Understanding Biomedical Engineering: An In-Depth Exploration
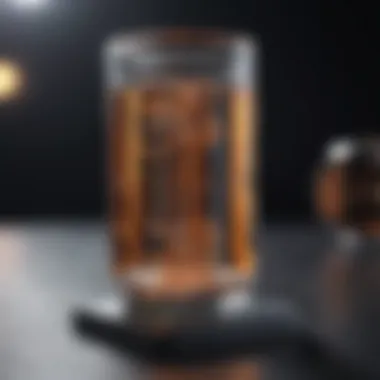
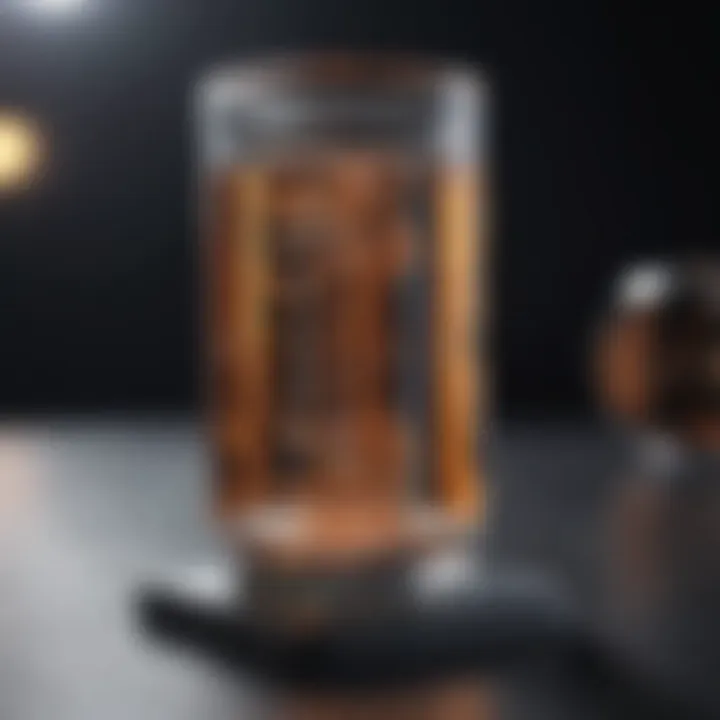
Intro
Biomedical engineering integrates the principles of engineering with biomedical sciences to create solutions that improve healthcare and the quality of life for patients. In recent years, this discipline has gained significant traction, leading to advancements that range from sophisticated imaging techniques to the development of innovative medical devices.
With this growing importance, it is essential to unpack the various facets of biomedical engineering. This article will explore crucial areas, such as the educational routes one can pursue, the skills required to thrive in this field, and the innovations that are reshaping the medical landscape. Additionally, it touches on where the field is headed in the future and its implications for society as a whole.
Understanding biomedical engineering requires a focus on its relevance within both healthcare and technology. The roles played by biomedical engineers are not just limited to creating devices; they also include collaborating with medical professionals, conducting research, and even guiding regulatory pathways for new medical products. As readers delve into this exploration, they will discover insights and knowledge necessary to navigate this complex and impactful discipline.
What is Biomedical Engineering
Biomedical engineering is an interdisciplinary field that merges principles from engineering with the biological and medical sciences. It plays a pivotal role in developing technologies that enhance healthcare and improve patient outcomes. Its significance lies not only in creating devices or processes but also in addressing complex challenges within the healthcare system. From designing prosthetics to crafting advanced imaging modalities, biomedical engineering impacts people's lives directly.
Definition of Biomedical Engineering
Biomedical engineering can be defined as the application of engineering principles and techniques to the medical and biological fields. This discipline aims to solve medical problems through innovation, often leading to improved health care solutions. Biomedical engineers engage in a wide variety of activities, from designing and manufacturing medical instruments to conducting research on medical devices and biotechnology. This varies aspect allows for a unique blend of creativity and technical knowledge.
