Thermal PCR: Concepts, Uses, and Future Trends
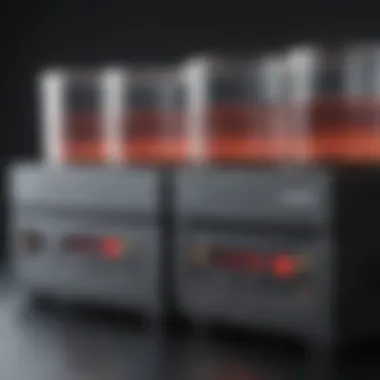
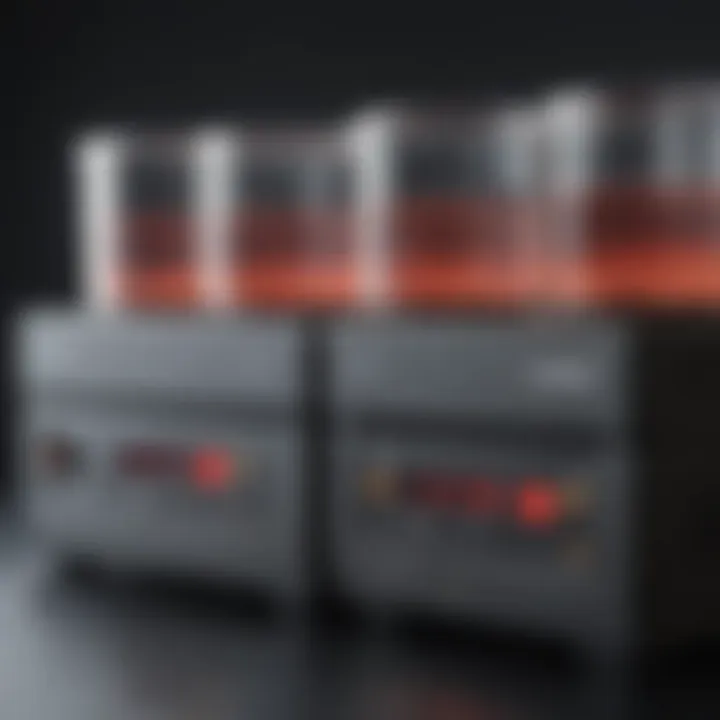
Intro
Thermal Polymerase Chain Reaction, commonly referred to as thermal PCR, stands as a foundational pillar in the realm of molecular biology. This powerful technique has revolutionized our ability to amplify DNA exponentially, making it indispensable in various fields ranging from genetic research to forensic science. At its core, thermal PCR operates through a series of temperature cycles that facilitate the denaturation, annealing, and extension of DNA. As the process unfolds, its versatile applications have broadened, making it a crucial tool in diagnostics and research on a global scale.
Understanding thermal PCR is not only crucial for specialists in laboratories but also for students and educators who aim to grasp contemporary biological methodologies. With ongoing advancements in this technology, the implications it holds for the future of science are profound and far-reaching. In this article, we will delve into the underlying principles of thermal PCR, its diverse applications, and the exciting future directions shaping its evolution.
Research Highlights
Overview of Key Findings
As we navigate through the intricacies of thermal PCR, several key findings emerge that underline its significance in scientific inquiry and practical applications:
- DNA Amplification: Thermal PCR can amplify trace amounts of DNA, allowing researchers to utilize limited samples effectively.
- Precision: Advances in polymerase enzymes have resulted in higher fidelity in amplifying DNA, reducing errors and enhancing the accuracy of results.
- Versatility: The flexibility of thermal PCR extends beyond typical applications, finding a place in areas like ancient DNA extraction and environmental monitoring.
In addition to these highlights, ongoing innovations in thermal PCR methodologies, such as digital PCR and multiplex PCR, promise to elevate the capability of this technique even further.
Significance of the Research
The significance of thermal PCR cannot be overstated. It has not only facilitated breakthroughs in various research domains but also made strides in practical applications:
- Genetic Research: Thermal PCR has opened new doors for studies in genetic variations, providing crucial insights into genetic diseases and heredity patterns.
- Medical Diagnostics: Outbreaks of infectious diseases have seen thermal PCR play a vital role in rapid diagnostics, leading to timely interventions and treatments.
- Forensics: The ability to amplify small quantities of DNA found at crime scenes is paramount, helping law enforcement agencies solve cases through DNA profiling.
"The power of thermal PCR lies not just in amplifying DNA, but in amplifying our potential to understand life at its most fundamental level."
