Understanding Cell Culture Growth Dynamics
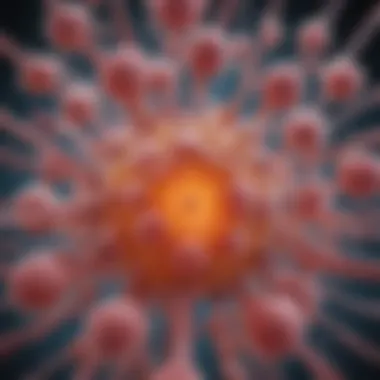
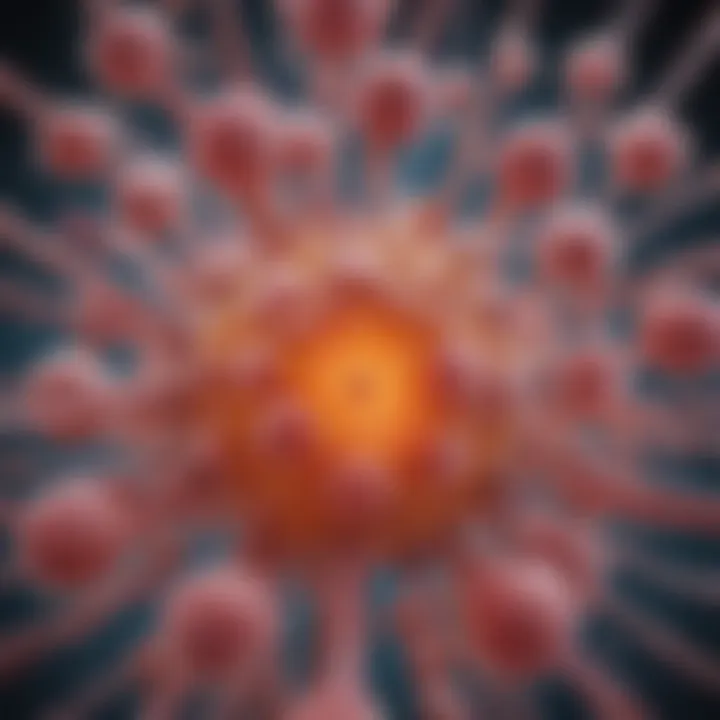
Intro
The world of cell culture is an intricate tapestry where science meets technology, evolving rapidly as we strive to understand and manipulate biological systems for myriad purposes. Cell culture growth is not only a cornerstone for research in areas like drug development and regenerative medicine but also a fascinating field in its own right. It is essential to grasp the dynamics behind cell cultures, as this knowledge informs best practices and innovations that can lead to significant breakthroughs.
At its core, cell culture involves maintaining and growing cells under controlled conditions, distinct from their natural environment. With the increasing demand for alternative methods to test drugs and develop therapies, cell culture has emerged as a critical player in biological research. What lies beneath the surface of this seemingly straightforward practice is a complexity filled with cellular interactions, metabolism, and adaptation to various stimuli. This article embarks on unearthing these layers, elucidating key aspects while appreciating the implications of advancements in this domain.
Research Highlights
Overview of Key Findings
Cell culture, often considered the bread and butter of the biological sciences, reveals a plethora of insights into cellular behavior that traditional methods fail to uncover. A critical review of current methodologies exposes gaps in techniques that researchers strive to address. Furthermore, technological advances, such as automating systems for monitoring growth patterns and nutrient consumption, pave the way for high-throughput screenings that benefit drug discovery processes.
Significance of the Research
Understanding cell dynamics is not merely an academic exercise. It bears real-world implications as we grapple with pressing challenges in healthcare. From screening drugs that can save lives to developing personalized therapies that consider an individual’s unique biology, the significance of cell culture growth cannot be overstated. To paraphrase a well-known saying, one might say that the future of medicine lies in the well-cultured cells in the laboratory.
