Understanding Acid Phenol Chloroform in Molecular Biology
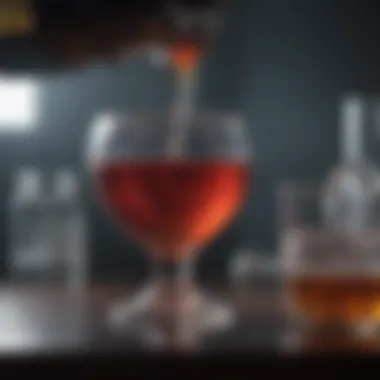
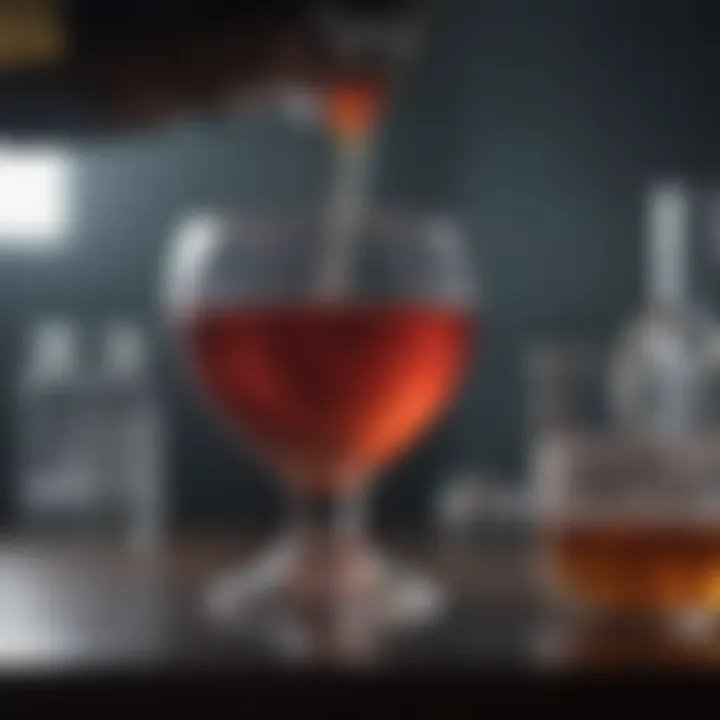
Intro
Acid phenol chloroform (APC) extraction has long been a cornerstone in molecular biology, primarily recognized for nucleic acid purification. This technique, rooted in a powerful interaction between organic solvents and biological substrates, serves as a critical tool, simplifying the complex processes involved in preparing DNA and RNA samples for various experiments and analyses.
While its chemical composition may seem straightforward at first glance, the method's effectiveness relies heavily on the principles of solubility and partitioning. The interplay of acidity, phenol, and chloroform creates the perfect environment for selectively isolating nucleic acids from proteins and other cellular debris. Such specificity is paramount, especially in sensitive applications like sequencing, cloning, and gene expression analysis, where purity plays a vital role in achieving reliable results.
In the following sections, we will delve deeper into the functional aspects of APC extraction. We'll uncover the method's strengths, illuminating its relevance in laboratory settings and research environments. Furthermore, we'll address its challenges and compare it to alternative purification techniques, establishing a holistic picture of its role in molecular biology.
Key advancements and future directions will also be discussed, emphasizing how APC extraction remains a dynamic and potent strategy for researchers in the field.
Preface to Acid Phenol Chloroform Extraction
Acid phenol chloroform extraction, often abbreviated as APC extraction, is an essential technique in the realm of molecular biology. Its vital importance lies in its ability to efficiently isolate nucleic acids and proteins from complex biological samples. This method, while perhaps not the most glamorous in the lab, is a cornerstone that enables researchers to perform downstream applications such as cloning, sequencing, and gene expression analysis.
The APC method is favored for several reasons. First, its relatively straightforward protocol allows researchers to obtain high-purity nucleic acids and proteins, which are crucial for accurate experimental results. The technique employs a mixture of phenol and chloroform, which leads to phase separation, allowing for the effective partitioning of biological molecules based on their solubility. In this article, we will explore the intricacies of APC extraction, shedding light on its history, chemical composition, mechanism of action, and practical applications.
Historical Background
The roots of acid phenol chloroform extraction can be traced back to the mid-20th century, a time when molecular biology was rapidly evolving. Initially, biochemists relied on cumbersome protocols that often yielded impure samples, impairing their research outcomes. The introduction of the APC method transformed the landscape, presenting an efficient, reliable means of nucleic acid and protein purification.