Practically, as researchers continuously push the envelope of what is possible, the implications for society—through enhanced healthcare, improved forensic capabilities, and a deeper understanding of genetics—become increasingly apparent.
Original Research Articles
Summary of the Article
This article serves as a compendium of crucial information surrounding thermal PCR, woven together with a focus on its principles and implications in various fields. The narrative flows through the technical aspects and applications, ensuring a comprehensive understanding of the topic while remaining accessible to those new to the field.
Author Contributions
The article synthesizes insights from numerous experts in molecular biology. It encapsulates both foundational knowledge and the latest frontiers in thermal PCR research, reflecting a collective effort to illuminate this essential technology for a diverse audience.
As the article unfolds, expect to unpack the complexities of thermal PCR in a manner that is insightful and engaging, making grasping these principles both enlightening and accessible.
Preamble to Thermal PCR
In the realm of molecular biology, the significance of thermal PCR resonates profoundly across various disciplines. This technology serves as a vital process for amplifying DNA, rendering it not only indispensable for laboratory practices but also a fundamental tool in genetic research, diagnostics, and forensic science. This introduction sheds light on the core principles behind thermal PCR, emphasizing its multifaceted application.
The Genesis of PCR Technology
Polymerase Chain Reaction, or PCR, originated from a drive for efficiency and precision in DNA analysis. Developed in 1983 by Kary Mullis, this innovative method sought to alleviate the challenges scientists faced in studying small quantities of DNA. At that time, genetic analysis was often limited, requiring extensive processing and subjecting samples to degradation.
Mullis, armed with the vision to simplify his work, stumbled upon the idea of exploiting the natural mechanism of DNA replication through a heat-stable enzyme known as Taq polymerase. This enzyme, derived from the thermophilic bacterium Thermus aquaticus, allowed for the high-temperature processes necessary for PCR without denaturing itself. By cycling through various temperatures, this technique could selectively amplify specific DNA sequences—majorly altering the landscape of molecular biology and countless fields relying on genetic analysis.
Importance of PCR in Molecular Biology
The implications of PCR in molecular biology are staggering. PCR has enabled scientists to dissect the intricacies of the genetic code, offering insights into hereditary diseases, evolutionary biology, and genetic diversity. Here are some reasons it stands out:
- Precision: PCR allows for accurate amplification of target DNA sequences, minimizing errors inherent in other methods.
- Speed: The ability to produce millions of copies in a matter of hours expedites research and diagnostic processes.
- Versatility: PCR can be applied to various samples, from blood to soil, making it adaptable to different scientific inquiries.
"PCR bridged a significant gap in molecular biology, thereby fostering innovations in diagnostics and therapeutics."
In addition to these benefits, thermal PCR cultivates advancements in various fields. From clinical settings that require timely detection of viruses to environmental monitoring where detecting microbial presence in soil samples is critical, PCR's role cannot be overstated. The ability to amplify minute DNA fragments has shaped research paradigms, paving the way for diverse applications—each laying a foundation for new scientific inquiries.
Understanding these fundamentals is essential for appreciating the overarching significance of thermal PCR in modern science. The subsequent sections will delve deeper into the principles that govern this technique, exploring its mechanics and varied applications.
Basic Principles of Thermal PCR
The bedrock of thermal PCR lies in its foundational principles, which are critical to understanding how this technology revolutionized molecular biology. Diving into these principles reveals not just the workings but also the importance of various components and the thermal cycling process. Each element plays a significant role in achieving the end goal - amplification of DNA sequences. This process not only enhances molecular biology research but also finds crucial applications across various fields.
Fundamental Components
DNA Template
The DNA template serves as the blueprint in the thermal PCR process. Without it, amplification wouldn’t even be on the table. The key characteristic of a DNA template is its specificity. It essentially dictates which segment of DNA is to be amplified, making selection of the right template pivotal for successful experiments. Moreover, the choice of DNA template influences the efficiency and accuracy of the PCR. Ideally, the template should be in sufficient quantity, as lower concentrations might lead to subpar results. However, a higher concentration can cause issues such as nonspecific amplification.