"Cell culture is the lens through which we can view the minutiae of cellular life, each experiment revealing another piece of the puzzle in understanding life itself."
Original Research Articles
Summary of the Article
Research articles examining innovative methodologies in cell culture growth have surged in number. Recent papers focus on the need for precision in how we define culture conditions to achieve optimal outcomes. These studies often emphasize integrating biological and environmental factors that drive growth and maturation of cells.
Author Contributions
The researchers contributing to these articles come from diverse backgrounds, blending expertise in cellular biology, bioengineering, and pharmaceutical sciences. Collaboration across disciplinary lines fosters creativity that leads to novel insights and informs future research directions. As cell culture methodologies improve, so too will our understanding of complex diseases and therapeutic interventions.
Prologue to Cell Culture Growth
Understanding the dynamics of cell culture growth is essential for anyone navigating the realms of biological research and biotechnology. The very foundation of modern life sciences rests on the methodologies and techniques involved in growing cells outside their natural environment. In today’s context, with innovations mushrooming every day, having a grasp of how and why these cultures thrive is not merely academic; it's vital for groundbreaking discoveries that can shape health care and treatment strategies.
Definition and Importance
Cell culture refers to the process of cultivating cells in controlled environments, allowing researchers to study their growth, behavior, and response to various stimuli. This practice has paved the way for significant advances in medical research, drug development, and regenerative medicine. The ability to replicate cellular environments enables scientists to conduct experiments that would be impossible in living organisms.
The importance of cell culture cannot be overstated:
- It provides a platform for drug testing, significantly reducing the need for initially using live animals.
- Cultured cells can mimic native tissue, offering insights into disease mechanisms and facilitating the exploration of therapeutic applications.
- It supports vaccine development and genetic engineering efforts, crucial for addressing public health challenges.
In essence, cell culture is not just a method; it is a bridge connecting theoretical research with practical applications, illustrating the relevance of biological insights in real-world scenarios.
Historical Context
The journey of cell culture is both rich and revealing, tracing back to the late 19th century and early 20th century when early researchers embarked on the challenge of isolating cells. The significance of these early experiments laid the groundwork for what would evolve into a core component of biological sciences.
- In 1885, Wilhelm Roux conducted one of the first known experiments where he cultured embryonic chick spinal cord tissue, ushering in a new era of cellular research.
- Fast forward to the 1950s, and you have George and Margaret Gey who famously developed the HeLa cell line from cervical cancer cells taken from Henrietta Lacks. These immortal cells became a cornerstone of modern medical research, endlessly reproducing and providing invaluable data across various scientific fields.
The historical milestones highlight not just the evolution of techniques but also the changing perspectives on ethical considerations and practices in cell culture. As cellular biology continues to advance, understanding its historical context offers vital lessons on responsibility and innovation moving forward.
Types of Cell Culture
Understanding the types of cell culture is crucial as it lays the groundwork for various applications in research and industry. Each type has its own strengths and weaknesses, and the choice can significantly affect the outcomes of experiments. Here, we'll explore three primary categories: primary cell cultures, continuous cell lines, and stem cell cultures, detailing the implications of each type in cellular research.
Primary Cell Cultures
Primary cell cultures are derived directly from tissues, representing a close approximation to in vivo conditions. These cells maintain many of the physiological characteristics of their tissue of origin, which can be essential when studying specific cellular behaviors or drug responses. However, they come with their own set of challenges, notably their limited lifespan. Cells can grow for only a finite number of divisions before they enter senescence. This makes it tricky to scale up experiments over time.
When using primary cell cultures, it's important to consider the following points:
- Authenticity: These cultures tend to mirror the conditions found in the body more accurately than continuous cell lines.
- Variability: Being derived from living organisms means they can exhibit considerable biological variability, making reproducibility a concern.
- Specialized Needs: Primary cultures often require specific growth conditions, including precise media composition and the right environmental parameters.
