Exploring the Role of Catalysts in Chemical Reactions
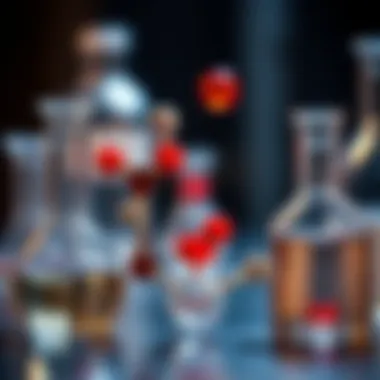
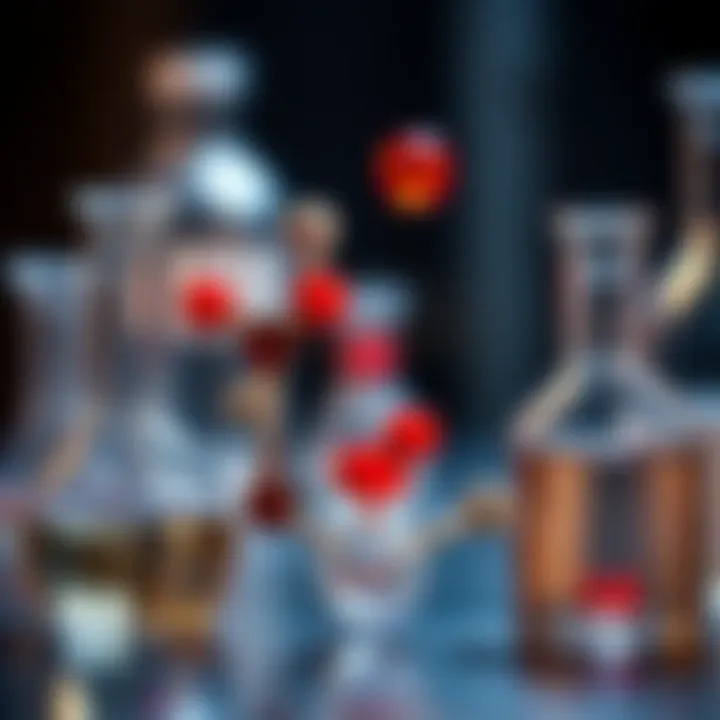
Intro
Catalysts play a pivotal role in chemical reactions, acting as facilitators that can dramatically influence the speed and efficiency of reactions without being consumed in the process. They reduce the energy barrier that reactants must overcome, allowing for faster reaction rates and often enabling reactions to occur under milder conditions than would otherwise be possible. This ability to enhance reaction rates makes catalysts indispensable in both laboratory and industrial settings, where even small improvements in efficiency can lead to significant financial savings or environmental benefits.
The exploration of catalysts stretches beyond simple definitions; it encompasses a vast array of types, mechanisms, and applications that are crucial for various fields. From the catalytic converters in automobiles, which help to reduce harmful emissions, to enzymes in biological systems that accelerate metabolic reactions, understanding catalysts is essential for anyone involved in the sciences. The following sections will delve into the fundamentals, elucidating the underlying principles guiding catalytic activity, discussing the various types of catalysts, and highlighting their importance in both industrial processes and biological systems.
By dissecting these themes, this article aims to foster a robust comprehension of how catalysts function, the nuances of their behavior in chemical reactions, and their overarching significance in advancing both technological progress and scientific discovery.
Preamble to Catalysts
Catalysts play a pivotal role in the realm of chemistry, acting as facilitators that expedite reactions without undergoing permanent change. Their importance cannot be overstated; they are the unsung heroes of chemical processes, often responsible for the efficiency and feasibility of numerous industrial reactions. Understanding catalysts is essential, not only for grasping the foundational principles of chemistry but also for recognizing their expansive applications across various fields including pharmaceuticals, energy production, and environmental sustainability.
To put it simply, a catalyst serves as a bridge that lowers the energy barrier for a reaction, allowing it to proceed at a faster rate than it would otherwise. This enhances productivity in chemical manufacturing, which is often a key consideration in industries seeking to optimize their processes. The exploration of catalysts unfolds a rich tapestry of topics, from their definitions to their historical development, which illustrates how our understanding of these chemical agents has evolved.
Definition of Catalysts
A catalyst can be defined as a substance that increases the rate of a chemical reaction by lowering the activation energy required. This definition is essential as it encapsulates the primary function of catalysts—they facilitate the transformation of reactants into products, often in a manner that circumvents energy-intensive pathways. It’s a bit like having a seasoned chess player guide a novice: the game may still be played, but with the right strategies, it becomes far more accessible.