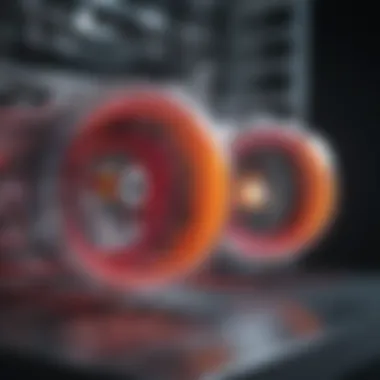
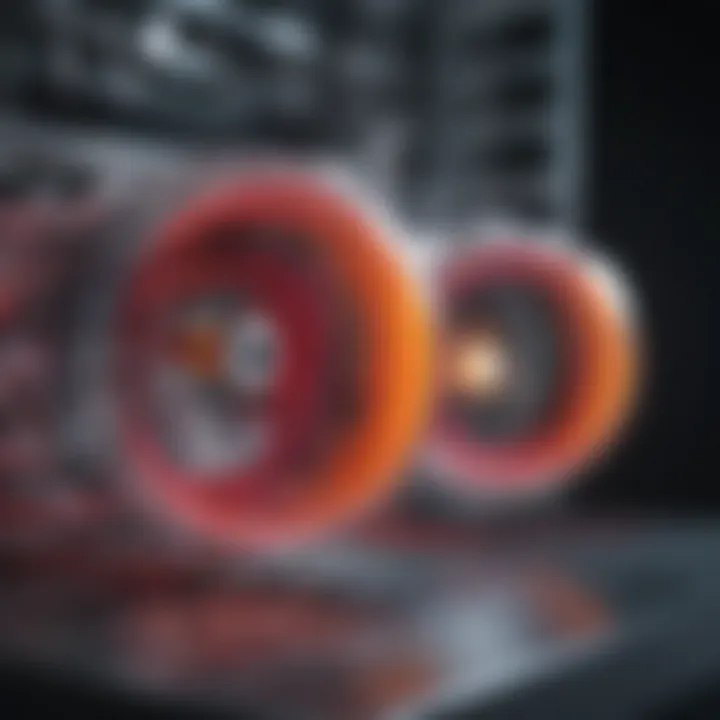
Primers
Primers are short sequences of nucleotides that are essential for the initiation of DNA synthesis. The significance of primers cannot be overstated; they provide the starting point for DNA polymerase to synthesize new strands. A crucial feature of primers is their balance of specificity and versatility. The complementary nature of primers to the target region allows them to anneal precisely where amplification is desired. However, designing the right set of primers can be a pinch. For instance, poor design might cause mismatched binding, resulting in undesirable amplification products, which can confuse results.
DNA Polymerase
DNA polymerase is often deemed the powerhouse of the PCR. This enzyme performs the actual synthesis of new DNA strands by adding nucleotides complementary to the template strand. One popular choice is Taq polymerase, known for its heat resilience, which allows it to thrive even in the high temperatures of PCR. This characteristic is advantageous since it enables the enzyme to survive the denaturation phase during thermal cycling, ensuring effective amplification. However, it’s also crucial to remember that not all polymerases are created equal; their fidelity and efficiency can vary, influencing the quality of the final product.
Buffer Solutions
Buffer solutions are the unsung heroes in the world of thermal PCR. These solutions create an optimal environment for the reaction, ensuring that all components function correctly. The key characteristic of a good buffer is its ability to maintain pH levels while providing essential ions like magnesium, which are critical for enzymatic activity. That said, choosing the right buffer can sometimes feel like walking a tightrope – too much or too little can throw everything off balance, potentially leading to poor amplification.
The Thermal Cycling Process
Denaturation Phase
The denaturation phase is the first step in the thermal cycling process, where the double-stranded DNA unwinds into single strands due to high temperatures, typically around 94-98°C. This phase is crucial because it sets the stage for the entire amplification process, ensuring that the DNA template is accessible for the next stages. A unique feature of this phase is its speed; denaturation happens in mere seconds. However, if the temperature is too high for too long, it might risk damaging the DNA, highlighting the need for precision in temperature control.
Annealing Phase
Subsequently, the reaction transitions to the annealing phase, where the temperature is lowered to allow primers to bind to their complementary sequences on the single-stranded DNA template. Commonly, this temperature ranges from 50-65°C depending on the primer sequences. This is a pivotal moment, as it largely determines the specificity of the PCR process. Proper primer annealing is essential; if the conditions are not optimal, nonspecific binding may occur. Inadequate annealing might lead to inefficient amplification, underscoring the importance of meticulous temperature regulation.