Notably, this method gained significant attention in the 1970s when molecular biologists began to recognize the importance of purity in their experiments. The technique's efficacy was established by pioneering researchers who demonstrated that by harnessing the properties of acidic phenol and chloroform, it was possible to selectively isolate DNA and RNA with minimal contamination.
Chemical Composition of APC
At the heart of the acid phenol chloroform extraction method is its unique chemical composition. The primary components of APC are:
- Acidic Phenol: This compound serves as the key solvent, offering a polar environment that facilitates the extraction of nucleic acids. Its acidity helps to denature proteins and stabilize the nucleic acids, ensuring they remain intact during the extraction process.
- Chloroform: Non-polar in nature, chloroform assists in the separation process by creating a distinct phase for lipids and proteins. This property is crucial, as it allows for the efficient partitioning away from the nucleic acids.
- Buffered Solution: Often, a buffered solution is employed to maintain a consistent pH during the extraction. This buffering is essential because fluctuations in pH can lead to the degradation of sensitive nucleic acids.
Together, these components work in harmony to create an effective extraction environment, where biological macromolecules can be isolated while minimizing contamination.
"APC extraction is a balance of chemistry and technique, enabling researchers to peel back the layers of biological complexity to obtain pure samples for analysis."
In summary, understanding the historical context and the precise chemical makeup of acid phenol chloroform extraction lays the groundwork for appreciating its profound impact on molecular biology. As we delve deeper into the mechanisms of action and the practical applications of this method, it becomes clear how integral APC extraction is to the scientific community.
Mechanism of Action
Understanding the mechanism of action behind acid phenol chloroform extraction is akin to grasping the essence of a well-played symphony. Each component—the acid, phenol, chloroform, along with the cellular materials—must harmonize to produce a remarkable result. The following sections delve into the specifics of how this extraction method separates valuable biomolecules from complex mixtures, determining its efficacy in molecular biology.
Phase Separation Process
At the heart of the APC extraction method lies the phase separation process. By introducing the acid phenol compound into a mixture, a concerted battle between the aqueous and organic phases begins. The interaction leads to a stratification where components segregate based on their molecular properties.
- Mechanism of Segregation: Upon mixing, the sample's proteins start denaturing in the acidic environment created by phenol. Meanwhile, nucleic acids, predominantly DNA and RNA, remain soluble in the aqueous layer because of their hydrophilic nature. The chloroform plays a crucial role as it increases the efficiency of this separation, making it easier for the desired molecules to be extracted.
- Visualizing the Layers: After centrifugation, one can observe three distinct layers. The topmost layer comprises the aqueous phase, which contains the nucleic acids. The middle layer may consist of proteins and denatured materials, while the bottom layer is mainly chloroform with residual cellular debris. This visual cue is vital for researchers when performing the extraction, as it directly informs which layer to collect for further analysis.
This phase separation not only underlines the efficiency of the extraction process but also minimizes the risk of contamination, making it a preferred method in labs worldwide.
Role of pH in Extraction Efficiency
The pH level during the extraction process cannot be understated. It remains a pivotal player, impacting the stability and solubility of the various biomolecules involved.
- Optimal pH Range: Generally, an acidic pH helps in denaturing proteins while keeping nucleic acids intact. This is because nucleic acids are less prone to degradation in acidic conditions. For instance, keeping a pH around 4.5 maximizes the extraction of high-quality RNA and DNA.
- pH and Molecular Integrity: If the pH strays too far from this optimal range, one might face challenges such as potential hydrolysis of nucleic acids. This underscores the importance of monitoring the pH closely during extraction.
- Buffering Systems: In many laboratories, researchers often use buffering agents to maintain desired pH levels throughout the extraction process. By carefully choosing these agents, the extraction yields can be significantly improved, therefore enhancing the overall efficacy.
"The choice of pH is as critical as the choice of the extraction solvent itself; it is instrumental in achieving the best possible results in nucleic acid recovery."
In summary, grasping the mechanism of action behind acid phenol chloroform extraction is essential for anyone looking to leverage this approach in molecular biology. By understanding the phase separation process and the influence of pH, researchers can effectively harness the power of this versatile extraction method.
Protocol for APC Extraction
The protocol for Acid Phenol Chloroform (APC) extraction is a cornerstone in molecular biology techniques, well-known for its effectiveness in isolating nucleic acids and proteins. The process is not just a routine task; it’s a meticulous orchestration of several critical steps that can greatly influence the purity and yield of the extracted molecules. Proper adherence to the protocol ensures that researchers consistently achieve reproducible results, which can be a game-changer in experimental outcomes.