Historical Development
The evolution of biomedical engineering reflects advances in technology and medicine. Initially, the integration of engineering with medicine emerged prominently during the late 19th and early 20th centuries. The invention of the electrocardiogram in the 1900s showcased the potential for engineering to influence clinical practices. Over decades, as medical knowledge expanded alongside the development of electronics and computer technology, biomedical engineering began to take shape as a distinct field.
In the 1960s and 1970s, formal educational programs began to appear, resulting in increased recognition of the profession. Organizations like the IEEE Engineering in Medicine and Biology Society were established to foster growth in this area. Today, biomedical engineering encompasses a vast array of sub-disciplines, reflecting the complexity of human health and the variety of techniques available for addressing these challenges.
This blend of history and definition illustrates the ongoing journey of biomedical engineering. As technology progresses, so does the field, continually adapting to address emerging health issues and enhancing the quality of life.
Key Components of Biomedical Engineering
Understanding the key components of biomedical engineering is vital for appreciating how this field influences both clinical practices and technological advancements. These components include biomedical devices, biomaterials, tissue engineering, and medical imaging. Each area represents a significant domain within the industry, contributing uniquely to improving patient outcomes and enhancing medical capabilities.
Biomedical Devices
Biomedical devices form the backbone of this discipline. They range from simple tools, like thermometers, to complex machines such as MRI scanners. These devices are crucial in diagnosing, monitoring, and treating health conditions. Their development often requires a multidisciplinary approach that combines engineering principles with medical knowledge. As the technology evolves, the importance of design and functionality becomes critical. The effectiveness of a biomedical device not only impacts patient care but also influences workflow efficiency in healthcare settings.
Modern advancements, such as smart wearables and telehealth devices, exemplify how innovation in biomedical devices can enhance healthcare accessibility. For instance, devices like glucose monitors provide real-time data that helps diabetic patients manage their health more effectively.
Biomaterials
Biomaterials are substances engineered to interact with biological systems. These materials can be natural or synthetic and are employed for various applications, including implants, drug delivery systems, and tissue repair. The choice of biomaterial is essential for ensuring biocompatibility, which refers to the material's ability to perform its desired function in the body without eliciting an adverse reaction.
Different types of biomaterials include metals, ceramics, and polymers. Each has its own set of properties that can be tailored according to specific medical needs. For example, titanium is often used for implants due to its strength and compatibility with bone tissue, while hydrogels are favored for drug delivery due to their ability to maintain a moist environment. Advances in nanotechnology have further enriched this area, opening new possibilities for targeted and effective therapies.
Tissue Engineering
Tissue engineering merges biology and engineering to create biological substitutes that restore or improve tissue function. This innovative approach holds the potential to revolutionize medicine by enabling the regeneration of damaged tissues and organs. Techniques in this field often involve seeding human cells onto scaffolds made from biomaterials.
The significance of tissue engineering is profound. It not only addresses the shortage of organ donors but also reduces the risk of transplant rejection. Researchers are investigating various cell types, including stem cells, to enhance the regenerative capacity of engineered tissues. The challenges in this field include ensuring vascularization and proper integration with host tissues.
Medical Imaging
Medical imaging is a cornerstone of modern diagnostics. It encompasses techniques that allow health professionals to visualize the interior of a body for clinical analysis and medical intervention. Key modalities include X-rays, computed tomography (CT) scans, magnetic resonance imaging (MRI), and ultrasound. Each of these technologies provides unique insights that guide diagnosis and treatment planning.
The role of biomedical engineers in medical imaging is crucial for the development of advanced imaging technologies that improve resolution and reduce patient exposure to harmful radiation. Innovations such as functional MRI and molecular imaging are expanding the boundaries of how we understand diseases, particularly in oncology and neurology.
"Biomedical engineering is fundamental to advancing healthcare through creating and improving medical devices, biomaterials, and imaging technologies."