In summary, while primary cell cultures provide vital insights, their limitations urge researchers to carefully consider how they fit into their specific experimental designs.
Continuous Cell Lines
In stark contrast, continuous cell lines offer an advantage in terms of availability and consistency. These cells can proliferate indefinitely, allowing for long-term studies and experiments. Continuous cell lines are often modified to be more resilient and can withstand various stresses, which is beneficial for many research applications. One well-known example is the HeLa cell line, derived from cervical cancer cells, which has been pivotal in numerous biomedical discoveries.
The key aspects to consider with continuous cell lines include:
- Genetic Alterations: Many continuous lines have undergone transformation and may not accurately replicate the normal cellular environment.
- Ease of Use: Their stability and abundance make them easier to handle, especially in large-scale applications, such as drug testing.
- Reproducibility: Due to their uniform characteristics, results gathered from continuous cell lines can often be more reliably replicated compared to primary cultures.
Nevertheless, researchers should be aware of potential limitations. Continuous cell lines might not fully encapsulate the original cellular context, leading to results that could be misleading.
Stem Cell Cultures
Stem cell cultures embody a unique category, given their potential to develop into various cell types. They can be classified into embryonic stem cells and adult stem cells, both of which hold substantial promise for regenerative medicine. These cultures contribute to understanding developmental biology and offer prospects for tissue engineering, creating living tissues and organs in vitro.
Important considerations regarding stem cell cultures:
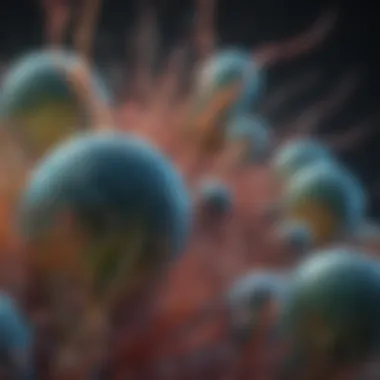
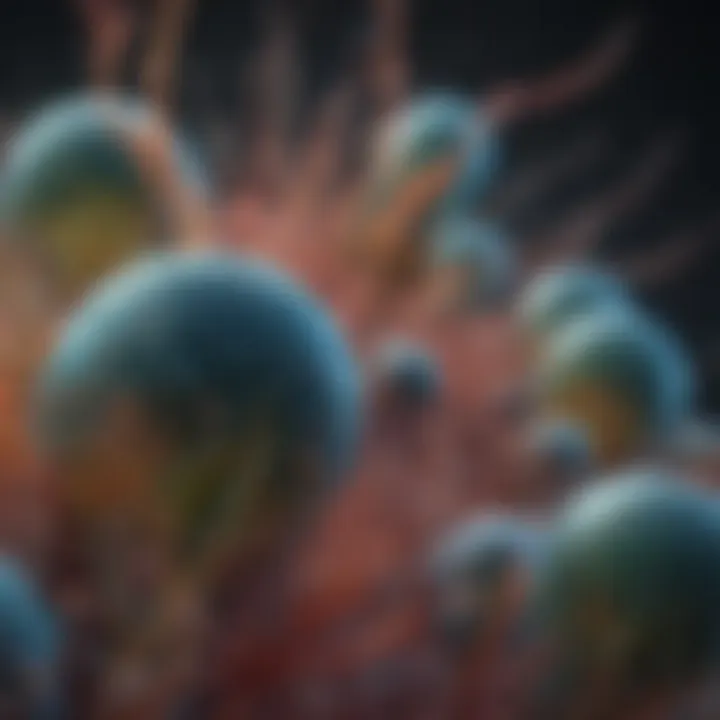
- Potency: Embryonic stem cells have the capacity to differentiate into any cell type, whereas adult stem cells are usually restricted to specific lineages.
- Ethics: Work with embryonic stem cells often invites ethical debates concerning their source and use, which researchers need to navigate thoughtfully.
