Advancements and Applications of Small Molecule Fluorescent Probes
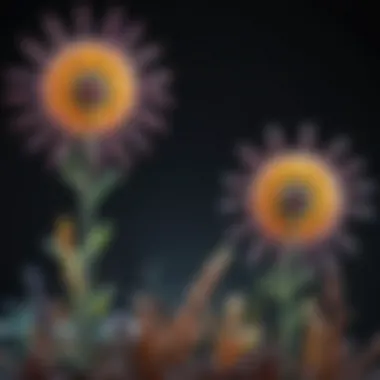
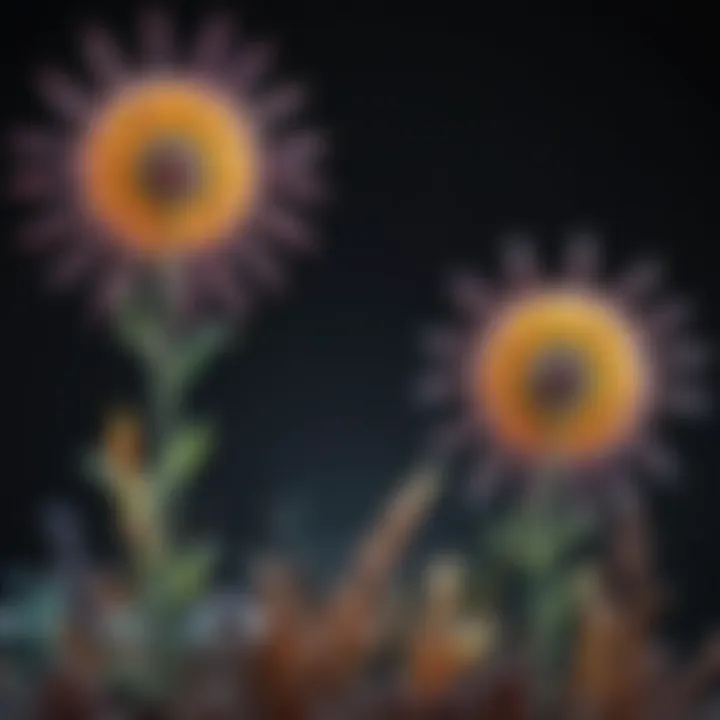
Intro
The realm of small molecule fluorescent probes has burgeoned into a keystone for numerous scientific inquiries, particularly as researchers strive to unravel complex biological processes. These probes, with their unique ability to emit light upon excitation, serve as indispensable tools in various applications, from cellular imaging to biosensing. Their dazzling capabilities are not just a matter of aesthetic allure; they delve deep into realms of molecular biology and chemistry, where understanding interaction and dynamics at a cellular level becomes pivotal.
At the forefront of recent discoveries, these probes have attributed to the remarkable strides in diagnostics, enhancing both specificity and sensitivity. It’s not mere serendipity; these advancements stem from an intricate understanding of molecular interactions and a dedication to newly formulated designs. As we navigate through the layers of this topic, it becomes evident that the exploration of these small molecule fluorescent probes is not only a scientific inquiry but also a profound journey into enhancing our understanding of life itself.
Research Highlights
Overview of Key Findings
The intricate world of small molecule fluorescent probes presents a wealth of findings that can reshape our approach towards bioimaging and diagnostics. Recent literature indicates that the synthesis of novel probes has significantly improved imaging resolution and signal-to-noise ratios. For example, probes engineered to target specific cellular markers have drastically enhanced localization accuracy, allowing for a clearer understanding of cellular behavior.
- Enhanced specificity: New designs target biomarkers with precision a
- Increased sensitivity: Probes can now detect lower concentrations than ever before a
- Versatile applications: Ranging from cancer diagnostics to environmental monitoring
These innovations do not only improve the operational efficacy of probes but also expand their relevance across various disciplines.
Significance of the Research
Understanding the utility of these probes transcends just their application in labs or hospitals; it influences fields as diverse as environmental science and drug development. By manipulating the features of these chemical entities, researchers can bring new light—quite literally—to intricate processes such as cellular signaling mechanisms or pathogen detection.
"The capability of fluorescent probes to illuminate molecular phenomena in real-time fundamentally alters how we observe and interpret biological systems."
The significance of this research stretches far beyond individual projects. It encourages a more integrative perspective in science, potentially leading to breakthroughs in therapies and diagnostic tools that were previously stymied by limitations in detection methods.
Original Research Articles
Summary of the Article
Recent findings in small molecule fluorescent probes exhibit extensive promise for various applications, showcasing the confluence of chemistry and biology. New articles emphasize advances in the tuning of fluorescence properties, enabling targeted actions at molecular levels. This nuanced understanding of probe behavior is paving the way for dynamic applications in live-cell studies and beyond.
