficiency of separations, but it also elevates the specificity with which target molecules can be isolated from complex mixtures. In this section, we will explore the intricacies of its working mechanism and the critical parameters that influence its performance, offering insight into the advantages it brings to various applications.
Working Mechanism
The working mechanism of a Q column is fundamentally about how ions interact within the column. Here, molecules are attracted or repelled based on their charge, allowing for targeted separations.
Ionic Interactions
Ionic interactions form the backbone of Q column ion exchange, providing a reliable means for achieving selectivity in purification processes. The peculiar nature of these interactions lies in how oppositely charged species come together. This affinity is not merely a random occurrence; it’s purposefully manipulated to enhance separation efficacy. One significant characteristic of ionic interactions is their reversible nature, allowing for the elution of bound ions from the resin without altering its overall integrity. In practical terms, this often leads to higher yields during purification.
A unique feature that stands out is the durability of these resin materials. Once established, they can be reused multiple times without substantial loss in performance, which is a considerable advantage over alternative methods that may require frequent replacements. However, it’s also important to recognize potential downsides, such as the vulnerability of resin to fouling from impurities, necessitating regular maintenance.
Elution Techniques
Next, let’s look at elution techniques, which are essential for detaching the purified molecules from the Q column. These approaches essentially dictate how effectively the bound ions can be released for further analysis or use. A noteworthy aspect is the variety of techniques available, such as gradient elution or stepwise elution. Each of these plays a critical role in refining the purification process.
Gradient elution, for instance, involves gradually changing the ionic strength or pH of the elution buffer. This method is particularly advantageous for separating different biomolecules based on their varying affinities for the resin. By modifying conditions, the elution can be finely tuned, allowing certain proteins to elute at specific times, thus enhancing the resolution.
The unique characteristic of elution techniques lies in their adaptability. Depending on the requirements of the separation, different techniques can be employed or modified to achieve the desired results. While this flexibility is beneficial, it also requires a certain level of expertise to select the most effective elution method for particular substances.
Key Parameters Affecting Performance
The performance of Q column ion exchange is influenced by various key parameters. Understanding these factors is vital for optimizing the separation process and achieving the best outcomes.
pH Levels
The pH level is a major factor impacting ion exchange processes. In Q columns, pH can significantly alter the charging state of biomolecules, affecting their retention time and overall interaction with the resin. This attribute makes pH a critical parameter for control. A slightly acidic or basic environment may lead to distinct outcomes in terms of retention and elution of target molecules. For instance, proteins may exhibit different binding affinities depending on their net charge at various pH levels.
The unique aspect of monitoring pH is its straightforward method of adjustment. Small changes can have noticeable effects on the outcome. Nonetheless, it’s a double-edged sword, as maintaining an optimal pH can be challenging in complex samples.
Ionic Strengths
Next, we have ionic strengths in the solution, which also plays a crucial role. The ionic strength can influence the electrostatic interactions between the resin and the biomolecules, thus directly impacting the ion exchange efficiency. Higher ionic strengths generally lead to reduced binding of proteins, as the competitive ions in the solution can outcompete the target biomolecules for binding sites on the resin.
This makes ionic strength a significant consideration in protocol development, as adjusting it can either optimize or impair separation outcomes. A unique attribute of ionic strength is its ability to be finely calibrated, making it possible to tailor the conditions for specific applications. However, one must be cautious; a poorly chosen ionic strength can result in suboptimal yields or resolution.
Flow Rates
Lastly, flow rates through the Q column can significantly affect performance. A slower flow rate typically allows for greater interaction time between the biomolecules and the resin, enhancing the chances of effective binding. Conversely, flowing too quickly can deprive the molecules of sufficient time to interact, leading to poor separation outcomes.