- Complexity: Cultivating stem cells requires meticulous procedures to maintain their undifferentiated state or induce them to differentiate as desired, making them more complex than other types of culture.
In short, stem cell cultures stand at the forefront of numerous innovative medical advancements, but they also come with a labyrinth of ethical and technical challenges.
In essence, the types of cell cultures form the backbone of cellular research, each providing vital, yet distinct, avenues for exploration in the life sciences. Their selection plays a pivotal role in the accuracy and applicability of research findings.
"Choosing the right type of cell culture is like picking the right tool from a toolbox – it can make or break your project."
As researchers move forward in their work, a comprehensive understanding of these differences will enhance both the design and interpretation of their experiments.
Fundamental Techniques in Cell Culture
Fundamental techniques in cell culture are the backbone of any successful research endeavor involving live cells. They not only dictate the quality of the cell cultures produced but also the reliability of the data derived from experimental results. Mastering these techniques is essential for ensuring that the cell cultures thrive, proliferate, and produce accurate outcomes. A deep understanding of the processes involved—ranging from maintaining sterility to preparing growth media—is paramount. Each method contributes significantly to the overall success and reproducibility of cell culture experiments.
Sterile Technique
Adopting sterile techniques is arguably one of the most critical aspects of cell culture. Contamination can wreak havoc in a lab, leading to erroneous conclusions and wasted resources. The core of sterility hinges on preventing microbial intrusion from the outset. This can be achieved through several methods, including:
- Use of sterilized tools and equipment: Prior to any experiment, ensure that all tools are cleaned and sterilized. An autoclave is effective in achieving this.
- Personal protective equipment (PPE): Lab coats, gloves, and masks should always be worn to minimize exposure to outside contaminants.
- Laminar flow hoods: Utilizing these enclosed spaces provides an uncontaminated environment for handling cell cultures.
These practices reduce the risk of bacterial or fungal strikes, ensuring that the cell cultures are monitored in a controlled environment.
Media Preparation
Media preparation is yet another fundamental skill in cell culture. The growth medium not only provides nutrients but also a supportive environment that mimics the cells' natural habitat. Here’s what you should keep in mind when preparing media:
- Selection of appropriate media: Choose media based on the specific cell type. For example, Dulbecco's Modified Eagle Medium (DMEM) is suitable for many adherent cell lines, while RPMI 1640 works well for suspension cultures.
- Supplementation: Adding specific growth factors or serum, like fetal bovine serum (FBS), can enhance cell growth. However, the concentrations must be optimal; too little may hinder growth, while too much can negatively affect cellular behavior.
- pH and Osmolarity: Always check and adjust pH to around 7.2-7.4. Additionally, maintaining osmolarity is vital since cells are sensitive to changes in solute concentrations.
Proper media prepares the stage for cells to grow and divide effectively, making media preparation an art as much as it is a science.
"Regardless of your cell culture goals, failing to prepare the right media can cripple even the most promising studies."
Subculturing Methods
Subculturing is the process of transferring cells from one culture vessel to another, and it typically becomes necessary when cells reach confluence, that is when they cover the entire surface of the culture dish. This step is crucial for sustaining cell health and avoiding over-confluence, which can complicate cellular assays and experiments.
Key methods include:
- Trypsinization: Using trypsin helps detach adherent cells from the surface of the culture vessel. It's important to monitor the timing; prolonged exposure can damage the cells.
- Mechanical disruption: For certain cell types, gentle pipetting can help dislodge cells without the use of enzymes.
- Counting and diluting cells: Once harvested, it’s essential to count cells using a hemocytometer. Knowing the exact number allows for efficient subculturing and maintenance of optimal density.
Maintaining careful subculturing practices is essential not only to clamp down on contamination but also to uphold the genetic integrity of the cell lines being studied.