Catalysts can be categorized into three main types: homogeneous, heterogeneous, and enzymatic. Each type has distinct characteristics and applications, making their study vital for anyone seeking a comprehensive understanding of chemical reactions.
Historical Perspective
Tracing back through the annals of chemistry, the concept of catalysis has a storied history. The term "catalyst" was first introduced in the early 19th century by the chemist Jöns Jacob Berzelius, who recognized the influence certain substances had on chemical reactions. Initially, catalysts were viewed through a somewhat simplistic lens; they were believed to merely provide a surface for reactions to occur. As time marched on and scientific methods advanced, researchers began to uncover the more intricate behaviors of catalysts.
The development of catalysts has paralleled advancements in various industries. For instance, in the late 19th and early 20th centuries, the emergence of catalytic converters revolutionized the automobile industry, significantly reducing harmful emissions and altering environmental chemistry. Fast forward to contemporary times, and catalysts remain at the forefront of research and innovation, particularly in the realm of green chemistry and sustainability.
"Catalysts are not just overlooked aids in reactions; they are at the heart of modern chemical innovation, enabling processes that would otherwise be unfeasible or inefficient."
In summation, the journey into understanding catalysts intertwines a rich history with practical applications, shining a spotlight on their significance in the chemical sciences and beyond. Their study is a gateway to comprehending broader scientific phenomena, emphasizing the role of innovation in making chemistry a more practical and beneficial field.
Types of Catalysts
Understanding the various types of catalysts is crucial for anyone delving into the field of chemistry. Catalysts are classified based on their phases and interaction methods, which influence their efficiency and application in chemical reactions. Each type presents unique advantages—whether it’s in terms of reaction speed, selectivity or operational conditions. Knowing these distinctions not only provides insight into how reactions can be optimized but also helps in tailoring catalytic processes for a wide range of industrial and research applications.
Homogeneous Catalysts
Homogeneous catalysts are those that exist in the same phase as the reactants, often found in solution. These catalysts can facilitate reactions more efficiently due to their uniform composition. A classic example can be seen in the acid-catalyzed hydration of alkenes, where sulfuric acid is used in the same liquid phase as the reactants. The species interact freely at the molecular level, promoting a more effective collision frequency which often leads to faster reaction rates.
Benefits of Homogeneous Catalysts
- Enhanced Mixing: Being in the same phase allows for better mixing and interaction among reactants.
- Selective Reactions: They can be tailored for specific reactions, enhancing selectivity for desired products.
- Simple Recovery: Although recovery from the reaction mixture may present challenges, advancements in separation technologies have begun to ease this issue.
However, homogeneous catalysts often face limitations, such as stability under reaction conditions and potential difficulties in recovery. This can lead to higher operational costs and challenges in larger-scale industrial applications if not managed properly.
Heterogeneous Catalysts
In contrast to their homogeneous counterparts, heterogeneous catalysts exist in a different phase than the reactants—typically solids interacting with gaseous or liquid reactants. An everyday workhorse in this category is the platinum catalyst used in catalytic converters in automobiles. This setup demonstrates how a solid catalyst can promote oxidation and reduction reactions effectively within a primarily gaseous environment.
Importance of Heterogeneous Catalysts
- Robustness: Heterogeneous catalysts generally have greater stability and are less prone to deactivation compared to homogeneous catalysts.
- Easier Separation: Their separate phase allows for straightforward removal from the reaction mixture. This characteristic is particularly beneficial in industrial processes, where catalyst recycling can significantly reduce costs.
- Commercial Viability: Due to their ease of handling and general robustness, heterogeneous catalysts are widely used in petrochemical processes, such as the Haber-Bosch process for ammonia synthesis.
Despite these advantages, limitations such as diffusion limitations can arise, as reactants must travel to the active sites on the catalyst surface. Optimizing the catalyst surface area is key to enhancing its performance.
Enzymatic Catalysts
Enzymatic catalysts, or enzymes, are biological molecules that act as catalysts in various metabolic reactions within living organisms. These catalysts exhibit remarkable specificity and efficiency, often catalyzing reactions at significantly faster rates compared to traditional chemical catalysts. For instance, the enzyme catalase breaks down hydrogen peroxide into water and oxygen quickly, showcasing its vital role in the detoxification process of cells.
Highlights of Enzymatic Catalysts
- Specificity: Enzymes are highly specific, meaning they usually only catalyze one particular reaction type, leading to fewer side reactions.