Material Preparation
Material preparation is the foundation upon which successful APC extraction stands. The entire procedure hinges on having the right tools and reagents at hand. Here are the key components:
- Phenol: Commonly used for its ability to disrupt cellular membranes and denature proteins.
- Chloroform: Enhances the separation of different phases during extraction, optimally isolating nucleic acids.
- Buffers: Phosphate-buffered saline (PBS) or Tris buffers maintain the desired pH level crucial for the integrity of nucleic acids.
- Samples: Cell or tissue samples should be collected in an appropriate medium to minimize degradation.
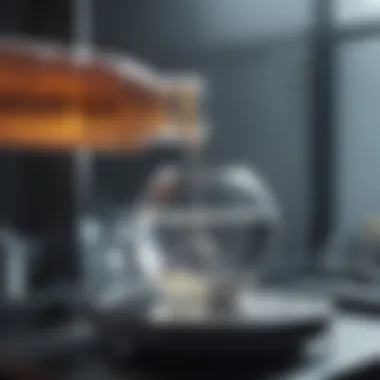
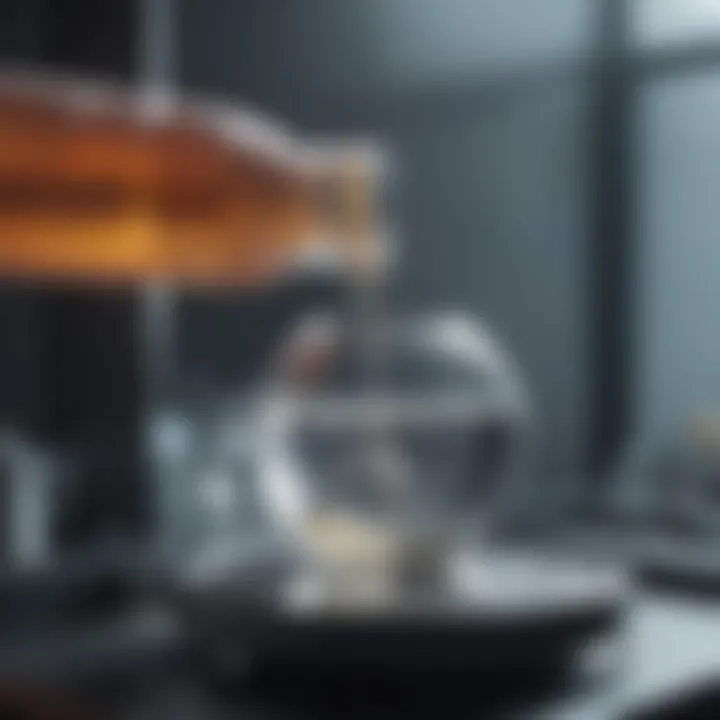
Preparation also includes ensuring all glassware and pipettes are clean and, if possible, pre-treated. This minimizes any contamination risk, which can derail the results. A detailed checklist can help to ensure all materials are ready, preventing last-minute scrambles.
Step-by-Step Extraction Process
The step-by-step extraction process from APC requires careful attention to maintain efficiency and integrity of the sample. Here’s how it typically unfolds:
- Sample disruption: Start by homogenizing the biological sample in a suitable buffer. This could be via physical means such as grinding or using a homogenizer.
- Addition of Phenol and Chloroform: Mix the homogenate with an equal volume of phenol and chloroform. This is crucial for separating the components.
- Vortexing: Vortex the mixture for a few seconds to ensure thorough mixing. This step is critical as it allows for optimal phase separation.
- Centrifugation: Spin the mixture in a centrifuge. This will separate the contents into distinct layers: the aqueous phase (containing nucleic acids), the interphase with proteins, and the organic phase with lipids.
- Aliquoting the Aqueous Phase: Carefully pipette the aqueous layer containing nucleic acids into a fresh tube without disturbing the other layers.
- Precipitation: To further purify the nucleic acids, add isopropanol or ethanol to the aqueous phase and allow the solution to sit at -20°C, often overnight, to precipitate the nucleic acids.
- Washing: Following precipitation, wash the nucleic acids with cold ethanol, followed by air drying to remove residual alcohol. Finally, re-suspend in a suitable buffer for downstream applications.
Post-Extraction Handling
Post-extraction handling is paramount to maintaining the integrity and stability of the purified nucleic acids and proteins. Here are key considerations:
- Storage: Proper storage conditions are essential. Samples should be stored at -80°C for long-term storage, which effectively minimizes degradation.