Author Contributions
The contributions from multiple authors across journals display a collective effort to enhance probe functionalities. Researchers focusing on chemical synthesis have collaborated with biologists to ensure that the designed probes serve practical and comprehensive purposes. Such collaboration underscores the significance of interdisciplinary approaches needed to propel scientific advancements.
Foreword to Small Molecule Fluorescent Probes
The realm of small molecule fluorescent probes is an essential part of modern chemistry and biology, providing remarkable tools for scientists seeking to understand complex biological systems better. The significance of these probes lies not just in their ability to illuminate cellular and molecular processes, but also in their unique properties that make them versatile in various applications. Through the integration of fluorescence, researchers can study diverse biological pathways, develop diagnostics, and even explore new therapeutic approaches.
Definition and Significance
At its core, a small molecule fluorescent probe is a chemical compound that emits light upon excitation, typically in the visible spectrum. These compounds have a relatively small size, which enables them to interact more readily with cellular structures and biomolecules. By binding to specific targets within a biological system, these probes can provide insights into cellular dynamics, metabolic processes, and disease states. Their significance is highlighted by several factors:
- Versatility: Fluorescent probes can be tailored to bind specifically to various biomolecules, including proteins, nucleic acids, and ions.
- Real-Time Observation: They allow scientists to observe processes in real time, offering dynamic insights that are often missed through conventional methods.
- High Sensitivity: The ability to detect subtle changes in fluorescence makes these probes particularly useful for studying low-abundance biomolecules.
Overall, small molecule fluorescent probes are invaluable for advancing biological sciences, making them a focal point of recent research and development efforts.
Historical Development
The journey of fluorescent probes began long before their current applications were realized. Research into fluorescent compounds dates back to the latter half of the 19th century. In those early days, scientists mainly focused on natural fluorescent compounds or simple synthetic dyes, which were primarily used in microscopy. As the field of biochemistry evolved, interest shifted towards developing dyes that could specifically target biological structures.
The development of the first fluorescent probes for cellular imaging gained traction in the 20th century, when researchers created probes capable of staining cells and tissues, allowing for detailed visualization under ultraviolet light. By the early 2000s, advancements in molecular biology and synthetic chemistry spurred the creation of new families of fluorescent molecules, including the renowned cyanine dyes and a variety of organic compounds.
In recent years, strides in nanotechnology and molecular engineering have revolutionized the design and application of fluorescent probes. These advancements have enhanced their specificity and sensitivity, leading to groundbreaking discoveries in medical research and diagnostics. Every decade has seen a continuous refinement in probe design, adapting to new challenges and unlocking novel applications in disease monitoring and environmental analysis.
In summary, the historical development of small molecule fluorescent probes illustrates a fascinating evolution that has paved the way for their current utility in exploring the complexities of life at the molecular level.
Fundamental Properties of Fluorescent Probes
The fundamental properties of fluorescent probes are central to their effectiveness in a myriad of applications. Understanding these properties allows scientists to better harness the potential of these small molecules in biological and environmental research. Specifically, the exploration of their fundamental characteristics provides critical insights into how these probes can be designed and utilized to optimize their performance.
Fluorescence Mechanism
At the heart of every fluorescent probe lies the fluorescence mechanism, a process responsible for the emission of light when a substance absorbs photons. This phenomenon begins with the excitation of an electron to a higher energy state. When the electron returns to its ground state, it releases energy in the form of a photon, resulting in visible light emission. This transformation can be broadly categorized into three key stages:
- Excitation: The probe absorbs energy from an external light source. This energy is typically within the ultraviolet or visible spectrum.
- Energy State Transition: While in the excited state, the electron may undergo non-radiative relaxation processes before returning to a lower energy level.
- Emission: Upon returning to the ground state, the probe emits light of a longer wavelength, which is visible as fluorescence.
This intricate ballet of energy exchange is crucial. As a result of the varying efficiencies of the excitation and emission processes, different probes exhibit distinctive fluorescence properties. Understanding these can lead to the design of probes that can be tailored for specific applications.
Additionally, factors such as solvent polarity and pH can significantly influence fluorescence quantum yields, thus requiring careful consideration in experimental design.
"The fluorescence of a probe is as much a function of its environment as it is of its inherent properties."