- Mild Conditions: Many enzymes operate effectively under mild conditions of temperature, pressure, and pH, which can reduce energy costs and improve safety.
- Biocompatibility: Being naturally occurring, enzymes are generally more environmentally friendly, often used in biotechnological applications such as food processing and pharmaceuticals without harsh chemicals.
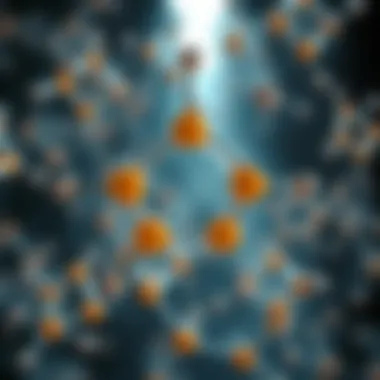
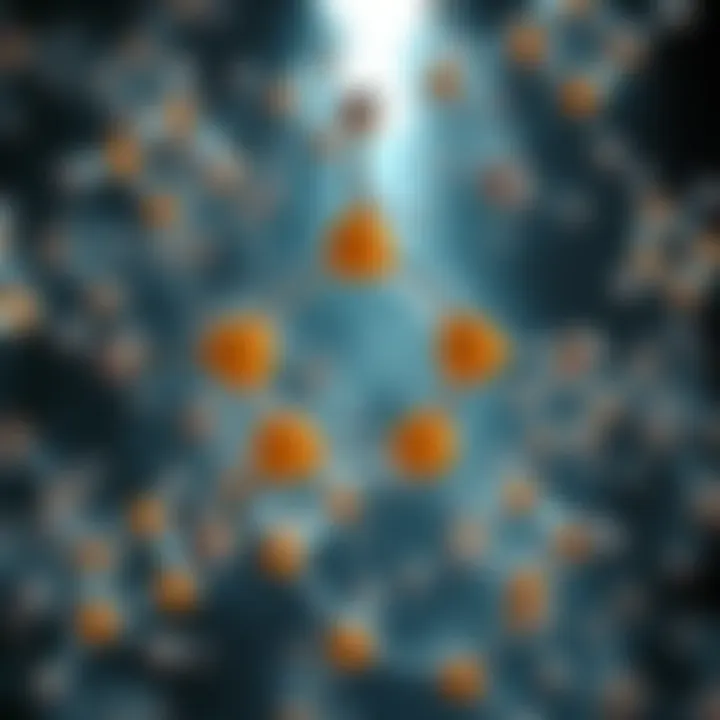
Nevertheless, enzyme activity can be sensitive to environmental factors, often requiring precise conditions for optimal function. This sensitivity means that while they can be extraordinarily effective in controlled settings, scaling up for industrial applications can pose challenges.
“The right catalyst at the right time can be the difference between an inefficient process and a chemical symphony.”
Mechanism of Catalytic Action
The mechanism of catalytic action plays a pivotal role in comprehending how catalysts facilitate chemical reactions. By understanding the intricate dance of molecules during a reaction, scientists can better harness the power of catalysts to drive processes in both lab settings and industrial applications. Catalysts essentially lower the energy barriers that hinder reactions, making them crucial for efficiency in any chemical manufacturing scenario.
Energy Barrier Reduction
One of the fundamental aspects of catalytic action is energy barrier reduction. Every chemical reaction has an activation energy threshold that must be surmounted for it to proceed. Think of it as climbing a hill – if the hill is steep, you need more energy to reach the top. Catalysts provide a way to create a gentler slope, allowing reactants to transition to products with less effort.
Consider this; without a catalyst, the energy input required to initiate a reaction might be so high that the reaction does not occur under normal conditions. For example, in the Haber process, which synthesizes ammonia from nitrogen and hydrogen, the use of iron-based catalysts significantly lowers the activation energy, enabling the reaction to proceed at practical temperatures and pressures. Through this method, industrial production becomes economically viable, supplying ammonia for fertilizers that feed the planet.
Transition States and Intermediates
The idea of transition states and intermediates is another critical component of catalytic mechanisms. During a reaction, molecules do not simply morph from reactants to products. Instead, they pass through a transitional phase where the bonds are in a state of flux. This fleeting moment, when the molecules are neither entirely reactant nor product, is referred to as the transition state.
Catalysts often stabilize these transition states, allowing the reaction to occur more easily. They provide specific environments that reduce the energy associated with these states. For instance, in enzymatic reactions, the enzyme temporarily binds to substrates, lowering the energy needed to form products. The intermediate products can vary widely, depending on the reaction pathway, and understanding these can yield insights into the overall mechanism.