- Quality Assessment: Conducting a spectrophotometric analysis can help assess purity and concentration. Measuring absorbance at 260 nm and 280 nm can indicate the presence of nucleic acids and proteins, respectively.
- Documentation: Keeping detailed records of the extraction procedure—including any deviations—ensures reproducibility and aids in troubleshooting.
"Proper post-extraction handling can save time and resources, leading to more reliable experimental outcomes."
Following the protocol with diligence not only smooths the path to successful extraction but is also vital for the next stages of research. The meticulous nature of APC extraction lays a robust framework for numerous applications in molecular biology.
Applications of APC Method
The acid phenol chloroform (APC) extraction method is a classic technique widely used in molecular biology for various applications. Its versatility allows scientists to extract and purify nucleic acids and proteins effectively. Understanding the importance of these applications can provide insight into why this method remains a staple in laboratories around the world.
Nucleic Acid Purification
The extraction of nucleic acids is one of the primary applications of the APC method. This technique ensures that RNA and DNA are isolated in a pure form, which is crucial for subsequent analyses. Whether it’s for sequencing, cloning, or quantitative PCR, the purity of nucleic acids can significantly impact experimental outcomes. In practical use, mRNA extraction from tissues using acid phenol chloroform has become a go-to method for many researchers.
- High Efficiency: APC method showcases high efficiency in isolating nucleic acids due to its dual-phase system, allowing for the separation of nucleic acids from proteins and other undesirable substances.
- Compatibility with Various Samples: This method works with a range of biological samples, including cultured cells, plant tissues, and blood, making it invaluable for different research fields.
Protein Extraction Techniques
Another key application of the APC method is in protein extraction. This technique is particularly beneficial when researchers aim to study proteins alongside nucleic acids. During the extraction process, proteins can be solubilized and separated, helping to elucidate their functions and interactions.
- Preservation of Protein Integrity: Researchers often use APC for its ability to maintain protein structure during extraction, which is crucial for downstream analyses like Western blotting or enzyme assays.
- Multi-Component Analysis: The simultaneous extraction of nucleic acids and proteins allows for comprehensive studies of biological materials, making it a favored choice when exploring gene expression and regulation.
Use in Genetic Research
Genetic research leans heavily on the capabilities of the APC method. From the exploration of gene function to the investigation of genetic variation, the purity and integrity of samples are paramount.
- Gene Expression Studies: The accurate quantification of mRNA levels can reveal insights into gene regulation under different conditions, providing a window into cellular responses.
- Mutation Analysis: Through the efficient isolation of high-quality DNA, researchers can identify and analyze mutations that contribute to various genetic disorders or traits.
In summary, the applications of the APC method in nucleic acid purification, protein extraction, and genetic research strengthen its position as an essential technique in molecular biology. These benefits underscore its importance in advancing our understanding of biological processes and mechanisms.
Advantages of Acid Phenol Chloroform Extraction
The acid phenol chloroform extraction method boasts several advantages that make it a cornerstone technique in molecular biology. The significance of these advantages can not be overstated; they contribute to its longevity and appeal in laboratories worldwide. By unpacking these benefits, we can gain a clearer view of why researchers tend to favor this method for nucleic acid and protein extraction. From its ability to yield high purity samples to its adaptability, APC extraction offers numerous perks that cater to the diverse needs of the scientific community.
High Yield of Pure Nucleic Acids
One of the most compelling benefits of using acid phenol chloroform extraction is the high yield of pure nucleic acids it produces. This method can effectively isolate DNA and RNA from a variety of biological samples, which is crucial for downstream applications such as sequencing, cloning, and amplification. Why does this matter? Well, when working with genetic material, the integrity and purity of the sample are non-negotiable.
The successful extraction of nucleic acids hinges on both the separation process and the quality of the materials used. APC extraction is designed to minimize contaminants, ensuring that the resulting nucleic acids are not only abundant but also of high quality. In a world where sample integrity can make or break experiments, APC stands out by consistently delivering on this front.
Versatility in Sample Types
Acid phenol chloroform extraction shines in its versatility. It is effective across a broad spectrum of sample types, including cell cultures, tissues, and even environmental samples. This flexibility means a researcher can use the same technique for various projects without needing to adapt to drastically different protocols. For example, whether isolating DNA from human samples or purifying RNA from bacterial cultures, APC extraction fits the bill.