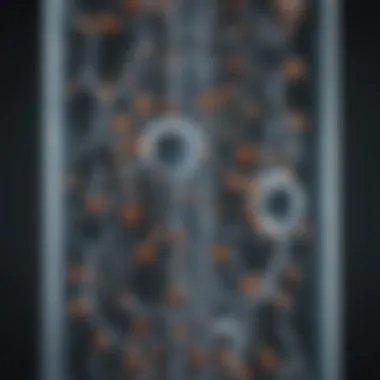
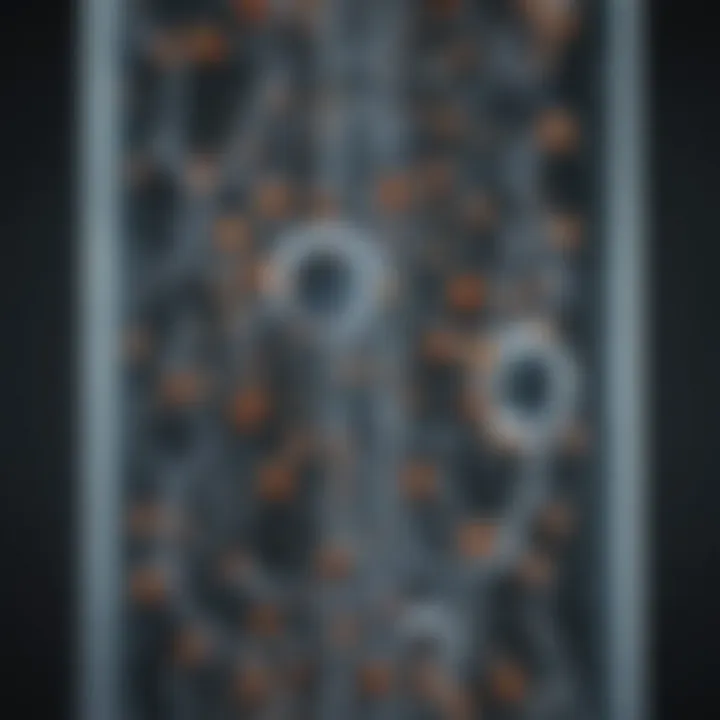
Another noteworthy element is that adjusting flow rates allows for operational flexibility; researchers can strategically experiment with different speeds to find the sweet spot for optimal purification depending on the specifics of the sample being processed.
In summary, by understanding and manipulating these parameters—pH levels, ionic strengths, and flow rates—the efficiency of Q column ion exchange can be maximized, paving the way for more effective biochemical purification strategies.
Applications in Biochemistry and Biotechnology
The application of Q column ion exchange in the realms of biochemistry and biotechnology is greatly significant. This technique is not just a lab gimmick but a foundational tool used in various processes. It stands tall, facilitating the purification of complex biological molecules which is crucial for many applications ranging from research to industrial manufacturing.
The process emphasizes specificity and efficiency, allowing researchers and professionals to isolate proteins, enzymes, and antibodies with remarkable precision. As a result, many industries rely heavily on this method to address challenges within drug development and other biochemical applications. Let's break down some of its key components.
Protein Purification
Enzyme Recovery
Enzyme recovery through Q column ion exchange is pivotal for many biotechnological applications. The key characteristic here is the affinity of enzymes to their respective substrates and how they can be separated based on their charge. This is particularly beneficial when you want to maintain the functionality of the enzyme post-isolation, as it often ensures that the enzyme retains its activity, which is vital for any downstream processes.
One unique feature of enzyme recovery in this context is the selectivity it provides. By adjusting the parameters like pH and ionic strength, one can optimize the conditions for the recovery process. However, a potential downside could be the need for rigorous testing to ensure that the purity levels are satisfactory, as enzyme activity can sometimes be diminished during the separation.
Monoclonal Antibody Isolation
The isolation of monoclonal antibodies is another significant application of Q column ion exchange. This method gives researchers the ability to harness antibodies that have been designed for specific antigens, thus contributing directly to therapeutic development or diagnostic tools. Its key characteristic is the high resolution in separating antibodies from other proteins, making it a favored approach for labs working with biologics.