Extension Phase
Following annealing, the extension phase occurs, which generally takes place at around 72°C. During this time, DNA polymerase extends the primers by adding nucleotides, effectively building new strands of DNA. This phase is critical because it actualizes the amplification process. The unique aspect of this phase is its duration; it often varies based on the size of the target DNA fragment. A common rule of thumb is that about one minute for every kilobase of DNA is a good parameter. However, prematurely cut short or extended extensions can affect yield and accuracy, making careful timing imperative.
In summary, the principles behind thermal PCR provide a thorough understanding of this indispensable technology in molecular biology. The collaboration of each component and the precision of the thermal cycling process pave the way for effective DNA amplification, facilitating groundbreaking research and advancements across multiple disciplines.
Different Types of PCR Techniques
Thermal PCR is not a one-size-fits-all technology; rather, it has branched out into various techniques, each with their unique strengths and applications. Understanding the differences among these techniques is crucial for selecting the appropriate one for a specific application. Different types of PCR techniques allow for greater versatility, improved efficiency, and tailored outputs, each bringing something new to the table of molecular biology. This section focuses on four key PCR techniques – Quantitative PCR (qPCR), Reverse Transcription PCR (RT-PCR), Multiplex PCR, and Digital PCR – exploring their significance and individual contributions.
Quantitative PCR (qPCR)
Quantitative PCR, commonly referred to as qPCR, has gained popularity due to its ability to quantify DNA or RNA in real time. Unlike traditional PCR, which results only in the amplification of DNA, qPCR provides quantitative data as it happens. This level of detail is paramount for researchers looking to measure gene expression levels or detect low-abundance targets.
The process involves the use of fluorescent dyes that bind to the amplified product, allowing for measurement after each cycle.
This dynamic feedback system can be beneficial for:
- Gene Expression Analysis: Understanding variations in expression levels under different conditions.
- Pathogen Detection: Identifying the presence of pathogens at incredibly low concentrations, which is indispensable in clinical diagnostics.
- Genetic Variation Studies: Providing insight into polymorphisms and copy number variations.
The precision of qPCR, however, requires careful calibration and design of primers to prevent non-specific binding, which is a crucial consideration during its application.
Reverse Transcription PCR (RT-PCR)
Reverse Transcription PCR is a clever adaptation that allows for the amplification of RNA sequences by converting them into complementary DNA (cDNA). This process is particularly important in studying gene expression because mRNA levels can indicate how actively a gene is being transcribed.
During RT-PCR, an enzyme called reverse transcriptase synthesizes cDNA from the RNA template. Once the cDNA is formed, it can be amplified using standard PCR methods. This technique provides crucial benefits, including:
- Real-time Monitoring of RNA: Enabling researchers to observe fluctuations in RNA levels across various conditions.
- Viral Load Quantification: Frequently used in virology, especially for RNA viruses like HIV and SARS-CoV-2.
- Detection of Splice Variants: Allowing for nuanced understanding of alternative splicing events that occur during mRNA processing.
Nevertheless, the sensitivity of RT-PCR also opens doors to potential contamination, making careful laboratory protocols essential.
Multiplex PCR
Multiplex PCR amplifies multiple DNA sequences simultaneously within the same reaction mix. This approach significantly enhances efficiency by reducing time and resource expenditure while allowing vast amounts of information to be extracted from a single test.
In multiplexing, multiple primer pairs are designed to target different sequences. The benefits of this technique include:
- Time-savings: A single run can provide information on several targets rather than running individual reactions.
- Improved Diagnostic Capabilities: Critical in clinical settings where multiple pathogens may need to be identified at once, such as in infection testing.
- Forensic Applications: Useful in analyzing multiple short tandem repeats (STRs) for identity verification and paternity testing.
Despite these advantages, the design of multiplex assays requires meticulous attention to minimize cross-reactivity among primers and to achieve balanced amplification efficiency.
Digital PCR
Digital PCR is a powerful evolution of traditional PCR methods that offers unparalleled precision in quantifying nucleic acids. By partitioning the sample into thousands of individual reactions, digital PCR allows for the absolute quantification of target DNA without the need for standard curves.