Educational Pathways in Biomedical Engineering
The field of biomedical engineering requires a robust educational foundation that equips students with essential skills and knowledge. Understanding these pathways is crucial for those interested in this discipline, as they highlight the various stages and opportunities for growth. Biomedical engineering combines principles from both engineering and biological sciences, demanding a diverse educational approach. This section explores the key educational pathways one may take, focusing on undergraduate programs, graduate studies, and essential certifications.
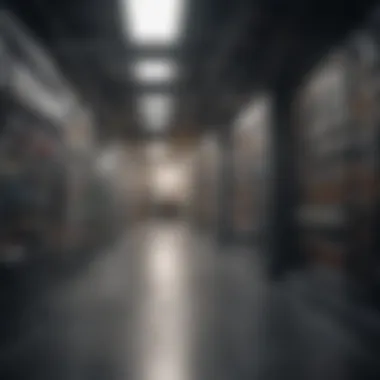
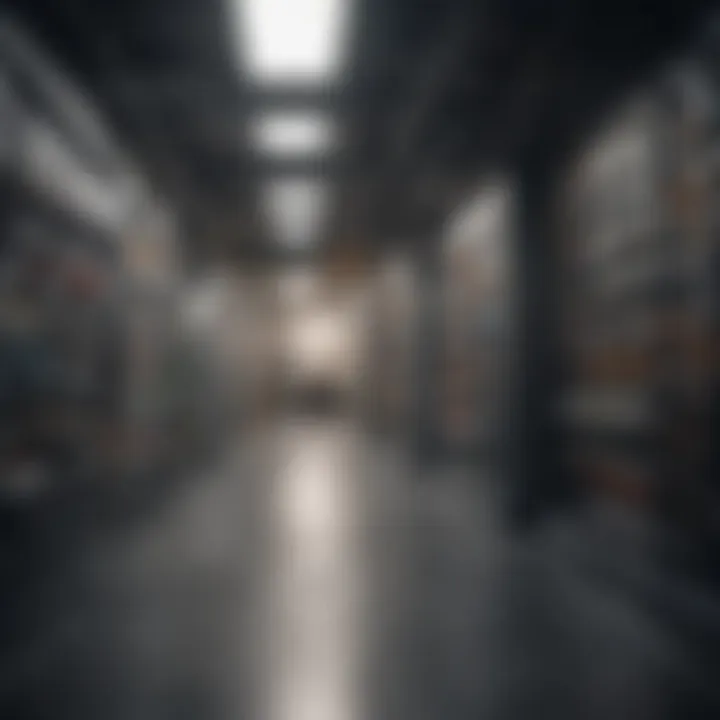
Undergraduate Programs
Undergraduate programs in biomedical engineering generally offer a Bachelor of Science degree. These programs combine core engineering principles with specific studies related to biology and medicine. Students typically take courses in mathematics, physics, chemistry, and biology, alongside specialized subjects such as biomechanics, biomaterials, and medical imaging. A strong emphasis on laboratory work is also a hallmark of these programs, fostering hands-on experience with technology and techniques used in real-world biomedical applications.
The undergraduate experience is not limited to academic coursework. Many universities encourage involvement in research projects, internships, and co-op programs. Such experiences allow students to apply what they learn in the classroom to practical settings. This is highly beneficial for those preparing for careers in sectors such as healthcare, device manufacturing, and regulatory affairs.
Graduate Programs
Upon completion of an undergraduate program, many biomedical engineers choose to pursue graduate studies. Graduate programs often offer a Master’s or Doctoral degree in biomedical engineering or related fields. These programs provide an opportunity for deeper specialization, allowing students to focus on particular areas such as bioinformatics, tissue engineering, or medical robotics.
Graduate programs typically involve rigorous coursework, research, and thesis elements. Students work closely with faculty members on innovative research projects, contributing to advancements in biomedical technology and practices.
Advanced degrees can lead to higher-level job prospects, including positions in research and development, clinical consultancy, and leadership roles in healthcare organizations. Moreover, obtaining a PhD can pave the way for academic careers, particularly in teaching and research.