What stands out about monoclonal antibody isolation using this method is its adaptability. Researchers can tweak resin types or buffer compositions to cater to various antibodies, optimizing separation conditions effectively. However, challenges might arise when dealing with complex mixtures, where overlapping charge characteristics may lead to less effective separations. Such issues underline the importance of method refinement and validation.
Vaccine Development
In vaccine development, Q column ion exchange plays a crucial role by assisting in the purification of vaccine antigens or other essential biomolecules. By employing this method, scientists can efficiently isolate high-purity components, which is pivotal in ensuring vaccine efficacy and safety. The ability to manipulate ionic interactions translates into better yield and functional activity of the purified product.
Participating in Metabolomics Studies
Within the field of metabolomics, Q column ion exchange is increasingly being utilized as a tool for profiling metabolic reactions and pathways. This not only aids in understanding biological processes but also supports the identification of biomarkers for diseases. Applying this technology can reveal metabolic changes under various conditions, granting significant insights for both academia and pharmaceutical companies alike.
Challenges and Limitations
When we delve into the realm of Q column ion exchange, it’s important to recognize the hurdles that researchers and practitioners face. Understanding the challenges and limitations isn't just an academic exercise; it's essential for optimizing performance and ensuring the accuracy of results across biochemical processes. The issues at play can significantly impact the effectiveness of ion exchange technology and should never be taken lightly.
Column Fouling and Contamination
One of the foremost concerns in using Q columns is column fouling. This deterioration often stems from the accumulation of unwanted substances, which can be particulate matter or proteins that adhere to the column's packing material. When this occurs, performance can drop like a lead balloon.
As fouling progresses, the efficiency and resolution of the ion exchange process may decline, resulting in poorer separation of the target analytes. This can lead to some serious setbacks in research, particularly in protein purification where precision is paramount. If the targeted protein makes its grand escape alongside contaminants, scientists may find themselves in hot water, struggling to isolate their desired product.
For instance, if a lab is working with a complex mixture of enzymes and contaminants that tend to stick around, they might find after several runs that their yields have taken a nosedive. Implementing regular cleaning protocols, as well as choosing high-quality resins and optimizing operating conditions, becomes crucial here.
"Preventing fouling requires constant vigilance and an understanding of your sample. Ignoring this can lead to time and resource wastage that no one wants on their hands.”
Resolution and Selectivity Issues
Resolution and selectivity are two sides of the same coin when it comes to the effectiveness of Q column ion exchange. The ability of a method to discriminate between different ions is what ultimately determines its utility in practical scenarios. However, achieving high resolution is often easier said than done.
For example, if you’re trying to separate two similar protein species that differ in charge only slightly, you could run into a classic case of resolution issues. Using a Q column under less-than-ideal conditions can result in overlapping peaks in chromatograms, which complicates data analysis and can lead to inaccuracies.
Selectivity, in simpler terms, is about how well a method can distinguish one compound from another. Complications can arise when the ionic environment changes. Variations in pH or ionic strength can drastically change the binding interactions, making something that was previously easy to separate become a real puzzle.
To address these challenges, researchers might need to fine-tune several factors, including adjusting buffer compositions or experimenting with different column packing materials. Utilizing advanced analytical techniques is another approach to enhance both resolution and selectivity in future experiments.