In summary, the mechanisms of catalytic action reveal not just how reactions occur, but also how they can be optimized. Understanding energy barriers, transition states, and intermediates allows chemists to design more effective catalysts, making reactions faster and more economical.
"Catalysts are the unsung heroes of chemistry, quietly doing the work necessary to drive our world's reactions and processes."
Through detailed studies on these mechanisms, we pave the way for innovations in various fields, from pharmaceuticals to environmental science. As we continue to research and explore, the potential for discovering new catalysts or improving existing ones remains vast.
Factors Influencing Catalytic Activity
The catalytic activity of substances isn't just a straightforward affair; it's swayed by various factors that can significantly affect how efficiently a reaction proceeds. Understanding these factors is critical for both theoretical concepts and practical applications in the realm of catalysis. By grasping how temperature, concentration, and pressure interplay with catalysts, chemists can fine-tune reactions to yield desirable products effectively. This knowledge is invaluable across multiple fields, including industrial processes and environmental science.
Temperature Effects
Temperature plays a crucial role in catalytic activity. Generally, as temperature rises, the kinetic energy of the molecules in a reaction also increases. The collisional frequency goes up, and so do the chances of successful interactions between reactants and catalysts. However, there's more to it than simply cranking up the thermostat.
At moderate temperatures, catalysts can accelerate reactions effectively. For example, in the Haber process for synthesizing ammonia, an increase in temperature enhances the rate of reaction but too high a temperature can lead to detrimental side reactions, which result in lower yields of ammonia. Therefore, chemists must find an optimal balance, one that maximizes reaction rates while minimizing unwanted by-products.
It's not just about temperature either; the state of the catalyst and the nature of the reactants can also affect reaction behavior at different temperatures. Some catalysts may lose their effectiveness at higher temperatures, while others can become more active. Thus, it’s paramount to understand the thermal stability of a catalyst when designing a process.
"An increase in temperature may increase the reaction rate, but optimal conditions must be known to maximize yields."
Concentration and Pressure
The relationship between concentration, pressure, and catalytic activity is equally significant. Higher concentrations of reactants generally result in increased rates of reaction. This is particularly important for gas-phase reactions where increasing pressure can lead to a higher concentration of gaseous reactants. For instance, in catalytic converters used in automobiles, the pressure of exhaust gases can influence the efficiency of catalysts in facilitating chemical reactions that reduce harmful emissions.
Additionally, for heterogeneous catalysts, where reactions take place at the interface of two different phases, the concentration of reactants can dictate the rate of adsorption onto the catalyst's surface. More reactants often mean more available sites for the reaction to occur, driving the rate up. On the flip side, overcrowding can lead to catalyst saturation, which might stall the reaction progress altogether.
One key consideration is that while increasing concentration and pressure can expedite reactions, there is a limit. Excessive pressure can incur risks such as catalyst damage or altered physical properties of the catalyst, prompting renovations in the design and selection of catalytic materials.
Catalyst Lifespan and Deactivation
The role of catalysts in chemical reactions cannot be overstated; they significantly enhance reaction rates and enable processes to occur under milder conditions. However, like many tools, they do not last forever. When discussing catalysts, one must also address the concept of catalyst lifespan and deactivation. Understanding these factors is crucial for anyone engaged in chemistry, be it in research, industry, or education. This discussion will explore the various types of catalyst deactivation, their underlying causes, and potential regeneration techniques.
Types of Deactivation
Catalysts can lose their effectiveness for several reasons. Deactivation can occur due to physical, chemical, or even thermal changes. Here are some common types:
- Poisoning: This happens when a foreign substance binds to the catalyst's active site, inhibiting its function. An example can be found in automotive catalysts where lead or sulfur compounds may hinder catalytic activity.
- Sintering: As catalysts undergo repeated exposure to high temperatures, they may start to agglomerate, reducing the surface area available for reactions. This occurs commonly in heterogeneous catalysts like those used in petrochemical industries.
- Coking: In some catalytic processes, carbonaceous deposits can form on the catalyst, which block active sites and thus decrease efficiency. It is particularly relevant in catalysts used for hydrocarbon reactions.
- Leaching: In processes involving liquid mixtures, certain catalyst components may dissolve into the solution, leading to a decline in activity. Precious metal catalysts are often vulnerable to this.
Understanding these types can aid chemists and engineers in designing more robust catalytic systems.