Cell Growth Factors
Cell growth factors play a pivotal role in the world of cell culture growth, acting as the lifeblood that fuels the proliferation of cells in a controlled environment. The significance of these factors cannot be overstated, as they directly influence the health, survival, and vital outcomes of cultured cells. Understanding the interplay between nutritional needs and growth factor supplementation is essential for anyone engaged in research or applications that rely on cell cultures. This section digs into the nutritional requirements and the importance of growth factor supplementation, laying the groundwork for optimizing cultures and facilitating vital research.
Nutritional Requirements
In the realm of cell culture, nutrition isn’t just a nice-to-have; it’s downright essential. Cells are like little factories, mercilessly consuming nutrients to thrive. The primary nutritional components can be broken down into several critical elements:
- Amino Acids: These organic compounds serve as the building blocks of proteins, which are crucial for nearly every cell function. Without adequate amino acids, cells simply can’t sustain themselves or replicate.
- Vitamins: Though needed in trace amounts, these organic substances are vital for numerous metabolic processes. They act as cofactors, facilitating important biochemical reactions.
- Minerals: Elements like sodium, potassium, and calcium contribute to cellular structure and function, influencing everything from osmoregulation to enzyme activity.
- Glucose: The primary energy source for many cells, glucose metabolism fuels cellular activities, supporting growth and division.
Having a growth medium that caters to these nutritional needs can make or break a cell culture experiment. Improperly balanced media can lead to subpar growth or, worse, cell death. It’s therefore paramount for researchers to select the right media formulations that provide these essential nutrients in a well-considered ratio.
Growth Factor Supplementation
While fundamental nutrients lay the groundwork, growth factors are the secret sauce that can maximize the potential of cultured cells. These proteins, often derived from natural sources like serum, provide critical signals for cell division and differentiation. Here’s why and how growth factor supplementation matters:
- Enhanced Proliferation: Growth factors can significantly boost cell proliferation rates. For instance, using epidermal growth factor (EGF) can push skin cells to replicate much faster than they would under standard conditions.
- Cell Survival: Certain growth factors help cells resist apoptosis or programmed cell death. This is particularly useful in cultures that require extended growth periods or those derived from sensitive cell types.
- Differentiation Potential: Besides mere growth, specific factors can guide cells to mature into specialized types. For example, granulocyte-colony stimulating factor (G-CSF) encourages the differentiation of stem cells into neutrophils, critical for immune response studies.
However, it’s not all about loading up on growth factors. There are considerations to keep in mind:
- Concentration Levels: Too much can lead to an overstimulation of cells, possibly resulting in uncontrollable growth or abnormal cellular behavior.
- Source Variation: Different sources of growth factors can exhibit varying potencies or activities, demanding careful calibration and testing before widespread use.
- Cost: Often, high-quality or recombinant growth factors carry a steep price, so budgeting plays a role in experimental design and selection.
"Understanding the delicate relationship between nutrition and growth factors is key to mastering cell culture growth. It's about striking that balance to optimize performance and outcomes."
In summation, navigating the intricacies of nutritional requirements and growth factor supplementation equips researchers with the tools needed to achieve robust cell growth and reliable experimental results. This knowledge lays the foundation for driving forward innovations in research and therapeutic applications.
Measurement of Cell Growth
Understanding how to measure cell growth is not just a technical footnote in the study of cell culture; it's the cornerstone of experimental reliability and reproducibility. With accurate measurement, researchers can glean insights into cellular behaviors, response to treatments, and overall health of cultures. This section will unpack the nuances of measuring cell growth, focusing on various methodologies and their relevance.
Proliferation Assays
Proliferation assays are essential tools for quantifying the rate at which cells divide. These assays typically measure aspects like DNA synthesis, which indicates cellular replication. One commonly used method is the MTT assay, where yellow tetrazolium salt is reduced by living cells to form purple formazan crystals. The intensity of the purple color reflects the number of viable cells and their metabolic activity.