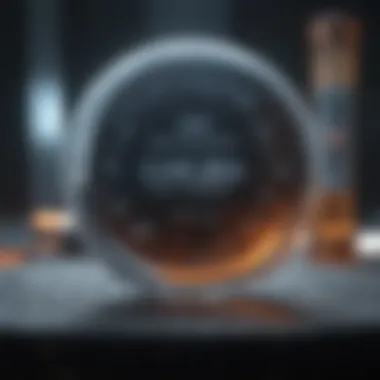
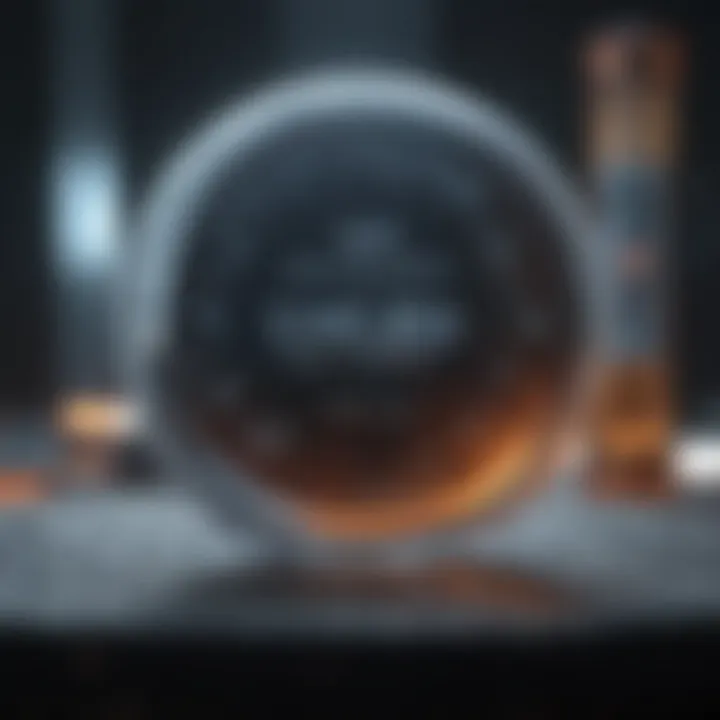
Recent Advances in Q Column Ion Exchange Technology
Recent advances in the domain of Q column ion exchange technology have significantly enhanced both the efficiency and applicability of this well-established method. Innovations in this arena are crucial as they address complex challenges while opening doors for novel research avenues. The intertwining of technological advancements with biochemical processes not only boosts productivity but also fortifies the specificity and resolution of separations, making it a vital topic of consideration in contemporary research.
Novel Resin Materials
A significant stride in Q column technology stems from the introduction of novel resin materials. Traditional resins have served their purpose well over the decades, yet they are often limited by issues such as low binding capacities or susceptibility to fouling. Modern advancements aim to overcome these limitations.
Many cutting-edge resin materials are now engineered to exhibit enhanced ionic capacities, ensuring a more effective binding of target molecules. For instance, a multi-functional resin promotes specific interactions with a wider array of biomolecules, where fine-tuning of chemical properties enables tailored interaction models. Such innovation plays a pivotal role in fields like protein purification and enzyme recovery. It allows for the separation of highly similar proteins, which was previously an uphill battle.
Some notable resins in recent studies include those developed with monodisperse polymer technology. These offer uniformity in particle size, drastically improving flow dynamics within columns. Additionally, incorporation of functionalized ligands provides charge-specific offers, unlocking more avenues for targeted separations.
"Advancements in resin materials are not just about better performance, it's about transforming the future of biochemical separations."
Hybrid Ion Exchange Systems
Another highlight in the recent developments is the emergence of hybrid ion exchange systems, blending the strengths of different methods. This approach leverages the principles of ion exchange alongside other separation technologies, resulting in enhanced operational efficiency.
For example, combining chromatography with ion exchange eliminates many limitations presented by each method when used in isolation. In practice, a hybrid system may utilize membrane technologies for initial filtering, which is then followed by Q column ion exchange for final purification. This synergy not only shortens the processing time but also significantly increases the overall yield of desired products.
Moreover, the adaptability of hybrid systems allows for real-time adjustments based on the sample composition, thus ensuring optimal performance tailored to specific needs. These systems underscore the continuous evolution of ion exchange techniques, making the technology not just relevant, but indispensable in modern biochemical applications.
In summary, the recent advances in Q column ion exchange technology, particularly in novel resin materials and hybrid systems, mark a significant enhancement in the field. This innovation not only addresses previous drawbacks but also broadens the scope of applications, propelling biochemical research to new heights.