Regeneration Techniques

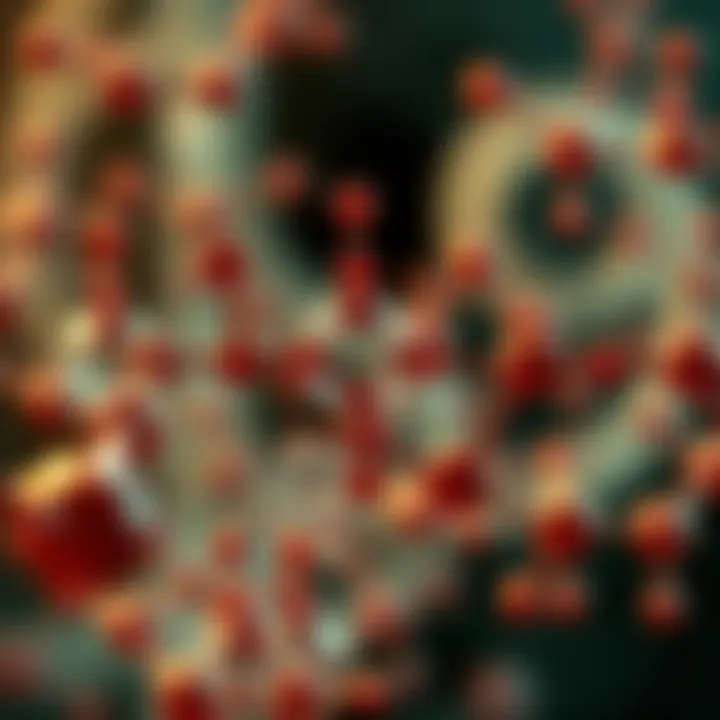
Once a catalyst has been deactivated, efforts can be made to restore its efficiency. The following are some techniques widely utilized in various industries:
- Thermal Regeneration: This technique typically involves heating the catalyst to temperatures high enough to burn off any carbon deposits or contaminants. It employs controlled conditions to avoid excessive damage to the catalytic material.
- Chemical Treatment: In some cases, washing the catalyst with specific solvents or reactants can remove poisons or contaminants. For example, washing with an acidic solution can help to eliminate unwanted metal ions that may be blocking active sites.
- Reactivation: Some catalysts can undergo a more complex reactivation process, which involves modifying their structure to restore functionality—an often complicated procedure that can require a deep understanding of the catalyst’s chemistry.
- Replacement: In scenarios where regeneration proves impractical, replacing a catalyst with a new one may be the only viable solution. While this incurs additional costs, newer catalysts may offer improved performance or longevity.
“Catalysis is not just about speeding things up; it's also about ensuring that what we use is efficient over time.”
Cumulatively, these techniques not only extend the lifespan of catalysts but also contribute to sustainable practices in chemistry, enhancing both economic and environmental outcomes.
Kinetics of Catalysis
Understanding the kinetics of catalysis is fundamental in chemistry as it delves into how catalysts affect the rates of chemical reactions. Catalysts play a pivotal role in altering the speed at which reactions occur without being consumed in the process. This dynamic interaction highlights their significance not just in laboratories but also in various industrial applications.
When analyzing catalysis, one must consider how rate laws come into play. A rate law quantifies the speed of a reaction in relation to the concentration of reactants and the influence of the catalyst. Essentially, it provides the mathematical framework that allows chemists to predict how adjustments in conditions—like temperature or pressure—can affect the rate of a reaction. The relationship can often be quite complex, especially when dealing with heterogeneous catalysts, where the surface area and the nature of the material can drastically influence reaction rates.
Moreover, understanding reaction mechanisms is crucial. Knowing the step-by-step process of how a catalyst interacts with reactants allows scientists to visualize and optimize conditions for specific outcomes. This detailed knowledge leads not only to improved efficiency in chemical processes but can also minimize waste and reduce costs associated with production.
Rate Laws and Catalysis
Rate laws describe how rapidly a chemical reaction proceeds and how this rate is influenced by various factors. When catalysts are involved, they often lower the energy barrier for reactions, which is key to understanding their impact on the rate law. In general, the reaction rate increases as the concentration of reactants rises, and when a catalyst is applied, this relationship can become even more pronounced.
Key Points:
- The rate law can be expressed mathematically as:r = k[A]^m[B]^n
Where:
- r = reaction rate
- k = rate constant
- [A] and [B] = concentrations of reactants
- m and n = reaction orders
A catalyst specifically alters the rate constant (k) while keeping the value of m and n constant. This alteration is crucial during reactions catalyzed by metals such as platinum, which are vital in processes like petroleum refining.