Certifications and Specializations
Certifications in biomedical engineering are available and essential for those looking to advance their careers. Different organizations offer various certification programs focusing on specific skills or areas of expertise within the field. For example, certifications such as Certified Biomedical Equipment Technician (CBET) or Certified Clinical Engineer (CCE) can enhance job prospects and demonstrate a professional's commitment to ongoing education and training.
Additionally, pursuing specialization in particular technologies or methods, such as medical imaging techniques or regulatory compliance, can set professionals apart in the competitive job market. This can be achieved through short courses, workshops, and online platforms, which provide flexible learning opportunities that fit into a busy professional life.
"Continuous learning and adaptation are essential for success in the rapidly evolving field of biomedical engineering."
Core Competencies of Biomedical Engineers
The role of biomedical engineers encompasses a variety of responsibilities, which necessitate a diverse set of core competencies. These competencies are critical in ensuring that engineers can effectively translate scientific knowledge into innovative solutions for healthcare challenges. By possessing these skills, biomedical engineers can contribute significantly to advancements in medical technology and patient care.
Technical Skills
Technical skills form the backbone of a biomedical engineer's expertise. This includes proficiency in areas such as programming, data analysis, and hands-on technical abilities with medical devices. Biomedical engineers often work with complex equipment, requiring a thorough understanding of both hardware and software.
- Programming Languages: Mastery in languages like Python, C++, or MATLAB is essential for modeling biological systems or developing software for medical devices.
- Device Development: Practical knowledge in designing and testing medical devices is crucial. Understanding regulations and compliance enhances their ability to work within the healthcare sector.
These technical skills enable biomedical engineers to innovate efficiently, facilitating the creation of more effective medical solutions.
Analytical Skills
Analytical skills are equally important. Biomedical engineers must analyze data, assess problems, and devise suitable solutions. This process often involves interpreting complex datasets and making decisions based on empirical evidence.
- Problem Solving: Engineers face various challenges, from optimizing treatment plans to integrating new technologies into existing systems. A systematic approach can lead to successful resolutions.
- Critical Thinking: The ability to evaluate different approaches and understand their implications is vital. Analyzing potential risks or the ethical considerations of new technologies also falls under this domain.
Strong analytical skills greatly enhance a biomedical engineer's capability to innovate and improve patient outcomes.
Communication Skills
Lastly, communication skills must not be overlooked. Biomedical engineers often serve as a bridge between technical teams and non-technical stakeholders, such as healthcare providers and patients.
- Collaboration: Working with multidisciplinary teams is common. Clear communication is essential for aligning goals and ensuring that all parties understand the technical aspects involved in projects.
- Documentation: Writing clear documentation and reports is important for regulatory submissions or for conveying research findings. Properly communicating complex ideas in simple terms helps foster understanding and trust between engineers and medical professionals.
Effective communication supports the successful implementation of biomedical engineering solutions while promoting teamwork and collaboration across various sectors.
"Core competencies shape the foundation for effective practice in biomedical engineering, impacting the quality of care delivered to patients."
In summary, the core competencies of biomedical engineers—technical skills, analytical skills, and communication skills—are essential for the advancement of the field. These competencies enable engineers to tackle complex challenges and foster innovation in healthcare.
Roles of Biomedical Engineers
Biomedical engineers play an essential role in bridging the gap between healthcare and technology. Their multifaceted responsibilities contribute significantly to advancements in medical practices and patient outcomes. Understanding their roles is vital for appreciating how this profession fosters innovation, addresses healthcare challenges, and enhances the quality of care. The involvement of biomedical engineers spans various sectors including research, clinical settings, and regulatory bodies, each highlighting significant areas of impact.