Furthermore, this method is not merely confined to nucleic acid isolation; it also extends its capability towards protein extraction. Whether it's extracting proteins for western blotting or assessing enzymatic activity, the versatility of APC extraction opens many doors for diverse research needs. As a researcher, being equipped with a method that can handle different sample varieties saves time and resources, making the research process leaner and more efficient.
Cost-Effectiveness
Delving into the financial implications, acid phenol chloroform extraction is known for being a cost-effective choice. The chemicals involved, while requiring careful handling due to their hazardous nature, are relatively inexpensive when compared to some more modern extraction techniques that rely on costly kits.
In today’s research environment, budgets can be tight, and the allure of free-flowing funds is often just a dream. By opting for APC extraction, labs can achieve high-quality results without breaking the bank. This is vital in academic settings where funding sources are limited and every dime counts.
The reduced need for expensive equipment also plays into the cost-effectiveness of APC extraction. Researchers can often utilize standard lab equipment to perform the extraction, minimizing the overhead costs associated with purchasing specialized machinery.
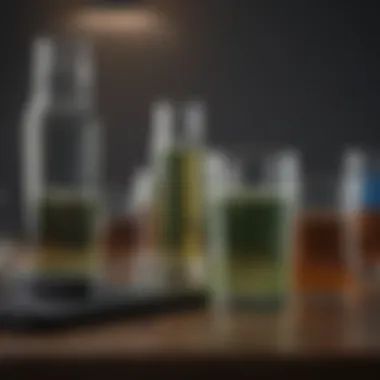
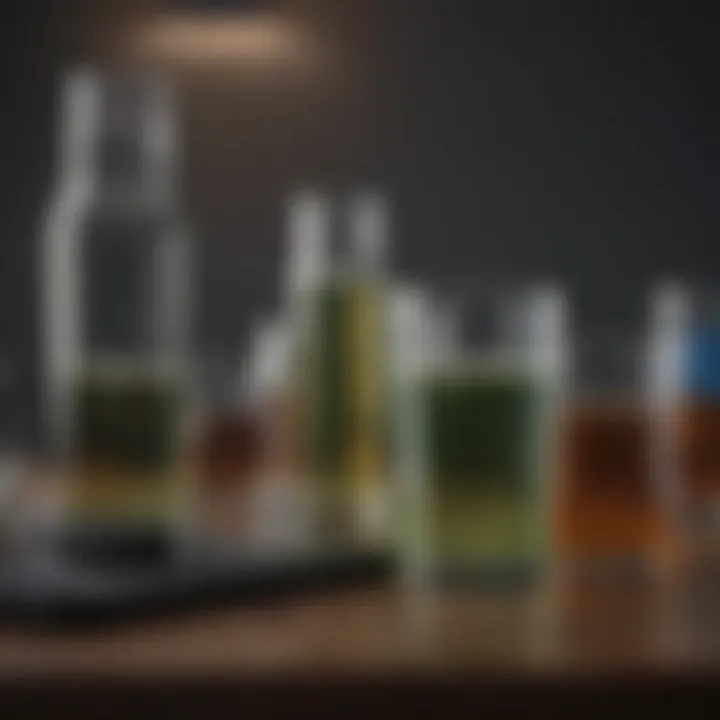
In summary, acid phenol chloroform extraction brings a trifecta of advantages to the table: high yields of pure nucleic acids, adaptability across various types of samples, and cost-effective solutions that make it particularly suited for diverse research applications.
As we navigate through molecular biology, understanding these advantages allows us to appreciate the established role of APC extraction in contributing to high-quality scientific outcomes.
Challenges and Limitations
In the field of molecular biology, while acid phenol chloroform (APC) extraction holds significant appeal due to its effectiveness, it is essential to recognize the inherent challenges and limitations it poses. Understanding these aspects is crucial for researchers who aim to refine their methodologies and enhance their outcomes, especially in a landscape where purity and yield are key metrics.
Hazardous Nature of Chemicals
The combination of acid phenol and chloroform in the extraction process presents various safety concerns. Both of these solvents are well-known for their toxicity and potential carcinogenic properties. Researchers must always handle these chemicals with care to mitigate risks. Implementing proper safety protocols, such as using fume hoods and personal protective equipment, cannot be overstated.
- Chloroform: This solvent is capable of causing significant harm to the liver and other organs. Prolonged exposure can lead to adverse health effects, necessitating thorough training for anyone working in labs that utilize this chemical.