This technique yields numerous advantages:
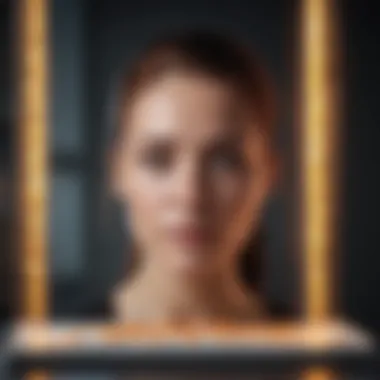
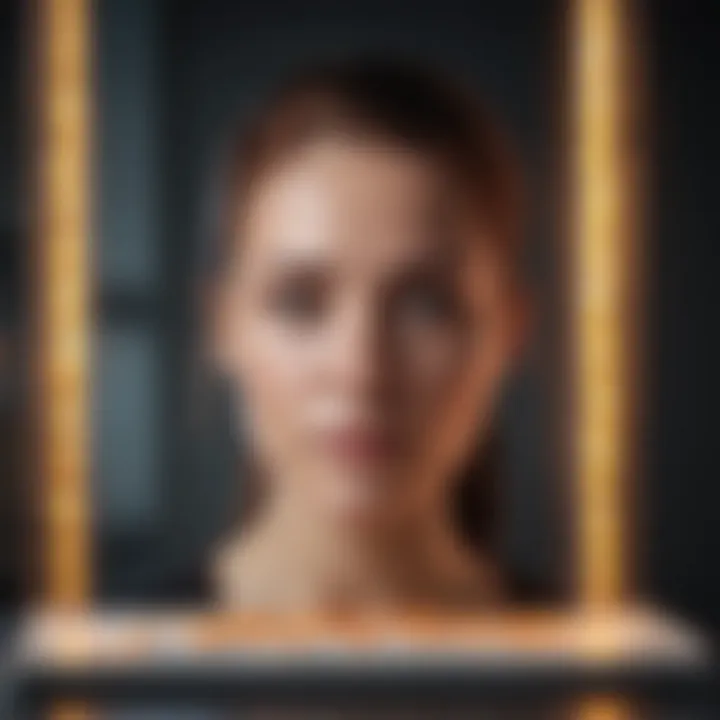
- Robustness against Inhibition: Digital PCR is more tolerant to PCR inhibitors found in complex samples.
- Highly Sensitive Detection: It can detect low-frequency mutations or genetically modified organisms (GMOs) in a sample, making it indispensable for applications like cancer research.
- Improved Reproducibility: Digital quantification is innate to the methodology, ensuring consistency across experiments.
That said, the complexity of setting up the reaction can be a high hurdle for new users; hence, understanding the equipment involved is necessary for successful application.
Applications of Thermal PCR
The significance of Thermal PCR extends far beyond its laboratory confines; it has truly transformed the landscape of scientific inquiry and practical applications alike. From genetic research to forensic science, this technology demonstrates versatility and profound impact across various fields. Understanding the breadth of its applications not only showcases its importance within molecular biology but also illustrates how it influences modern scientific practices and societal concerns.
Genetic Research
In the realm of genetic research, thermal PCR is nothing short of revolutionary. Scientists use it for amplifying fragments of DNA to study genetic disorders, traits, and evolutionary processes. For instance, researchers can analyze gene expressions associated with conditions like cystic fibrosis or Huntington's disease. This application enables a deeper understanding of genetics, which can lead to predictive diagnostics and targeted therapies.
The ability to generate millions of copies from a minute DNA sample opens doors to studying rare genetic variations. It's like finding a needle in a haystack, but with thermal PCR, that needle becomes easier to spot and examine. Furthermore, the rapid development of quantitative PCR (qPCR) enhances this process by allowing the measurement of DNA in real-time.
"Thermal PCR, when applied to genetic research, becomes akin to a magnifying glass, enabling us to inspect the intricate details of life at a molecular level."
Clinical Diagnostics
In clinical settings, the application of thermal PCR is perhaps most critical. It serves as a cornerstone in the diagnosis of infectious diseases, such as HIV, tuberculosis, and coronavirus. Clinicians can detect specific pathogens quickly, often within hours, allowing for timely treatment decisions.
Moreover, PCR is pivotal in genomic medicine, where it helps identify mutations related to various cancers. Tailoring personalized treatment based on specific genetic markers can significantly improve patient outcomes. Its sensitivity and specificity mean that even low amounts of target DNA can be identified, which is crucial for early-stage diagnostics.
Forensic Science
The impact of thermal PCR in forensic science is substantial. By amplifying DNA from crime scene samples, investigators can identify suspects or victims with remarkable precision. It's not uncommon for this DNA amplification to come from just a few cells found at the scene.
Forensic experts utilize PCR to analyze various biological samples, including blood, hair, and even saliva. This application not only aids in solving crimes but has also exonerated numerous individuals wrongly accused. The power of amplifying minuscule DNA samples operates like putting together pieces of a puzzle to create a clearer picture of events that transpired.