Another good method is the BrdU incorporation assay, which tracks the incorporation of 5-bromo-2-deoxyuridine into newly synthesized DNA during cellular division. This approach is particularly valuable when comparing growth rates of various cell types under different conditions. In this way, proliferation assays not only offer a snapshot of current growth but also allow for comparisons across experimental setups, offering deeper insights into the dynamics at play.
Cell Counting Methods
Cell counting methods serve as the backbone for understanding population density in cultures. The traditional approach involves using a hemocytometer, which allows for manual counting under a microscope. Here, researchers count the number of cells in a defined volume, providing a direct measure of cell density. This is effective but can be labor-intensive and prone to human error.
Alternatively, more advanced techniques—such as automated cell counters—can significantly streamline this process. These devices use image analysis and sophisticated algorithms to count cells and can distinguish between live and dead cells. Moreover, fluorescent dyes, like Trypan Blue, can be utilized to improve accuracy in distinguishing viable cells from non-viable ones.
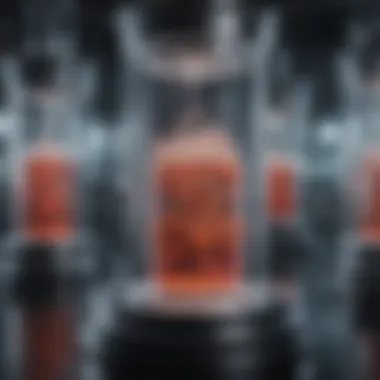
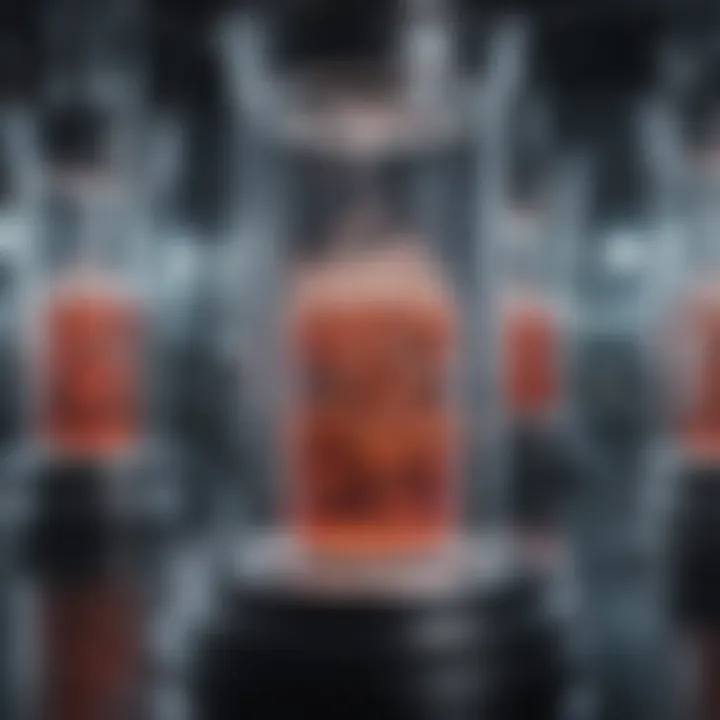
Accurate cell counting is crucial. If you miss it, your whole experiment might as well be a roll of the dice.
Such methods not only save time but also provide consistent results, an essential aspect for reproducibility in experiments. Different methods might be appropriate for various contexts, but the end goal remains the same: to deliver precise and meaningful data on cell populations.
Cell Viability Tests
Cell viability tests gauge the health of cell cultures, which is paramount in understanding whether cells are alive and functional following treatments or environmental changes. The MTT assay mentioned earlier also serves in this capacity, reflecting cell viability based on metabolic activity. In this sense, it's a dual-purpose assay—an excellent example of how techniques can overlap.
Also critical is the Lactic Dehydrogenase (LDH) assay, which measures cellular enzyme release into the surrounding media, indicating membrane integrity and cellular damage. When the plasma membrane is compromised, LDH leaks out, providing a direct measurement for cell death.