Chemical Structure and Characteristics

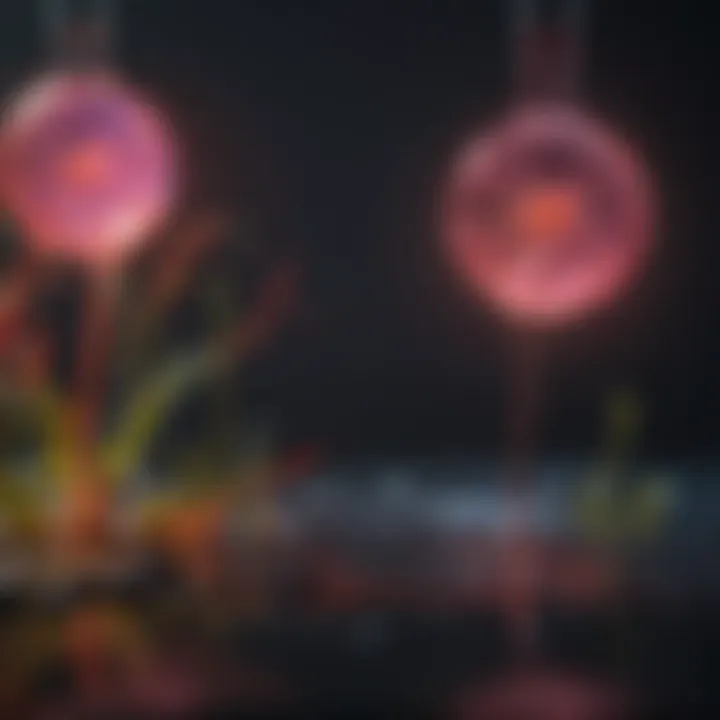
The chemical structure of a fluorescent probe plays a vital role in determining its characteristics and functionality. Small molecule probes are often organic compounds, and their structures typically consist of fluorophores—molecular entities that emit fluorescence. The types of chemical moieties present in a probe can greatly impact its solubility, stability, and reactivity. Here are several important aspects:
- Functional Groups: The presence of specific groups like amines, carboxyls, and hydroxyls can considerably affect the photophysical properties of the probes.
- Stability: A stable chemical structure is essential for consistent performance. Probes with reactive moieties can degrade under certain conditions, risking the integrity of the signals they provide during applications.
- Lipophilicity vs Hydrophilicity: Probes must balance these traits to ensure they can navigate cellular environments effectively. Highly lipophilic probes may struggle to dissolve in aqueous environments, while those that are overly hydrophilic may not penetrate cell membranes effectively.
The Role of Small Molecule Probes in Biological Research
Small molecule fluorescent probes play a crucial part in elucidating biological processes, offering scientists an innovative means to explore cell functioning. The effectiveness and versatility of these probes stem from their ability to provide real-time insights into various cellular phenomena. There are multiple layers of importance when it comes to their utility in biological research, including cellular imaging, tracking metabolic processes, and their contributions to diagnostics and therapeutics.
One of the key benefits is their capacity to penetrate cellular membranes with relative ease. This feature allows for precise observation of intracellular dynamics that had been hard to access previously. In essence, small molecule probes can visualize the unseen, opening doors to countless research avenues.
Cellular Imaging Techniques
Cellular imaging techniques have undergone considerable transformation due to the advent of small molecule fluorescent probes. These probes are utilized extensively in live-cell imaging, offering insights into cellular morphology, structure, and function. Traditional imaging methods, while informative, often fell short of providing real-time data in a living context.
Utilizing advanced fluorescence microscopy techniques such as confocal and two-photon microscopy, researchers can achieve impressive spatial resolutions. A specific example includes probe-based imaging of cancer cells, where fluorescent markers can tag proteins involved in tumor progression, thus laying bare the mechanisms that allow tumors to thrive and even evade detection by the immune system.
- High-throughput capabilities: Recent advancements in multiplexing allow multiple cellular targets to be assessed simultaneously. This approach gives a broader perspective on intercellular interactions and behaviors.
- Live-cell tracking: Small molecules capable of emitting fluorescence under specific conditions empower scientists to track cellular movements over time, enabling deeper insight into processes like migration and division.
Overall, cellular imaging techniques have been greatly enriched by the inclusion of small molecule probes, exemplifying their significance in revealing complex biological behaviors.
Tracking Metabolic Processes
The ability to trace metabolic pathways is another fundamental role that small molecule probes play in biological research. They can selectively bind to specific metabolites or enzymes, presenting a clear picture of how these substances are utilized within the body or in cultured cells.
For instance, probes designed to react with reactive oxygen species can be used to monitor oxidative stress levels—an essential factor in various diseases including neurodegeneration and cancer. When these probes are introduced into living systems, they undergo a chemical reaction that produces fluorescence, directly correlating with changes in the metabolic environment.
The adaptability of small molecule fluorescent probes is evident in their application across different biological contexts. They can be tailored to detect specific molecules, enhancing our understanding of metabolic processes in health and disease.
In addition to direct detection, these probes can yield quantitative data on enzyme activities. By measuring fluorescence intensity, researchers can effectively gauge enzyme kinetics in real-time, which is transformative for understanding biochemical reactions at a molecular level.
In summarization, small molecule fluorescent probes not only contribute significantly to cellular imaging but also play a vital role in tracking metabolic processes. Their unique properties and advanced functionalities have established them as indispensable tools in the realm of biological research. By continuing to innovate and optimize these probes, the scientific community stands to gain even deeper insights into the intricate workings of life itself.