Research and Development
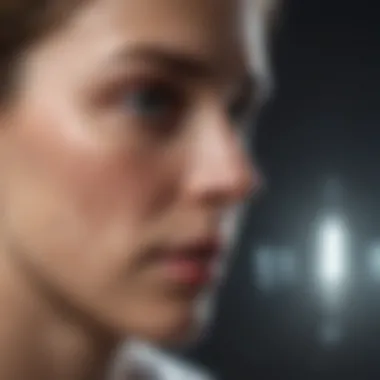
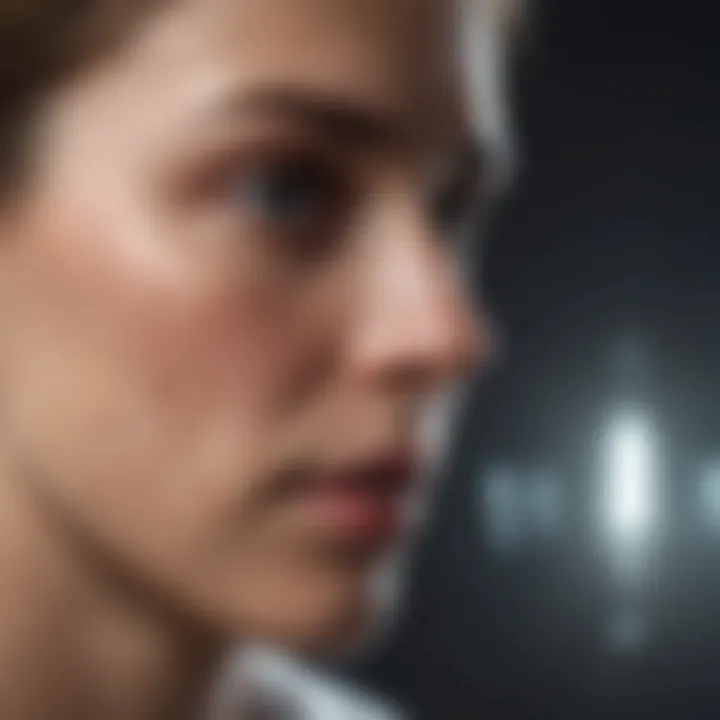
Biomedical engineers in research and development are at the forefront of medical innovation. They engage in projects that aim to create new technologies, devices, and practices that improve patient care. This could involve developing cutting-edge medical devices like pacemakers or imaging technologies that provide real-time diagnostics.
"Innovation in biomedical engineering goes beyond product development; it involves improving existing solutions to enhance patient safety and care efficiency."
In research settings, these professionals often collaborate with scientists and medical experts, ensuring that developments address real-world medical issues. Their work can have far-reaching implications, leading to breakthroughs that could save lives or improve healthcare delivery processes. This aspect of their role emphasizes the importance of teamwork and interdisciplinary approaches in advancing healthcare.
Clinical Engineering
Clinical engineering is another pivotal area where biomedical engineers contribute significantly. They work closely in hospitals and healthcare facilities to manage and maintain medical devices and systems. A clinical engineer ensures that devices are functioning properly and comply with safety regulations.
Their responsibilities also include:
- Evaluating new technologies for hospital use.
- Training staff on operating complex equipment.
- Addressing technical issues that arise during medical procedures.
The expertise of clinical engineers helps in creating a safer and more efficient environment for patient treatment. Their presence ensures that medical teams can rely on the functionality of tools and systems, critically impacting patient outcomes.
Regulatory Affairs
Biomedical engineers also engage in regulatory affairs, where they navigate the complex landscape of healthcare regulations. In this role, they ensure that medical devices and technologies comply with governmental standards before they reach the market. This aspect of their job is crucial because it protects patient safety and guarantees that new products are effective.
Key responsibilities in regulatory affairs include:
- Preparing submissions to regulatory bodies such as the FDA.
- Monitoring compliance with regulations throughout the product lifecycle.
- Interpreting regulatory requirements to guide development teams.
Their understanding of regulatory frameworks not only facilitates smooth market entry of new innovations but also enhances the trust of healthcare professionals and patients in using these technologies.