- Phenol: Similarly, phenol’s corrosive qualities mean that skin contact or even inhalation can be hazardous. It is essential to be familiar with Material Safety Data Sheets (MSDS) for both chemicals.
In addition to direct health implications, improper disposal of these substances can lead to severe environmental impacts, further complicating their use.
Sensitivity to Contamination
While the APC extraction method delivers high yields of nucleic acids and proteins, the process is fraught with risks concerning contamination. Even minute amounts of impurities like proteins, nucleases, or contaminants from reagents can adversely affect the integrity of the samples being processed.
Successfully addressing contamination involves:
- Strict Aseptic Techniques: Enforcing rigorous aseptic techniques during extraction is essential. Using sterile equipment and reagents can prevent microbial contamination, which could ruin experimental results.
- Quality Control Measures: Implementing robust quality control protocols ensures that not only the reagents used are of high purity but also that environmental factors in the lab are monitored to avoid unwanted contamination.
- Regular Training: Continual training for lab personnel on best practices for contamination control can safeguard the integrity of the research results.
Crucially, contamination sensitivity can vary based on the samples being processed. Researchers must be aware of the unique properties and vulnerabilities of their specific samples, ensuring appropriate modifications to extraction protocols are made to account for such factors.
"In molecular biology, stability of results often resides in the minutiae of handling; neglecting such details can lead to cascading failures in research outcomes."
Understanding these challenges not only equips scholars with the knowledge to navigate potential pitfalls but also motivates them to innovate more effective solutions in their practices. With proper consideration of the hazardous nature of chemicals involved and the vigilance required to avoid contamination, the efficacy of the APC method can still shine through in the hands of skilled practitioners.
Comparative Analysis with Alternative Methods
In the realm of molecular biology, the choice of extraction techniques can often spell the difference between success and failure in obtaining high-quality biomolecules. Acid phenol chloroform (APC) extraction has established its place as a reliable method, yet it is crucial to examine it alongside various alternative techniques. This comparative analysis aims to investigate the effectiveness, usability, and outcomes of using APC in relation to methods such as silica-based columns, magnetic beads, and enzymatic protocols.
Silica-Based Columns
Silica-based columns have become a go-to for many researchers due to their straightforward application and reliability. These columns utilize a distinct principle whereby nucleic acids bind to the silica matrix under specific conditions. For instance, in high-salt environments, negatively charged nucleic acids adhere to the positively charged silica, facilitating separation due to subsequent washing steps.
Some of the notable benefits of using silica-based columns include:
- Efficiency: The process is generally quick, yielding pure nucleic acids without excessive handling.
- User-Friendly: Most protocols are designed to be straightforward, making it less daunting for novices.
- No Toxic Solvents: Unlike APC, there is no need for hazardous organic solvents, which simplifies disposal and increases safety.
However, silica-based protocols might not achieve the same yield as APC when dealing with particularly challenging samples. The limitation to processing one sample at a time further constrains throughput in busy labs.
Magnetic Beads Technology
An alternative method worth considering is the use of magnetic beads for nucleic acid extraction. This technique operates on a similar principle of affinity but uses specially coated beads that bind the target molecules. The handy aspect of this approach is its versatility; it can be applied across a wide range of samples with minimal adjustments.
Advantages include:
- High Flexibility: Magnetic beads can easily adapt to various sample origins, whether it be blood, tissue, or culture cells.
- Parallel Processing: One can process multiple samples simultaneously, enhancing efficiency.
- Reusability: Many types of magnetic beads allow for repeated use, reducing costs over time.
On the flip side, issues with bead retention and handling may cause difficulties. For instance, manipulating these small particles, especially in starting protocols, can sometimes feel like trying to herd cats.
Enzymatic Protocols
Finally, enzymatic protocols bring another perspective to the extraction table. These methods typically employ enzymes to break down cellular components selectively, thus isolating nucleic acids while reducing contamination risks. Methods like this can leverage the specificity of protein or nucleic acid targets, producing highly purified samples.
The primary benefits of enzymatic approaches are:
- Targeted Digestion: This reduces the risk of compromising nucleic acid integrity by removing contaminants intelligently.
- Lower Toxicity: Generally, there is less reliance on harsh chemicals, making it a kinder option for users and the environment.
However, enzymatic protocols can be a double-edged sword; they might require longer incubation times and specific temperature controls, which can eat into time—something labs often have in short supply!
"Choosing the right extraction method often depends not just on the desired yield but also on practicality in the lab setting."