Applications in Biosensing
Biosensing is a field with a significant impact on medical diagnostics, environmental monitoring, and food safety. Small molecule fluorescent probes serve as essential tools for this purpose. They allow researchers and professionals to detect and measure various chemical and biological substances efficiently. The key benefits of utilizing these probes are their high sensitivity, specificity, and the ability to provide real-time analytical data.
In the context of biosensing, small molecule fluorescent probes can be engineered to respond to specific target biomolecules or environmental factors. This ability leads to enhanced accuracy in diagnostics and more effective monitoring systems, crucial for real-time decision-making. The flexibility in design means these probes can be adapted for a wide range of applications, underscoring their significance in both academic and applied sciences.
Detection of Biomolecules
The detection of biomolecules is pivotal in understanding physiological processes and disease mechanisms. Small molecule fluorescent probes can selectively bind to target biomolecules, such as proteins, nucleic acids, or metabolites. This binding often results in a measurable fluorescence change, which can be quantitatively assessed.
Benefits of Detecting Biomolecules
- High Sensitivity: These probes can detect even trace amounts of biomolecules, which is critical in early disease diagnosis.
- Enhancement of Specificity: Tailoring the probes to target specific biomolecules reduces the chances of cross-reactivity, leading to clearer results.
- Real-Time Monitoring: The dynamic nature of fluorescence makes it suitable for monitoring biological processes in live cells without significant interference.
"Fluorescent probes are fundamentally altering the landscape of biomolecular detection, enabling scientists to unveil hidden cellular activities in unprecedented detail."
Environmental Monitoring
Environmental monitoring is another area where small molecule fluorescent probes have made their mark. They can be utilized to detect pollutants, toxins, or various environmental conditions, contributing to a safer ecosystem. These probes can provide information about water quality, soil contaminants, and air pollution by measuring specific markers related to these factors.
Considerations for Environmental Monitoring
- Sensitive Detection of Contaminants: Small molecule fluorescent probes can identify harmful substances at low concentrations, which traditional methods may overlook.
- Versatility Across Different Mediums: Whether in water, soil, or air, these probes can be adapted for various samples to ensure comprehensive environmental assessments.
- Integration with Other Technologies: Combining fluorescent probes with mobile or portable devices can provide on-site testing solutions, significantly enhancing response times in environmental crises.
In summary, the applications of small molecule fluorescent probes in biosensing illustrate their vital role in advancing detection technologies across multiple disciplines. Their capacity for both biomolecule detection and environmental monitoring showcases their versatility and relevance in scientific research and real-world solutions.
Advancements in Probe Design
The field of fluorescent probes is witnessing a renaissance in design strategies. Advancements in probe design are crucial for enhancing the capabilities of small molecule fluorescent probes, allowing them to serve various applications in biological and environmental research. As the need for more precise and efficient tools grows, researchers and developers are focused on refining chemical structures to provide heightened specificity and sensitivity. These enhancements not only facilitate better imaging techniques but also improve the quantification of dynamic biological processes, which are often intricate in nature.
Enhancing Specificity
In the context of small molecule fluorescent probes, specificity refers to the probe's ability to selectively interact with its target—be it a specific biomolecule or cell type. Achieving enhanced specificity is pivotal in reducing background noise during experiments and ensuring that the signals obtained are representative of the target analyte. One approach to enhancing specificity is through the designing of probes that incorporate specific functional groups or chemical moieties that are known to have high affinity for certain targets.
For instance, the incorporation of cyclodextrin derivatives has shown promise in recognizing particular biomolecules due to their unique structural features. This advancement has paved the way for more precise fluorescent signals in complex mixtures.
Moreover, the use of click chemistry, an innovative method that allows for highly selective bonding between molecules, is revolutionizing the probe design landscape. By allowing scientists to functionalize probes with customized targeting groups, click chemistry promotes the development of probes that can discriminate between closely related structures.
- Key Benefits of Enhanced Specificity:
- Reduces nonspecific binding, leading to clearer data.
- Allows detection in complex biological samples with minimal interference.
- Facilitates targeted drug delivery and diagnosis.