Environmental Monitoring
Lastly, thermal PCR has a profound role in environmental monitoring. It helps researchers identify microbial populations in different ecosystems, providing insights into biodiversity, ecological health, and environmental changes. For example, scientists can monitor the presence and spread of diseases in wildlife populations, contributing to conservation efforts.
In agriculture, its application extends to detect plant pathogens that threaten food security. By swiftly identifying contaminants, farmers can take necessary measures to protect their crops. This proactive approach to managing ecological health illustrates how thermal PCR aids in sustaining not just local environments but global food systems.
In summary, the applications of thermal PCR demonstrate its substantial importance in modern science. Whether amplifying DNA for genetic research, diagnosing diseases in clinical practices, identifying human remains in forensic investigations, or monitoring environmental health, this technology stands as a testament to how molecular biology continues to evolve and influence diverse fields.
Innovations in PCR Technology
In the ever-evolving field of molecular biology, the significance of innovations in PCR technology cannot be overstated. As research demands shift and the complexities of biological samples increase, the ability to adapt and refine PCR techniques is essential for advancing both scientific discovery and practical applications. New methods and technologies enable researchers to enhance the sensitivity, specificity, and efficiency of PCR, making it a more robust tool for various applications.
Next-Generation PCR Techniques
Next-generation PCR techniques have emerged as pivotal advancements in the realm of molecular diagnostics and genetic research. This evolution in PCR capabilities introduces several key features that distinguish them from traditional methods. For instance, approaches like high-fidelity PCR and isothermal amplification methods, such as LAMP (Loop-mediated isothermal amplification), provide researchers with alternative ways to isolate and amplify DNA targets without the need for precise thermal cycling. These innovations can improve reaction speed and reduce the risk of contamination, which is a common concern in classical PCR.
Multiple applications benefit from next-generation techniques, particularly in situations where time is of the essence, such as during outbreak investigations or when analyzing environmental samples. Moreover, these methods often exhibit increased tolerance to inhibitors, making them suitable for use with complex biological matrices.
Automation and High-Throughput Systems
The integration of automation and high-throughput systems represents a significant leap forward in the efficiency of PCR processes. Automated platforms allow researchers to perform multiple PCR reactions simultaneously, dramatically increasing sample throughput and reducing hands-on time. This shift is especially important in clinical diagnostics and pharmaceutical development, where the rapid processing of numerous samples can be critical.
With automated systems, researchers can also reduce human error and improve reproducibility across experiments. The capability to up-scale PCR reactions via high-throughput technology fosters more comprehensive studies and accelerates the pace of scientific inquiry.
Consider the potential of automated qPCR systems, which can provide real-time results with unprecedented accuracy. This seamless integration of technology not only enhances data quality but tends to have a favorable impact on the overall workflow and turnaround time in laboratories.
Integration with CRISPR Technologies
The marriage between PCR and CRISPR technologies is something akin to a match made in scientific heaven. As CRISPR-based genome editing techniques gain traction, the role of PCR as a supporting method has become ever more pronounced. PCR is invaluable in the preparation of templates for CRISPR, allowing researchers to amplify specific sequences that require editing.
In addition, novel PCR strategies are being developed to facilitate the detection of CRISPR-induced changes in target DNA sequences. Techniques like PCR-based detection assays, which identify modified loci, can directly link gene editing outcomes to measurable results, thereby enriching our understanding of gene function.
Overall, integrating CRISPR with innovative PCR approaches adds a dimension to genetic research that holds promise for everything from therapeutic applications to agricultural improvements.
"The future of PCR technology is intertwined with advancements in genomic editing, leading to new horizons in both research and practical applications."
With such far-reaching implications, it’s clear that innovation in PCR technology will serve as a cornerstone for future breakthroughs in molecular biology.
Challenges and Considerations
In the realm of thermal PCR, grappling with various challenges is as unavoidable as a rainy day in spring. While the method stands as a beacon of innovation, several hurdles can complicate its implementation. Addressing these challenges is vital for ensuring that the technology achieves its full potential in both research and applied settings. Here, we’ll unpack the considerations around contamination, primer design, and temperature control, giving a clearer picture of the nuances at play.