Innovations in Biomedical Engineering
Biomedical engineering is a rapidly advancing discipline, characterized by its ability to create and implement innovative technologies that improve healthcare delivery and patient outcomes. These innovations are essential because they integrate science, engineering, and medicine to address complex health challenges. Advancements in this field can lead to enhanced diagnostics, more effective treatments, and better patient monitoring systems. As we explore the innovations occurring in biomedical engineering, it becomes clear that they play a critical role in shaping the future of healthcare.
Wearable Technology
Wearable technology represents a significant innovation in biomedical engineering. Devices like smartwatches and fitness trackers allow users to monitor their health in real-time. These devices can track various metrics such as heart rate, sleep patterns, and physical activity levels.
The benefits of wearable tech are manifold:
- Continuous Monitoring: Users can monitor their health continuously, which is especially useful for managing chronic conditions.
- Data Collection: Wearable devices collect large amounts of data that can be analyzed for trends, helping doctors make informed decisions.
- Patient Engagement: They promote active participation in health management by empowering individuals to take charge of their wellness.
Despite these advantages, there are considerations. Data privacy remains a concern, as sensitive health information could be exposed. Additionally, accuracy can vary between devices, which could lead to misinterpretations of health data.
Robotics in Surgery
Robotics in surgery marks another domain where biomedical engineering has made considerable strides. Surgical robots, like the da Vinci Surgical System, enhance the surgeon's capabilities, offering precision and improved visualization of the surgical field.
Here are key aspects to consider about robotic surgery:
- Minimally Invasive Procedures: Robots allow for smaller incisions, leading to decreased recovery times and lower risk of infection.
- Enhanced Precision: Surgeons have better control during complex procedures, reducing the likelihood of complications.
- Training Simulators: Robotic systems can also be used for training purposes, helping new surgeons develop their skills in a controlled environment.
However, the cost of robotic systems can be a significant barrier to their adoption in some healthcare settings. This raises questions about resource allocation within healthcare infrastructure.
Artificial Intelligence Applications
Artificial Intelligence (AI) is revolutionizing biomedical engineering, providing tools for better decision-making and personalizing patient care. AI algorithms analyze vast datasets to identify patterns that can lead to earlier diagnosis and more effective treatment plans.
Key elements of AI in this field include:
- Diagnostic Imaging: AI technologies can assist in interpreting medical images, increasing accuracy in identifying conditions such as tumors.
- Predictive Analytics: By analyzing patient data, AI can predict potential health issues before they become critical.
- Personalized Medicine: Tailoring treatments based on individual patient data can lead to more effective healthcare outcomes.
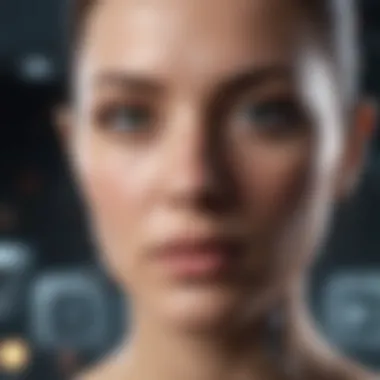
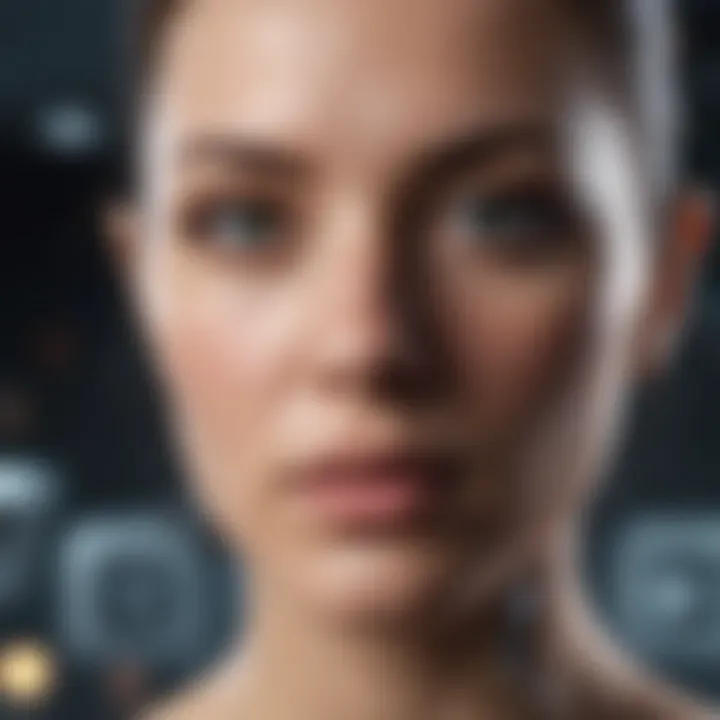
Nevertheless, ethical considerations arise regarding data use and algorithm biases. Ensuring AI systems are transparent and equitable is crucial moving forward.