Improving Sensitivity
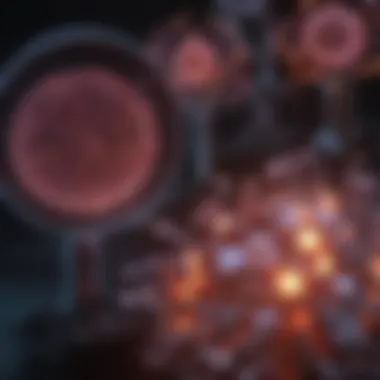
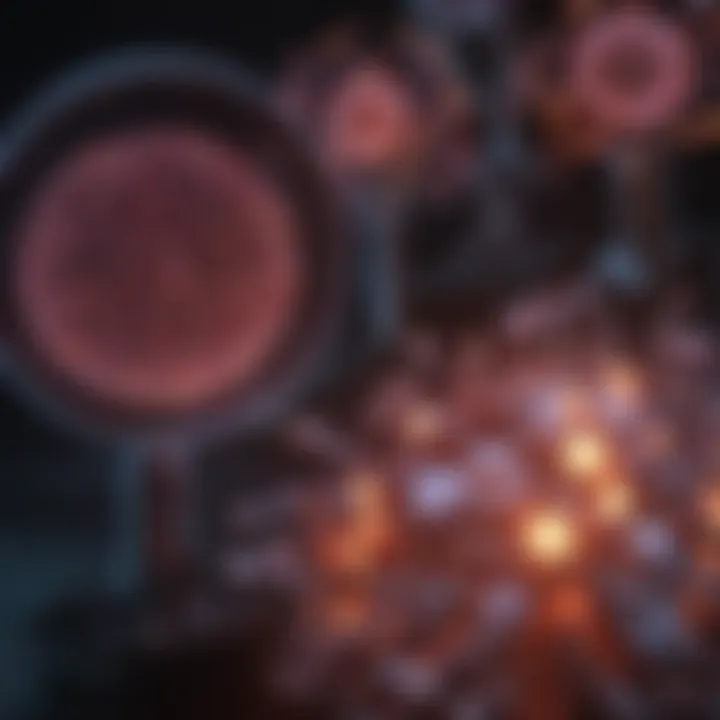
Alongside specificity, sensitivity is another critical facet affecting the effectiveness of small molecule fluorescent probes. Sensitivity refers to the probe's ability to detect low concentrations of target molecules. Improvements in sensitivity can lead to earlier detection of diseases or environmental toxins, making it invaluable for clinical diagnostics and monitoring.
Recent developments in probe design have leveraged the principles of nanotechnology. For example, the integration of quantum dots as labeling agents has enhanced the sensitivity of fluorescent probes significantly. These semiconducting nanoparticles possess unique optical properties that allow for greater light absorption and emission, thus enabling the detection of lower analyte concentrations.
Additionally, the development of hybrid probes combining fluorescent properties with other signal amplification techniques promises to improve sensitivity further. Such strategies can involve enzymatic reactions that enhance the fluorescent response or amplification methods such as rolling-circle amplification.
- Benefits of Improved Sensitivity:
- Enables the detection of biomarkers at earlier disease stages.
- Enhances environmental monitoring efforts by allowing the identification of trace pollutants.
- Provides a higher level of confidence in the quantitative analysis of samples.
Challenges in the Development of Fluorescent Probes
The progress in the use and design of small molecule fluorescent probes is nothing short of remarkable. However, like any scientific field, this arena is not without its hurdles. Understanding the challenges associated with fluorescent probes is pivotal because overcoming these issues can lead to even more significant advancements. This section delves into two critical challenges: stability issues and biocompatibility concerns, both of which can substantially impact the effectiveness of these probes in practical applications.
Stability Issues
Stability is a linchpin aspect for fluorescent probes. These molecules must maintain their fluorescence under various experimental conditions, which often include extreme pH levels, varied temperatures, or different ionic strengths. A probe that fades or degrades too quickly is practically useless. For instance, compounds like Rhodamine B are generally stable, but their fluorescence can diminish quickly in certain solvents or in the presence of specific ions.
In many biological experiments, probes are exposed to cellular environments that can wreak havoc on their stability. Light exposure can also lead to photobleaching, where the probe’s ability to fluoresce diminishes over time due to prolonged light exposure. This phenomenon is particularly troublesome during long-term imaging studies where researchers expect consistent signals.
To tackle stability issues, researchers are exploring modifications in the chemical structure of fluorescent probes. Such alterations can lead to enhanced resistance to photobleaching or degradation, ultimately allowing for prolonged usage in experimental settings.
"Addressing stability not only improves the experimental fidelity but also ensures that our interpretations of biological data are on point."
Biocompatibility Concerns
Another significant challenge is the biocompatibility of fluorescent probes. As these probes are often used in biological systems, including live cells and tissues, they must not interfere with normal cellular functions. This means they need to be nontoxic and should not elicit immune responses.
For example, some synthetic probes might produce side effects that complicate the interpretation of experimental results. Probes designed with conventional chemical structures can sometimes trigger toxic responses or accumulate within specific organelles, skewing the data.
Finding the right balance between effective performance and safety is crucial. Researchers often assess biocompatibility through various methods before initiating in vivo experiments. These assessments typically involve testing probes on cell lines to monitor for cytotoxicity or inflammatory responses.
Closure
In summary, the challenges of stability and biocompatibility underscore the complexities inherent in the field of fluorescent probe development. Addressing these issues isn't merely a scientific concern—it's foundational to enhancing the utility and precision of fluorescent probes in research applications. By prioritizing stability and biocompatibility, scientists can unlock new potentials for these versatile tools, paving the way for revolutionary procedures in both laboratory and clinical settings.