Another straightforward technique is using Annexin V staining, which can differentiate between apoptotic (early stage of cell death) and necrotic (late stage of cell death) cells. By employing a combination of tests, researchers gain a more nuanced understanding of cell health, vital not only in biological research but also in drug development and toxicity studies.
The significance of measuring cell growth cannot be overstated; it's the driving force that informs decisions and shapes findings in the intricate world of cell culture. With the right methodologies in hand, researchers can confidently steer their exploratory vessels through the complexities of biological inquiries.
Challenges in Cell Culture Growth
Understanding the challenges in cell culture growth is crucial. These hurdles can impact research outcomes and the progressive adoption of cell culture technologies in various fields, including biotechnology and pharmaceuticals. Identifying and addressing these challenges can lead to more efficient practices and elevated scientific discovery, rendering cell culture more reliable and scalable.
Contamination Issues
Contamination is one of the chief concerns faced in cell culture laboratories. It can arise from a blend of sources including environmental factors, equipment, and even the handling by laboratory personnel. Once contaminated, cultures can suffer significant setbacks; growth may stagnate or become entirely compromised.
- Bacterial Contamination: This is often the most common issue. Bacteria can proliferate at alarming rates, quickly outpacing the desired cells. Thus, they can disrupt experiments right out of the gate, introducing variability and rendering results suspect.
- Fungal Contamination: The presence of fungi can also wreak havoc on cell cultures. These organisms can invade cultures, creating an unmanageable mess and leading to totally unusable samples.
- Mycoplasma Contamination: A sneaky player in the world of contamination is mycoplasma. These bacteria are much smaller than typical bacteria, often slipping through standard filtration measures. They can alter cell behavior subtly, making it a daunting task to identify their presence.
To mitigate these risks, rigorous sterile techniques are essential. Regular monitoring of cell cultures and environmental conditions will also go a long way in reducing contamination incidents.
"An ounce of prevention is worth a pound of cure." This adage rings particularly true in cell culture where proactive measures can skirt serious setbacks.
Variability in Growth Rates
Variability in growth rates is another issue that can frustrate researchers. Not all cell lines behave consistently, and many factors can influence growth, leading to unpredictable results.
- Genetic Background: Different cell lines possess unique genetic identities. Even slight genetic variances can lead to distinct growth patterns, affecting replicability across experiments.
- Culture Conditions: The environment—temperature, CO2 levels, and nutrient media—can greatly affect growth rates. For example, a minor deviation in pH can alter cellular metabolism, impacting how quickly cells proliferate.
- Subculturing Practices: If cells are not split in a timely manner, overcrowding might occur. This overcrowding can lead to competition for resources, thus slowing down growth rates even further.
In attempting to streamline the growth of cells, researchers may establish standard operating procedures to minimize variability. It would also be wise to conduct preliminary experiments to better understand how specific conditions influence growth. Through careful analysis, researchers can strive to create more consistent and reproducible culture conditions, thereby improving the overall reliability of cell culture studies.
Innovations in Cell Culture Technology
In today’s fast-paced biotechnological landscape, the innovations in cell culture technology serve as a backbone for scientific exploration and advancement. The emergence of novel techniques has markedly improved the efficiency and scalability of cell culture processes, allowing researchers to achieve more with less. This section highlights two pivotal advancements: bioreactors designed for large-scale culturing and automated cell culture systems. Both innovations not only enhance production efficiency but also ensure reproducibility, which is critical in research and development.
Bioreactors for Large Scale Culturing
Bioreactors represent a significant leap in the ability to cultivate cells at scale. Traditionally, small laboratory flasks dominated the scene, but as demand for biological products grows—think vaccines, monoclonal antibodies, and cell-based therapies—the limitations of these methods become apparent.
Bioreactors, on the other hand, offer a controlled environment tailored for cell growth. These systems can regulate crucial parameters such as temperature, pH, and oxygen levels, fostering optimal conditions for cell proliferation. Here are some distinct benefits of utilizing bioreactors:
- Scalability: The transition from a few milliliters to several liters is seamless, providing ample material for trial therapies or vaccine doses.