"Innovations in biomedical engineering not only enhance health outcomes but also ensure a better quality of life for patients worldwide."
Ethical Considerations in Biomedical Engineering
Ethics is a cornerstone of biomedical engineering, shaping the practices and responsibilities of professionals in the field. As technology advances, the intersection of healthcare and engineering raises essential ethical questions. These questions concern the welfare of patients, the integrity of data, and the implications of emerging technologies. Recognizing and addressing these considerations is vital for ensuring that biomedical engineering contributes positively to society.
Patient Privacy
Patient privacy is a fundamental ethical principle in healthcare and biomedical engineering. As medical devices and systems increasingly collect and store personal health information, the risk of data breaches becomes significant. Biomedical engineers must ensure that systems are designed with privacy in mind. This includes implementing robust security measures to protect sensitive data against unauthorized access.
Moreover, the development of wearable technologies further complicates privacy issues. Devices such as fitness trackers and health monitoring tools continuously gather data, raising questions about who controls this information. Healthcare providers and engineers need to engage in dialogue about the most ethical ways to handle patient data while maintaining confidentiality and compliance with laws like HIPAA in the United States.
"The challenge lies in navigating patient privacy amidst rapid technological advancements."
Consent and Autonomy
Consent is another crucial aspect of ethics in biomedical engineering. Patients must have the right to make informed decisions regarding their medical care and treatment options. This autonomy is vital, especially in clinical trials involving new biomedical technologies. Researchers and engineers need to ensure that participants understand the implications of their involvement and the potential risks and benefits.
Informed consent goes beyond just signing a form; it involves clear communication about the technology being used and the data that will be collected. Biomedical engineers play a key role in developing educational materials that clarify complex information while respecting the autonomy of patients. It is essential to affirm that patients can withdraw their consent at any time, reinforcing their ongoing control over personal health decisions.
By addressing ethical considerations like patient privacy and informed consent, engineers can foster trust in biomedical innovations. This trust is essential for the successful adoption of new technologies and the overall improvement of patient outcomes. The social responsibility of biomedical engineers is to ensure their work enhances human dignity, respects the autonomy of individuals, and safeguards privacy.
Future Directions in Biomedical Engineering
Future directions in biomedical engineering are crucial as they pave the way for innovations that can dramatically enhance healthcare delivery and patient outcomes. The ongoing evolution of this field relies on the integration of multidisciplinary knowledge and advancements in technology. As healthcare systems face ever-changing challenges, biomedical engineering presents solutions that are both practical and insightful. This section delves into emerging trends and their impact on global health, providing a thorough understanding of the future landscape of biomedical engineering.
Emerging Trends
In recent years, several emerging trends have gained traction in biomedical engineering. Some of the most significant include:
- Personalized Medicine: Advances in genomics and biotechnology enable tailored treatments based on individual patient profiles. This approach optimizes care and enhances treatment efficacy.
- Telehealth Solutions: With the increasing reliance on digital platforms, telehealth expands access to healthcare. Biomedical engineers are developing innovative tools that facilitate remote monitoring and consultations, making healthcare more efficient.