Emerging Trends in Small Molecule Probes
Emerging trends in small molecule fluorescent probes represent a significant leap in the capability of these tools, pushing the boundaries of what we previously believed possible in both research and practical applications. At the core of this evolution is a desire for increased precision and versatility in monitoring biological processes, allowing researchers to gather more detailed data without resorting to invasive methods. As these probes evolve, they provide state-of-the-art solutions in a variety of fields, including therapeutic monitoring and environmental assessments.
Applications in Therapeutic Monitoring
One of the most notable applications of small molecule fluorescent probes lies in therapeutic monitoring. This involves tracking the effectiveness of treatments in real-time without disrupting ongoing biological processes. Such probes can indicate biomarkers that signal how well a drug is working or when to adjust dosages. For instance, treating chronic diseases often requires close evaluation of a patient’s response to medications. Fluorescent probes that are sensitive to specific molecular markers allow for non-invasive observation, thereby enabling timely adjustments in therapeutic interventions.
- Precision: Probes can detect minute changes in biomarker levels, giving an accurate picture of the treatment's impact.
- Real-time feedback: Ongoing monitoring allows healthcare professionals to make immediate decisions, which could be critical in acute care scenarios.
- Reduced risk: By using fluorescent probes instead of traditional biopsy methods, patient comfort is notably increased, reducing both pain and anxiety.
With advances in this area, it's become standard for researchers to strive for higher sensitivity and specificity. Thus, developing probes that can selectively detect single molecules without interference from background noise becomes paramount.
Integration with Nanotechnology
Nanotechnology has emerged as a powerful ally in enhancing the functionality of small molecule fluorescent probes. Combining the unique properties of nanomaterials with fluorescent probes can dramatically increase their sensitivity and specificity. One of the clear advantages is that nanoparticles can provide enhanced contrast in imaging techniques, making it possible to visualize even the tiniest biological structures in their natural environment.
Some key points regarding this integration include:
- Energy Transfer: Nanoparticles can facilitate energy transfer between fluorescent molecules, boosting signal intensity significantly.
- Targeted Delivery: Nanotechnology allows for the design of probes that can deliver fluorescent tags to specific cells or tissues, improving the specificity of imaging.
- Stability Improvements: Encapsulation of fluorescent probes within nanostructures can help improve their stability in biological systems, extending their lifespan during imaging procedures.
As the integration continues, we may see more multifunctional probes that not only fluoresce but also carry therapeutic agents, advancing cancer treatment or other therapies. This ambition paints an exciting future for the convergence of these scientific domains, constantly pushing the boundaries of discovery and application.
"With the rise of nanotechnology and advanced materials, the potential of fluorescent probes is expanding at an unprecedented pace."
Overall, understanding these current trends opens up avenues for more innovative and effective probe designs, which could revolutionize the way researchers and healthcare professionals monitor therapeutic responses.
In summary, emerging trends in small molecule probes signify a crucial part of scientific advancement. They not only redefine our approach to monitoring biological changes but also promise to usher in a new era of accuracy and efficiency in various applications, particularly in therapeutic and diagnostic fields.
Case Studies of Successful Applications
In the realm of scientific research, case studies are like the footprints left behind in the sands of discovery. They tell stories of triumph and challenges faced, offering insights that can guide future research endeavors. This section highlights the application of small molecule fluorescent probes in two critical areas: cancer research and pathogen detection. Understanding these real-world applications underscores not just the utility, but the profound impact these probes have in advancing science.
Fluorescent Probes in Cancer Research
The battle against cancer has seen remarkable developments, particularly with the introduction of small molecule fluorescent probes. These tools have transformed how researchers visualize and understand cancer at the cellular level.
One significant example is the use of probes like Cytotox™ Blue. This probe enables scientists to selectively label and track cancer cells, bringing clarity to their behaviors within living tissues. The ability to observe how these cells respond to different treatments in real-time can lead to a better understanding of tumor dynamics. Researchers discovered that certain small molecule probes could not only highlight cancerous cells but also reveal their metabolic activities, shedding light on how these cells evade destruction.