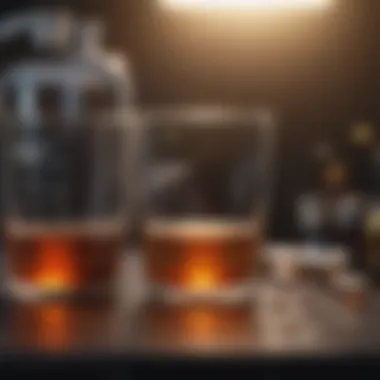
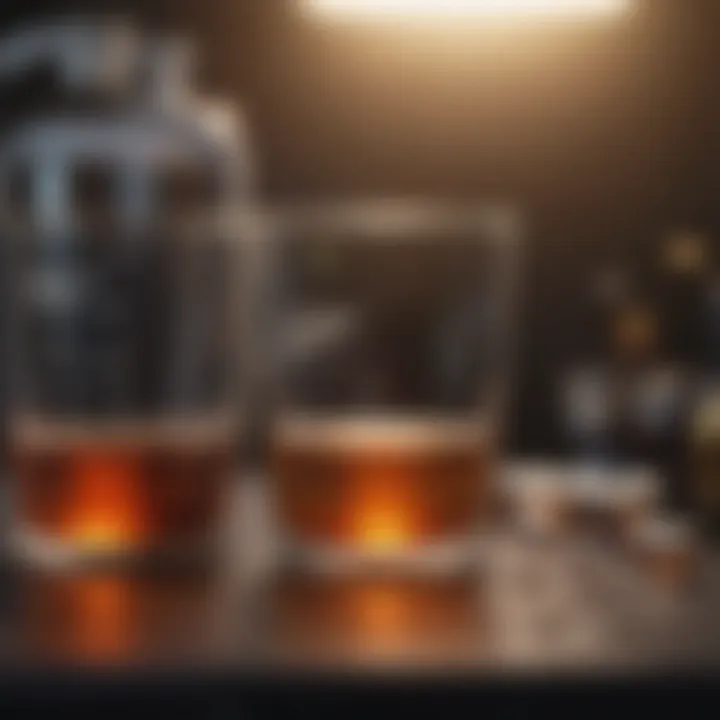
Recent Developments in APC Methodology
The field of molecular biology is constantly evolving, and the acid phenol chloroform (APC) extraction method is no exception. Recent advancements have shifted the paradigm of how this method is utilized, leading to improved outcomes in nucleic acid purification and a broader application across various scientific disciplines. Staying on top of these developments is crucial for students, researchers, and professionals alike. This section aims to explore the importance of these recent innovations and their implications for future research.
Optimizations for Enhanced Efficiency
In the realm of APC extraction, efficiency is the name of the game. Researchers are now exploring various optimization techniques to enhance the speed and yield of nucleic acids recovered through this method. Some key optimizations include:
- Refined Chemical Ratios: Adjusting the proportions of acid, phenol, and chloroform can yield better separation of nucleic acids from proteins and other cellular debris. By fine-tuning these chemical ratios, studies have shown enhanced extraction efficiency.
- Improved Phase Separation: Recent techniques utilize phase-guide agents to aid in separating the aqueous and organic layers more reliably. These advancements help to minimize cross-contamination, leading to purer nucleic acid samples.
- Temperature Control: Maintaining a specific temperature during the extraction process can have a significant impact on yield. Studies reveal that cooling the samples before extraction enhances the solubility of nucleic acids while preventing degradation.
Such advancements present a pathway towards greater efficiency and effectiveness in the use of APC extraction, making it a compelling methodology in diverse settings.
Integration with Automated Systems
As the demand for faster and more reliable molecular biology techniques increases, integrating APc extraction with automated systems has become a hot topic. Several benefits come from this merge:
- Consistency and Repeatability: Automated systems reduce human error, ensuring that each step of the extraction process is performed uniformly, thereby increasing the reliability of results.
- Time Savings: Automation streamlines the extraction process, allowing researchers to process multiple samples simultaneously. This increase in throughput is particularly vital for labs handling large-scale experiments.
- Safety Improvements: With automated systems, direct handling of hazardous chemicals like chloroform is minimized, reducing safety risks for laboratory personnel.
The incorporation of these automated solutions signifies an exciting trend toward making APC extraction more accessible and efficient. As technology advances, we can expect to see even more revolutionary changes that will further solidify the role of APC in molecular biology research.
"The ongoing advancements in APC methodology represent both a commitment to innovation and the pursuit of excellence in molecular biology experimentation. Each development opens new doors for researchers across the globe.”