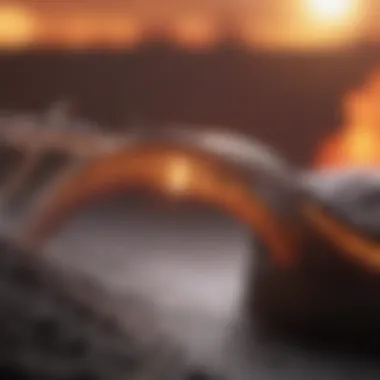
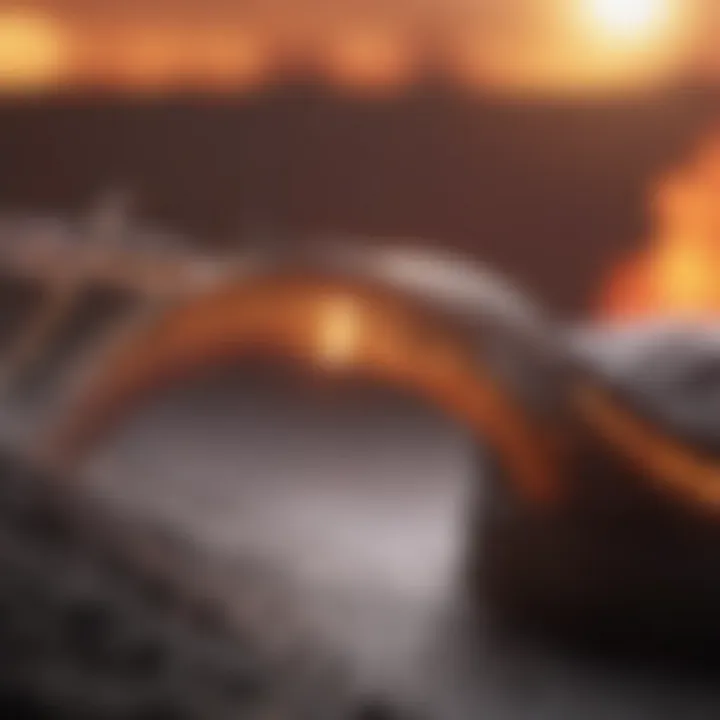
Contamination Issues
Contamination in thermal PCR can wreak havoc, much like a thorn in a rosebush. Even minute amounts of foreign DNA can produce misleading results, throwing a spanner in the works of meticulous research. Sources of contamination can range from environmental elements, such as airborne particles, to human errors during sample handling.
To mitigate these complications, labs often adopt strict protocols. These might include:
- Dedicated equipment: Using separate tools for different samples.
- Protective barriers: Employing clean rooms and air filters can reduce unwanted exposure.
- Regular decontamination: Cleaning surfaces and tools with UV light can help eradicate any residual DNA.
Addressing contamination effectively not only bolsters the reliability of experimental outcomes but also enhances the integrity of the data collected.
Primer Design Challenges
The role of primers in thermal PCR can’t be overstated; they are the unsung heroes that initiate the DNA synthesis process. However, designing primers is not as straightforward as one might think. A poorly designed primer can lead to non-specific binding, resulting in a smorgasbord of amplified products rather than the desired sequence.
A few critical factors to consider during primer design include:
- Melting temperature (Tm): A mismatch in Tms can hinder the efficiency of the PCR. Generally, primers should have a Tm between 55°C to 65°C.
- GC content: An ideal GC content ranges between 40% and 60%; too high or too low can affect stability.
- Length: Primers are typically 18 to 24 nucleotides long, allowing for specificity while maintaining the ability to anneal effectively.
Given these complexities, it’s often prudent to use software tools that assist in the design process. This step can significantly save time and improve results, helping researchers avoid common pitfalls.
Temperature Control Limitations
Temperature control is the linchpin of thermal PCR—if not done properly, the entire process can collapse like a house of cards. The precision required to cycle through denaturation, annealing, and extension phases is paramount. Variations in temperature can lead to inefficient amplification or, worse, no amplification at all.
Several key issues arise from temperature control:
- Equipment Calibration: PCR machines need to be calibrated regularly; without proper calibration, the temperatures can drift, leading to unreliable results.
- Block Uniformity: Hot or cold spots in the thermal block can yield inconsistent results across samples, necessitating checks and tests before proceeding.
- Time Management: Each phase's duration must be monitored closely. Being off by just a minute can adversely affect yield and specificity.
In summary, navigating the pitfalls of contamination, primer design, and temperature regulation require a keen understanding of the thermal PCR process. Success hinges on vigilance and methodological rigor, ensuring that findings are both accurate and reproducible. Without this careful attention, the magnificent capabilities of thermal PCR may be diminished, leaving researchers with less than stellar outcomes.