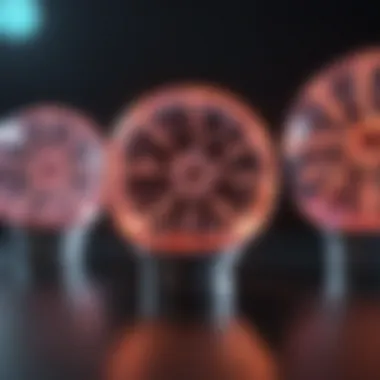
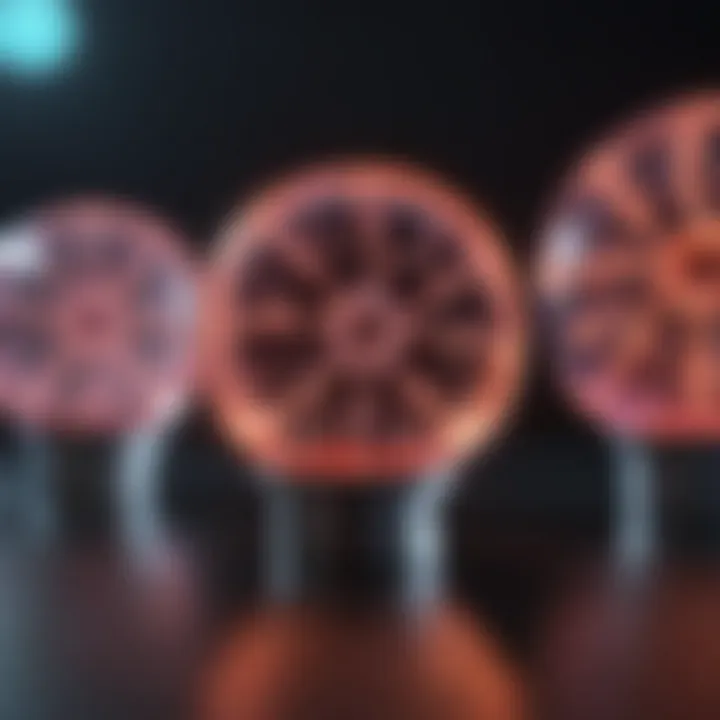
The ability to differentiate between cancerous and healthy cells allows for more precise targeting in therapies, a strategy known as "theranostics." This approach is becoming increasingly popular, combining diagnosis and therapy into a single method. Furthermore, the imaging capabilities provided by fluorescent probes facilitate the early detection of metastases—when cancer spreads to other parts of the body—enabling interventions that can significantly affect patient outcomes.
Probes for Pathogen Detection
In our increasingly interconnected world, the threat posed by pathogens is ever-present. Small molecule fluorescent probes have become vital tools for detecting and identifying infectious agents with accuracy and speed. An illustrative case is the use of FluoProbes™ for the rapid identification of bacterial pathogens in clinical samples. This method not only saves time but also ensures that appropriate treatment can be administered without delay.
In the context of infectious diseases, such as COVID-19, probes have been adapted to identify viral components. Utilizing fluorescent assays, researchers can now quickly ascertain the presence of viral RNA, allowing for expedited testing processes critical in outbreak situations. For instance, studies reported that specific fluorescent probes targeting SARS-CoV-2 exhibited a high degree of sensitivity, making them invaluable in monitoring infection rates.
Moreover, these probes can be designed to signal the presence of pathogens through changes in fluorescence intensity or color. This characteristic makes them accessible for use in various environments, including remote or resource-limited settings, which might lack sophisticated lab equipment. The adaptability and convenience of these probes hold significant promise in enhancing global health.
"Small molecule fluorescent probes are not just tools; they are gateways to understanding complex biological systems and improving health outcomes."
In summary, the applications of fluorescent probes in cancer research and pathogen detection exemplify their transformative potential. They provide researchers with innovative ways to explore biological questions and create actionable insights, paving the path for future advancements. As technology continues to evolve, we can expect these probes to play an even more significant role in health and disease research.
Regulatory and Ethical Considerations
In the realm of scientific research, particularly in areas leveraging small molecule fluorescent probes, regulatory and ethical considerations are paramount. Developing new probes not only involves scientific insights but also requires adherence to specific guidelines that ensure safety and integrity in research practices. This section seeks to unpack the significance of these considerations, focusing on safety assessments and the ethical use of fluorescent probes in various disciplines.
Safety Assessments
When small molecule fluorescent probes are designed for use, thorough safety assessments cannot be overlooked. This process serves multiple purposes:
- Risk Identification: It aids in identifying potential hazards related to the compounds, including toxicity levels or environmental impacts. Each fluorescent probe has its unique trajectory of interaction with biological systems, making it crucial to assess those interactions carefully.
- Regulatory Compliance: Scientists must adhere to legal standards set by organizations such as the Environmental Protection Agency (EPA) and FDA. These bodies often set strict laws to ensure that substances entering the market are adequately tested and proven to be safe.
- Public Trust: Transparency in safety protocols assures the public that researchers prioritize human health and the environment. Without this, public skepticism towards scientific advances could hinder funding and collaboration opportunities.
Through proper safety assessments, researchers ensure that their probes meet stringent requirements and also align with ethical research practices. This trust can foster wider acceptance and utilization of these probes across various fields.
Ethical Use in Research
The ethical use of small molecule fluorescent probes encompasses a range of factors that researchers must navigate. Key points include:
- Informed Consent: When probes are employed in clinical settings, patient consent is imperative. Patients must understand potential risks and benefits before participating in any studies involving probes.
- Transparency in Findings: Researchers have an ethical obligation to report findings honestly, irrespective of the outcomes. Whether results are favorable or disappointing, the integrity of the research community relies on this transparency.