Moreover, applying your knowledge of rate laws can help in designing catalytic systems. For instance, when engineers design a reactor for a specific chemical process, they need to take into account the kinetic parameters to predict the most efficient configuration.
Catalytic Cycles
Catalytic cycles are sequences of events that illustrate how a catalyst facilitates a reaction. These cycles can be visualized as closed loops where intermediates are formed and eventually resolved back into the catalyst and product. The importance of understanding these cycles is multi-fold.
- Identification of Intermediates: By mapping out the steps in a cycle, researchers can identify transient species that could be potential targets for modifying the catalyst to improve efficiency.
- Optimization Process: Knowledge of the catalytic cycle allows chemists to tweak conditions or components, such as catalyst concentration, to enhance performance.
- Application in Biocatalysis: Many enzymatic reactions can be explained through similar cycles, illustrating the relevance of this concept not just in synthetic chemistry but also in biological systems.
For instance, the catalytic cycle in an enzyme may involve substrate binding, product formation, and subsequent release of the product, with the enzyme returning to its original state, ready to catalyze further reactions. Understanding these cycles often leads researchers to discover more efficient pathways for catalysis.
In summary, the study of kinetics in catalysis underscores the intricate dance of molecules at play during chemical reactions. It equips scientists with the tools needed to understand and manipulate the very fabric of chemical interactions, unlocking pathways that can lead to innovation across multiple industries.
With these principles, the realms of both industrial and biological chemistry can benefit immensely, boosting efficiency and sustainability in chemical processes.
Industrial Applications of Catalysts
Catalysts play a pivotal role in various industrial processes. Their ability to lower the energy required for chemical reactions means they not only enhance efficiency but also contribute significantly to the economic viability of operations. Without these agents, many chemical reactions would be prohibitively slow or would require conditions that are impractical or too costly to achieve. Thus, understanding industrial applications offers insights into how catalysts shape production, efficiency, and sustainability in chemistry.
Catalysis in Petrochemical Processes
The petrochemical industry heavily relies on catalysts to turn crude oil into useful chemicals, fuels, and materials. Key processes, such as cracking and reforming, are catalyst-driven, ensuring high yields of desired products while minimizing waste.
- Cracking: This process breaks larger hydrocarbon molecules into smaller, more useful ones like gasoline. Catalytic cracking uses solid catalysts like zeolites, which enable these reactions at lower temperatures and pressures than thermal methods.
- Reforming: Catalytic reforming transforms naphtha into high-octane gasoline components and valuable aromatic compounds. Platinum-based catalysts are often employed, showcasing their ability to rearrange molecular structures effectively.
The efficiency of these processes hinges on the catalysts' longevity and activity. The ability to handle harsher operating conditions without significant degradation means higher profitability for industries. Utilising catalysts is less reminiscent of a simple chemistry application and more like fine-tuning an engine for maximum performance, where the end goal is to maintain product quality while cutting down on by-products.
Catalysts in Environmental Chemistry
In recent years, environmental concerns have steered industrial practices towards more sustainable methods. Catalysts serve as game-changers in addressing pollution and promoting cleaner manufacturing processes.
- Automotive Catalysts: Catalytic converters in vehicles significantly reduce harmful emissions. These devices use platinum, palladium, and rhodium as catalysts to convert toxic gases like carbon monoxide and nitrogen oxides into less harmful substances. The ongoing advancements aim at improving the efficiency of these converters, thereby reducing their environmental footprint further.
- Waste Treatment: Catalysts also play a key role in wastewater treatment, facilitating the breakdown of pollutants. For instance, photocatalysts can degrade organic toxins in water, making it cleaner and safer.