Future Directions and Research Opportunities
The field of Q column ion exchange is continuously evolving, and its future promises exciting possibilities. As biochemical research expands, there are crucial elements worth exploring in the realm of ion exchange technologies. This section looks at the emerging trends and the integration of advanced technologies such as artificial intelligence, which can reshape practices in this field for better efficiency and effectiveness.
Emerging Trends in Ion Exchange Applications
Recent years have seen a surge in innovative applications for Q column ion exchange, breaking ground in various scientific domains. Here are some notable trends:
- Personalized Medicine: There is an increasing focus on customizing treatments based on individual patient profiles. Q column ion exchange could play a vital role in tailoring therapies that are specific to biochemical markers in patients.
- Sustainable Practices: The demand for eco-friendly production methods has prompted researchers to optimize ion exchange processes. For example, employing biomaterials in resins could be a more sustainable solution without sacrificing performance.
- Nanotechnology Integration: Utilizing nanomaterials can enhance the selectivity and efficiency of ion exchange processes. This could lead to reduced resource usage while maintaining high throughput in various applications such as protein purification and biosensor development.
The potential for these trends to significantly impact efficiency and precision in research and industrial contexts is immense. With ongoing research, we may witness even more innovative applications solidifying the importance of Q column ion exchange.
Integrating AI in Ion Exchange Processes
Artificial intelligence is the new buzzword in many scientific fields, including ion exchange. Its integration presents numerous opportunities and benefits:
- Predictive Modeling: AI can analyze large datasets to predict the outcomes of various ion exchange scenarios. This means optimizing settings preemptively, which saves time and resources.
- Dynamic Process Adjustments: With AI systems, real-time monitoring of ion exchange processes can be deployed. This means immediate adjustments can occur, enhancing the performance and efficiency of Q columns during operations.
- Enhanced Data Analysis: Complex data generated from ion exchange experiments can be distilled using AI, providing clearer insights and facilitating more informed decision-making in research.
Given the ongoing shift towards digitization in laboratory practices, the integration of AI in ion exchange processes represents a pivotal transformation. Embracing this change could lead to breakthroughs that redefine the capabilities of Q column ion exchange.
"The future of Q column ion exchange lies not just in its current applications but in its potential to adapt to scientific advancements and societal needs."
Overall, understanding and initiating research in these areas is imperative for those looking to stay ahead in biochemical research and industrial applications. As discoveries unfold, they will undoubtedly enhance the efficiency and relevance of Q column ion exchange in tackling contemporary challenges.
The End
The final takaway from our exploration of Q column ion exchange technology binds together its essential role in biochemical purification and separation processes. The countless applications in laboratories and industries highlight this method's versatility. The importance of understanding the mechanics of Q column ion exchange cannot be overstated; it facilitates efficient separations and can adapt to various biochemical contexts. Moreover, it serves as a backbone for constant innovation in research methods, aimed at enhancing results in areas like protein purification and vaccine development.
In terms of benefits, here are some noteworthy points to consider:
- Efficiency: Q column ion exchange is especially effective at isolating specific molecules based on charge interactions, which is critical for achieving high purity levels.
- Adaptability: With advancements in materials and methods, this process is increasingly refined, allowing for novel applications across different fields of research.
- Cost-effectiveness: This method can be more cost-effective than others, particularly when you consider the high efficacy in isolating compounds.
However, it's vital to also acknowledge certain considerations. Increased efficiency can sometimes lead to diminished resolution or selectivity, particularly if there is column fouling or contamination.
In summary, the significance of Q column ion exchange is pronounced and multi-dimensional. Its impact on various scientific domains, especially in contemporary biochemical research, underscored the ongoing need for mastery of its mechanics and applications. As we tread deeper into an era of technological advancements and continuous discovery, the future of Q column ion exchange looks promising, offering new frontiers for researchers and industries alike.
"Understanding the mechanics of this technology allows us to unlock potential innovations in our research endeavors."
In closing, as researchers, educators, and practitioners, grasping these insights will not only aid in practical applications but also inspire continual learning and exploration within our fields.