- Animal Welfare: Many studies necessitate the use of animal models to gauge the effectiveness of fluorescent probes before human application. Researchers must ensure that animal trials are designed to minimize suffering and distress, adhering to the principles of 3Rs: replacement, reduction, and refinement.
"Ethical research is not just about strict guidelines but also about respecting the individuals and the environment that we work with."
In summary, navigating the regulatory landscape and ethical implications surrounding small molecule fluorescent probes is essential for fostering scientific innovation while ensuring moral responsibility. By embedding these considerations deep within research protocols, scientists can better secure their place in advancing knowledge responsibly.
Future Directions in Fluorescent Probe Research
As we look ahead, the future directions in fluorescent probe research promise to be both exciting and crucial for various scientific domains. This section aims to extrapolate on upcoming trends, innovations, and potential integrations that could enhance our understanding and application of small molecule fluorescent probes. Knowing the trajectory of this field helps not only in academic inquiry but in practical applications, such as diagnostics and therapeutics. The research community is focusing on two key avenues that could significantly alter the landscape: novel chemical approaches and multidisciplinary collaborations.
Novel Chemical Approaches
Advancing fluorescent probes requires diving into new chemical methodologies. Researchers are tirelessly exploring unique synthetic pathways to produce probes with enhanced functionalities. For instance, incorporating novel scaffolds can lead to brighter signals and improved stability, making these probes more effective in real-time imaging.
The integration of click chemistry has emerged as a particularly useful innovation. This approach facilitates straightforward conjugation of probes to target molecules, potentially increasing specificity and minimizing background noise. The adaptability of these new chemical techniques has piqued the curiosity of scientists worldwide, eager to test their validity in various biological contexts.
Additionally, modified fluorophores with tailored photophysical properties are under intense scrutiny. The aim is to create probes that can seamlessly switch between fluorescent states depending on their microenvironment, offering real-time insights into dynamic biological processes. In this quest, the designs push boundaries, utilizing both known and unexplored chemical properties.
"Innovative chemical methods are paving the way for probes that are not only smarter but also more reliable in practical applications."
Collaboration Between Disciplines
The intermingling of disciplines stands as an essential pillar for the advancement of fluorescent probe research. Traditionally rooted in chemistry and biology, this field can benefit significantly from interdisciplinary approaches that incorporate principles from physics, engineering, and even computer science.
For example, engineers can help refine the techniques of probe delivery, ensuring that the probes reach their ecological niches with higher precision. Meanwhile, physicists can contribute by enhancing the spatial and temporal resolution of imaging technologies, leading to clearer, more informative data. Moreover, computer scientists can utilize machine learning algorithms to analyze vast datasets generated by these probing techniques, identifying patterns and anomalies at speeds unattainable by humans alone.
This collaboration also encourages diversity of thought, which stimulates innovative problem-solving. Academic institutions and industry leaders are increasingly recognizing that knowledge transfer among fields can yield groundbreaking advancements. Connecting specialists from various areas encourages a culture of shared ideas, maximizing the potential to solve complex scientific challenges.
Thus, engaging in cooperative efforts—whether through formal partnerships or community discussions within forums—could cement the future of small molecule fluorescent probes as an indispensable tool in scientific research.
In summary, both novel chemical methodologies and interdisciplinary collaboration are cornerstones for the evolution of fluorescent probes. Keeping an eye on these developments will not only shed light on new potentials but also empower researchers to tackle obstacles previously thought insurmountable.
Culmination
The role of small molecule fluorescent probes in scientific research cannot be overstated. These compounds are not merely useful tools; they are the backbone of many advances in cellular imaging, biosensing, and diagnostics. As highlighted throughout this article, their fundamental properties enable researchers to visualize and measure biological processes with great precision.
Summary of Key Insights
Summarizing the key insights gained from this exploration:
- Versatility: These probes are applicable across diverse domains, from cancer research to environmental monitoring.
- Advancements: Recent improvements in specificity and sensitivity are paving the way for more accurate diagnostics and vibrant imaging.
- Challenges: Despite their potential, issues such as stability and biocompatibility remain pressing concerns that require further investigation.
The integration of these probes into research practices offers significant benefits, enhancing our understanding and capabilities in scientific exploration. Their ongoing development signals a promising horizon for the life sciences and beyond.
Implications for Future Research
Looking ahead, the future research involving small molecule fluorescent probes stands to bring forth numerous possibilities.
- Increased Collaboration: Scientific inquiries can greatly benefit from collaboration across disciplines, marrying biology with engineering and materials science to optimize probe effectiveness.
- Innovative Designs: The ongoing evolution of chemical approaches promises to yield probes with enhanced performance metrics.
- Sustainable Practices: As attention to environmental impacts grows, probes that incorporate green chemistry principles may become crucial.
Emerging technologies, such as machine learning, could also be harnessed to refine probe design, thereby increasing their utility in various scientific fields. The journey of small molecule fluorescent probes is far from over, and their future holds rich potential for groundbreaking discoveries.