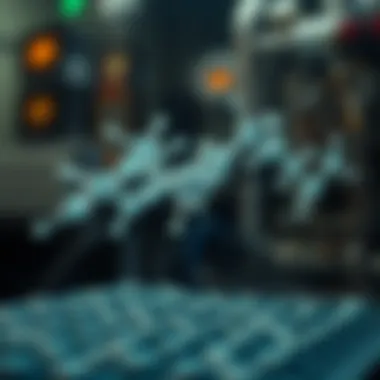
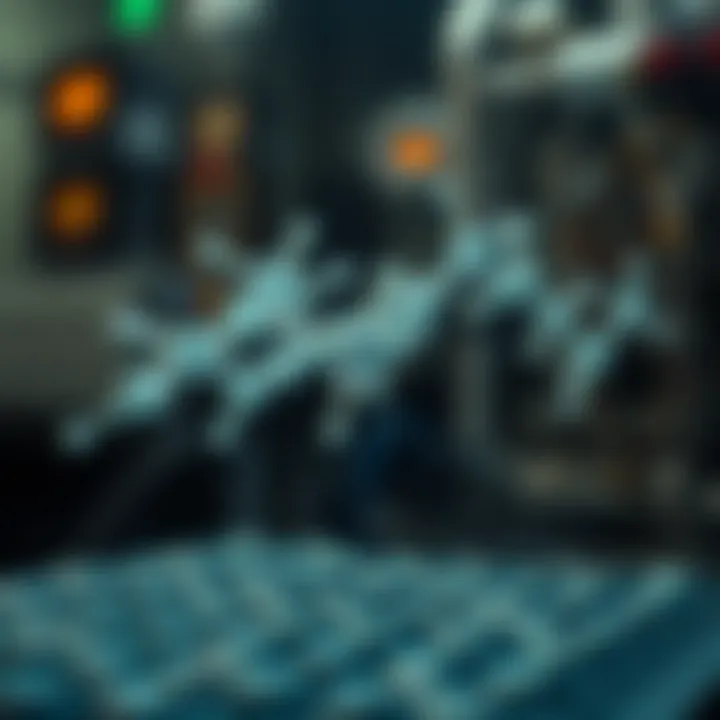
Successful implementation of catalysts in environmental applications underscores their dual role: boosting efficiency while safeguarding our planet's health.
Biocatalysts and Biotechnology
Biocatalysts, primarily enzymes, play a pivotal role in many biological processes. In the realm of biotechnology, they serve as a key tool that enhances the efficiency and specificity of chemical reactions. The significance of biocatalysts lies in their ability to operate under mild conditions, which often means lower energy requirements and reduced environmental impact compared to traditional catalytic processes. These attributes make biocatalysis appealing for a range of applications, especially in sustainable practices.
One notable advantage of utilizing biocatalysts is their high specificity. Enzymes can selectively catalyze reactions, which reduces the occurrence of by-products—a challenge that sometimes afflicts chemical catalysts. This selectivity often leads to simpler and more efficient purifications, ultimately lowering costs and improving yields in various processes. Enzymes often work in aqueous environments, making them particularly well-suited for reactions where conditions need to mimic those found in nature.
The integration of biocatalysts into biotechnology also supports the growing trend toward sustainable methods in chemical manufacturing. As society turns its focus to minimizing waste and pollution, employing enzymes can provide greener alternatives. Not only does this reduce harmful by-products, but it also aligns with regulatory requirements and market demand for environmentally friendly processes.
Role of Enzymes in Metabolic Processes
Enzymes are catalysts that facilitate nearly all biochemical reactions that occur within living organisms. They are remarkable in their ability to accelerate reactions at rates many times faster than would occur naturally. Enzymes operate by lowering the activation energy required for a reaction to proceed. For instance, in the case of glucose metabolism, enzymes like hexokinase convert glucose into glucose-6-phosphate. This biochemical pathway is critical for energy production and, subsequently, for sustaining life.
Moreover, the regulation of metabolic pathways often hinges on enzymes. Cells carefully control enzyme concentration and activity, responding to both internal and external factors. This regulation can be crucial; for example, feedback mechanisms can inhibit or activate pathways based on the needs of the cell, thus contributing to overall homeostasis. Without enzymes, the complex web of metabolic interactions ceases to function effectively, highlighting their essential role in maintaining life.
"Enzymes are nature’s catalysts, working day and night to keep the wheels of life turning smoothly."
Applications in Pharmaceutical Development
The pharmaceutical industry harnesses biocatalysts for drug development and production, leveraging their unique properties to synthesize complex molecules. The ability to perform selective transformations in mild conditions makes enzymes valuable for creating specific drug compounds that might be difficult to produce otherwise. For instance, enzymes are used to produce active pharmaceutical ingredients (APIs) through processes that are not only cost-effective but also more environmentally benign.
From synthetic pathways to drug degradation studies, the role of enzymes in pharmaceuticals extends beyond simple synthesis. They can also be employed in the analysis and testing of drug interactions, ensuring safety and effectiveness. Furthermore, enzymes enable the design of prodrugs—medications that are initially inactive until metabolized. This can enhance the drug's bioavailability, moving it swiftly through biological barriers before transforming into its active form.
In summary, biocatalysts, particularly enzymes, stand at the forefront of both metabolic processes and pharmaceutical innovation. They facilitate reactions vital to life and represent a forward-thinking method in drug development, underscoring the potential of biotechnological advancements in shaping the future of chemistry and other related fields.