Future Directions in Thermal PCR
The exploration of thermal Polymerase Chain Reaction (PCR) continues to evolve, threaded deeply into the fabric of molecular biology. As we look ahead, understanding the future directions of thermal PCR isn't just an exercise in speculation—it's a roadmap towards broadening its horizon and enhancing its utility. Advances in technology and a growing awareness of practical applications promise to expand how researchers approach PCR methodology. The emphasis on innovation not only addresses current limitations but also paves the way for groundbreaking developments in diagnostics, gene editing, and more.
Potential New Applications
One area that could see considerable growth is the application of thermal PCR in point-of-care testing. With ongoing advancements, the dream is that PCR will no longer be confined to laboratory settings, but instead, can be deployed swiftly wherever needed—be it in remote clinics, emergency rooms, or even at home. This shifts the paradigm from centralized testing to decentralized, on-the-spot diagnostic capabilities.
Key fields for potential new applications include:
- Infectious Disease Detection: Rapid identification of pathogens using portable devices, enhancing response times during outbreaks.
- Food Safety Monitoring: Utilizing PCR for quick screening of bacterial contamination in food products, safeguarding public health.
- Personalized Medicine: Tailoring treatments based on individual genetic profiles, thus enhancing therapeutic efficacy.
Adopting these new applications involves not just technological innovation but also thoughtful integration into existing healthcare frameworks to ensure effectiveness.
Advancements in Sensitivity and Specificity
The continual pursuit of improved sensitivity and specificity in PCR techniques will significantly impact the quality of results generated. As technologies advance, researchers are looking at ways to refine these crucial parameters.
Enhanced sensitivity means the ability to detect lower quantities of target nucleic acids, which is especially vital in early detection of diseases, like cancer or autoimmune conditions. This could lead to life-saving interventions at a much earlier stage than currently possible.
On the flip side, increasing specificity reduces the risk of false positives—ensuring that test results are not only accurate but also reliable. Techniques such as the development of more sophisticated probes and primers can play a pivotal role. Potential advancements might include:
- New Polymerases: Innovations in enzymes that exhibit higher fidelity can lead to better replication of DNA sequences without errors, hence improving overall output quality.
- Digital PCR Techniques: New approaches like this can quantify DNA with unparalleled precision, further enhancing the analytical capabilities of PCR.
In summary, as this field matures, the exploration of potential applications coupled with advancements in sensitivity and specificity will undoubtedly shape the future landscape of thermal PCR. The implications for science, healthcare, and even our daily lives could be profound.
Closure
The conclusion serves as the final brushstroke on the intricate canvas that is thermal PCR. It's more than just a summary; it's a reflection on the technology's evolving essence and its significant role in shaping scientific inquiry. This discussion delves into critical elements essential for understanding thermal PCR's impact on molecular biology.
Recap of Key Points
Thermal PCR has undeniably revolutionized several fields with its adaptability and precision. Here are the most salient points we've covered:
- Foundational Principles: The basic mechanisms including the importance of DNA templates, primers, and polymerases.
- Applications: The versatility of PCR in genetic research, clinical diagnostics, forensic analysis, and environmental monitoring.
- Types of PCR Techniques: Variations like quantitative PCR and digital PCR showcase ongoing innovation.
- Challenges: Evaluating barriers like contamination and primer design is crucial for future advancements.
- Future Directions: The potential expansion into new applications and enhancements in sensitivity and specificity are on the horizon.
Each component we examined sheds light on the multi-faceted nature of thermal PCR, revealing its capacity to adapt and respond to emerging scientific demands.
The Ongoing Impact of Thermal PCR
Thermal PCR is not merely a tool but a transformative force in the realm of molecular biology. Its ongoing impact resonates across multiple disciplines, affecting a range of scientific fields:
- Clinical Diagnostics: Fast and reliable diagnostics for infectious diseases can be achieved via techniques like RT-PCR, saving countless lives.
- Forensic Science: The precision of DNA amplification in criminal investigations has revolutionized how cases are solved, evolving justice systems worldwide.
- Environmental Monitoring: As the world grapples with ecological issues, thermal PCR offers solutions in monitoring biodiversity and tracking pathogens in various ecosystems.
The footprints of thermal PCR in the sands of scientific progress are indelible. As technology advances, its integration with new methodologies, such as CRISPR and automation, makes its application even broader, transforming not only how we conduct research but also how we approach health and environmental challenges. Indeed, the journey of thermal PCR is far from over, and its potential to reshape our future in molecular biology is as promising as ever.