- Smart Medical Devices: The rise of IoT devices in healthcare has led to the creation of connected devices that monitor health metrics in real-time. These smart devices provide valuable data for both patients and healthcare providers, enhancing management and decision-making.
- 3D Bioprinting: This technology allows for the fabrication of tissues and organs using biodegradable materials. Progress in this area could revolutionize transplantation and regenerative medicine.
- Wearable Technology: Wearables, such as fitness trackers and smartwatches, are increasingly incorporating health monitoring features. Biomedical engineers are refining these technologies to improve accuracy and usability, ultimately empowering users to take charge of their health.
Collectively, these trends signify a shift towards more efficient and patient-centered care. The role of biomedical engineers in these developments is vital. Their expertise enables the design and integration of these technologies into real-world applications.
Impact on Global Health
The future directions in biomedical engineering hold profound implications for global health. As technologies evolve, so will their ability to address pressing health concerns. Key aspects of this impact include:
- Improved Access to Care: Innovations in telemedicine and mobile health platforms can reach underserved populations, bridging gaps in access to healthcare services. This is particularly important for remote areas with limited medical facilities.
- Enhanced Disease Management: By utilizing wearable devices and smart medical technologies, patients can monitor chronic diseases more effectively. Real-time data allows for timely interventions, reducing complications and hospital visits.
- Cost-Effectiveness: Technological advancements can lead to more efficient healthcare processes, ultimately reducing costs for both providers and patients. For instance, remote monitoring reduces the need for in-person visits, alleviating the burden on healthcare systems.
- Global Collaboration: Biomedical engineering is not confined to national borders. Collaborative initiatives can stimulate the exchange of knowledge and resources, promoting innovations that serve populations worldwide.
"Innovative biomedical technologies are not just advancements; they are vital pathways to improve global health and equality in healthcare access."
In summary, the future of biomedical engineering is promising. Emerging trends will likely catalyze significant improvements in patient care. As we navigate these developments, the focus should remain on leveraging technology to enhance global health outcomes. This will require collaboration among engineers, healthcare professionals, and researchers to ensure that solutions are both effective and equitable.
End
The conclusion serves as a critical component in synthesizing the vast information presented throughout the article. It is the final opportunity to reinforce the significance of biomedical engineering within the context of modern healthcare and technology. As the field continues to evolve, understanding its implications is crucial for various stakeholders, including students, health professionals, and policymakers.
Summary of Key Points
To summarize, the article explored several core elements of biomedical engineering:
- Definition: Biomedical engineering combines engineering principles with medical and biological sciences to enhance healthcare.
- Key Components: Areas such as biomedical devices, biomaterials, tissue engineering, and medical imaging are vital to this discipline.
- Educational Pathways: Various academic programs and certifications are crucial for preparing professionals in this field.
- Core Competencies: Technical, analytical, and communication skills are essential for success.
- Roles and Innovations: Biomedical engineers engage in research, development, and implementation of cutting-edge technologies like wearable devices and robotics in surgery.
- Ethical Considerations: Issues such as patient privacy and autonomy must always be considered.
- Future Directions: The continual emergence of trends poses both opportunities and challenges in global health contexts.
This comprehensive overview not only highlights the critical areas of focus but also sets a framework for readers to understand the multifaceted nature of biomedical engineering and its relevance in today’s world.
Call for Ongoing Research
Looking forward, it is imperative to call for ongoing research within the field of biomedical engineering. The rapid pace of technological advancements necessitates continuous exploration and innovation. As biomedical engineers tackle existing problems and anticipate future healthcare challenges, ongoing research will lead to breakthroughs that can dramatically improve patient outcomes.
Additionally, multidisciplinary collaboration will enhance the effectiveness of biomedical engineering solutions. By integrating insights from various fields such as computer science, biology, and materials science, new methods and technologies can emerge.
"Innovation in biomedical engineering does not happen in isolation; it thrives on collaboration and shared knowledge."