The Future of Catalysis Research
The landscape of catalysis research is continously evolving, driven by the need for more efficient and environmentally friendly processes. As we delve into the future of this field, several key elements come to the forefront, including the development of novel materials and the rise of sustainable strategies. It's about harnessing innovation to tackle global challenges, from energy consumption to waste reduction.
Emerging Catalytic Materials
Recent years have seen a surge in the investigation of new catalytic materials. These materials are not only more effective but also play a crucial role in reducing the environmental footprint of chemical processes.
One notable advancement is the use of nanomaterials. Due to their enhanced surface area and unique properties, catalysts built on the nanoscale can operate at lower temperatures and pressures, ultimately saving energy. For example, gold nanoparticles have shown remarkable activity in various reactions that traditional catalysts struggle with. This opens up doors to more sustainable pathways in chemical reactions.
Other emerging materials include metal-organic frameworks (MOFs) and covalent organic frameworks (COFs). These structures provide high porosity and tunable properties, making them promising candidates for applications like gas separation and storage. They also offer potential for catalysis in areas such as carbon capture. By tailoring these materials at a molecular level, researchers can significantly improve their catalytic performance, leading to breakthroughs that can benefit multiple industries.
Sustainable Catalysis Strategies
The push for sustainability has made it imperative to innovate within catalysis research. Sustainable catalysis is about ensuring that chemical processes are not just efficient but also beneficial for the environment. This entails using renewable resources and minimizing by-products and waste.
One strategy gaining traction is the use of biocatalysts, which utilize natural enzymes to drive reactions. Enzymes are effective and selective, leading to fewer side reactions and less energy consumption. This biocatalytic approach aligns well with the principles of green chemistry, offering a way to perform reactions under milder conditions.
In addition, researchers are exploring alternative solvents, such as ionic liquids and supercritical fluids, which have lower environmental impacts compared to traditional solvents. These alternatives can enhance reaction efficiency while mitigating toxicity and waste problems. Moreover, integrating automation and artificial intelligence into catalyst design and testing presents another layer of improvement, expediting the discovery of effective sustainable processes.
"The future of catalysis is contingent on our ability to innovate while being mindful of our impact on the planet. Moving towards greener materials and processes is not just a choice but a necessary evolution."
In summary, the future of catalysis research is promising, anchored in developments that prioritize efficiency and sustainability. Emerging materials are paving the way for new catalytic applications, while sustainable strategies ensure that these innovations align with environmental goals. Engaging in this research not only enhances the scientific community but also holds the potential for significant societal impacts.
End
The discussion on catalysts unveils their indispensable role in both industrial and biological contexts, highlighting crucial insights relevant to chemists, researchers, and professionals. This article synthesizes knowledge about catalysts, dissecting how they operate, their types, and the conditions that affect their performance. The importance of catalysts cannot be overstated—they are akin to the unsung heroes of chemical reactions. They facilitate processes that would otherwise be prohibitively slow or impossible. Understanding catalysts is fundamental for innovation in multiple fields.
Summary of Key Points
In sum, this article has delved into several pivotal aspects of catalysis:
- Types of Catalysts: From homogeneous to heterogeneous and enzymatic, each type exhibits unique behavior and applications in chemical settings.
- Mechanism of Catalytic Action: The discussion around energy barriers and transition states illustrates how catalysts lower activation energy, enhancing reaction rates.
- Catalytic Activity Influencers: Temperature, pressure, and concentration are critical parameters that either boost or hinder catalytic processes.
- Lifespan and Deactivation: Awareness of how catalysts degrade and methods for regeneration can prolong the efficiency of catalytic systems.
- Modern Applications: Catalysts play crucial roles in petrochemical processes and environmental chemistry, influencing industry practices.
- Future Outlook: Emerging materials and sustainable strategies signal progress in catalytic research, paving the way for greener chemistry.
"Catalysts are the backbone of efficient chemical processes; without them, we would struggle in achieving desired outcomes in a timely manner."
Implications for Future Research
The exploration of catalysts is far from over. Several areas beckon for deeper investigation:
- Novel Materials: Research into nanomaterials and other advanced substances could revolutionize catalysis by improving efficiency and selectivity.
- Sustainability Concerns: Developing eco-friendly catalysts that require less energy and produce fewer by-products is crucial. This involves exploring lower-impact synthetic routes and waste-minimizing processes.
- Biocatalysts: The future likely holds significant promise for enzymes and other biological catalysts in pharmaceuticals and green chemistry, warranting further studies into their potential.