With the continual refinement of techniques and technologies in APC extraction, the future is bright for molecular biology. As these practices become standard, the field will undoubtedly benefit from enhancements in both productivity and research quality.
Future Directions in APC Research
The landscape of molecular biology is ever-evolving, and the methods we deploy for extractions must keep pace with the advancing technology. As researchers continue to encounter new challenges and explore innovative applications, the role of acid phenol chloroform (APC) extraction shines through as a versatile tool. The importance of examining future directions in APC research cannot be overstated; these explorations hold the potential to augment the efficiency, effectiveness, and applicability of this methodology across various scientific arenas.
Potential Innovations in Extraction Techniques
As we gaze into the crystal ball of molecular biology, several potential innovations in extraction techniques promise to revolutionize the conventional use of APC. For one, enhancements in the formulation of reagents may increase the specificity and yield of nucleic acids and proteins. Incorporating novel compounds that improve the interaction between biomolecules and the extraction system could lead to higher purity levels, making downstream applications more successful.
Moreover, the integration of microfluidic technologies represents an exciting frontier. By miniaturizing the extraction process, researchers could achieve quicker results while using smaller sample volumes. This would not only cater to scarce sample situations but also appeal to fields focused on precision, such as personalized medicine.
Lastly, the use of targeted extraction techniques utilizing antibodies or aptamers to isolate specific biomolecules could streamline the process, thus minimizing unwanted contaminations during multistep procedures. In essence, such innovations could make APC a powerhouse for future molecular investigations.
Exploration of APC in Diverse Disciplines
The vast applications of APC are not limited to traditional molecular biology; this method can be pivotal in various other fields. The exploration of APC in diverse disciplines highlights its adaptability and potential for broader research relevance.
In the realm of environmental science, for instance, APC can assist in extracting genetic material from soil samples. This could pave the way for studies investigating microbial diversity and ecosystem health.
In agriculture, incorporating APC into the extraction of agricultural samples could enhance breeding programs by yielding high-quality DNA from diverse plant species for genetic mapping.
Furthermore, the medical field stands to benefit significantly. The extraction of viral RNA or bacterial DNA through APC techniques may assist in the early detection of infectious diseases or in developing therapeutic approaches through better understanding of pathogen behavior.
"The potential applications of APC beyond molecular biology are immense, showcasing its versatility as a fundamental extraction method."
In summary, the future directions of APC research involve not only refining existing methodologies but also venturing into unexplored territories. As the scientific community collaborates and innovates around APC, the benefits can resonate through numerous fields, reinforcing its standing as an irreplaceable tool in the research toolkit.
End
The role of acid phenol chloroform (APC) extraction in molecular biology cannot be overstated. This method has become a foundational technique, critical for researchers in fields ranging from genetics to biochemistry. Its ability to efficiently extract nucleic acids from various sample types makes it indispensable.
Summative Insights on APC Method
The APC method offers a suite of benefits that stand out in laboratory settings. Firstly, its high recovery rate of nucleic acids ensures that researchers can obtain sufficient quantities for downstream applications. Whether purifying DNA from tissue samples or isolating RNA from cultured cells, APC consistently delivers high-quality material.
Furthermore, its versatility allows for use across diverse biological samples, including bacterial cultures, plant tissues, and animal cells. In terms of practical execution, the protocol for APC extraction is straightforward, making it accessible to both novice and experienced practitioners. The phase separation relies on a combination of organic solvents, which effectively segregate biological molecules based on their physical and chemical properties. This method of extraction is not only efficient but also minimizes the loss of precious biological samples.
"The acid phenol chloroform method is a prime example of how traditional techniques have held their ground in the face of modern innovations. Its reliability remains a beacon in the turbulent sea of molecular extraction methods."
Implications for Future Research
As we delve deeper into genomic research and personalized medicine, the relevance of APC extraction will likely expand. Future studies could focus on optimizing the protocol to minimize hazard—perhaps by integrating safer solvents or by exploring new phases of phase separation. There’s also a growing interest in automating the APC extraction process. With technological advancements, the integration of robotics in laboratories could streamline the extraction workflow, allowing scientists to focus on analysis rather than technique.
Moreover, the exploration of APC's applicability in newly emerging fields, such as
- metagenomics,
- transcriptomics, and
- proteomics
is ripe for investigation. As researchers strive for faster and more reliable methodologies, assessing APC’s adaptability may pave the way for innovative discoveries. The intersection of APC methodology with advancements in artificial intelligence and computational biology delivers exciting prospects, making it a significant avenue for exploration in coming years.