- Consistency: Automated control results in uniform cell responses, significantly reducing batch variability and improving the predictability of experimental outcomes.
- Cost-Effectiveness: While initial investments can be steep, the enhanced yield can drastically lower costs per unit, a consideration that cannot be ignored by pharmaceutical companies.
However, careful consideration must be given to the choice of bioreactor and its design. Not all cells flourish in the same conditions, so optimizing the bioreactor to match the specific needs of the cell line is vital. The adaptability of bioreactors allows them to be customized, from stirring methods to gas exchange techniques, depending on the cells being cultured.
Automated Cell Culture Systems
Automation is transforming cell culture into a more streamlined process, where human error can become a thing of the past. Automated systems are revolutionizing the field by streamlining workflows, enhancing consistency, and ensuring high-throughput capabilities. With these systems, experiments can run smoother and more efficiently.
Benefits of automated systems include:
- Time Efficiency: These systems handle repetitive tasks—media changes, monitoring environmental conditions—allowing researchers to focus their efforts on experimental design and analysis instead of mundane chores.
- Increased Throughput: The ability to culture hundreds, even thousands, of cell lines simultaneously opens new avenues for research, particularly in drug screening and genetic studies.
- Data Integration: Modern automated systems can be integrated with data analysis software, providing real-time feedback and insights, making it easier to track cell health and growth patterns.
Despite the advantages, a bit of caution is necessary. Fully automated systems can sometimes lead to an understanding gap; researchers may lose touch with the hands-on skills that are crucial for troubleshooting. Ongoing training and familiarity with both the technology and biological principles remain key to harnessing these innovations effectively.
In sum, the impact of innovations in bioreactors and automated systems significantly enhances the efficiency and effectiveness of cell culture operations, paving the way for breakthroughs in various fields.
These innovations form only part of the broader narrative of cell culture technology's evolution. As we continue our journey, it's evident that these advancements do not only serve researchers but also ripple outwards, impacting industries like pharmaceuticals and regenerative medicine.
Applications of Cell Culture in Research
Cell culture serves as a backbone for a variety of research applications, providing invaluable insights and advancements across multiple scientific disciplines. The utilization of in vitro cultured cells has become a routine practice in labs around the globe, underpinning developments in pharmaceutical drug testing, disease modeling, and genetic exploration. The significance of these applications cannot be overstated, as they facilitate controlled experiments that often align more closely with human biology than traditional animal models. Let’s delve into three major areas where cell culture has made a tremendous impact.
Drug Development Processes
The journey from a compound in a lab to a market-ready medication is often fraught with trials and errors. Cell culture is pivotal in drug development, allowing researchers to screen various compounds and assess their efficacy and safety before advancing to preclinical and clinical trials.
- High-throughput Screening: Cell culture systems can be designed to conduct high-throughput screenings of chemical libraries. This process accelerates the identification of potential drug candidates, cutting down the time needed to bring new therapies to patients.
- Mechanistic Studies: Researchers can investigate how a drug interacts with cellular pathways and mechanisms. This understanding helps refine therapeutic approaches and mitigate side effects.
- Personalized Medicine: With advancements in personalized medicine, specific patient cell lines are cultured to evaluate drug responses. This paves the way for tailored treatments that optimize therapeutic outcomes.
As the scientist and entrepreneur Yvonne C. J. Hwang states, "The transition from bench to bedside often hinges on the outcomes derived from cell cultures."
Cancer Research
Cell culture techniques are an integral part of cancer research, shedding light on complex mechanisms of tumorigenesis and enabling the development of targeted therapies. Cancer cells are often grown from patient biopsies, helping researchers understand individual tumor characteristics.
- Studying Cancer Biology: Using cell culture, scientists can observe cancer cell behavior in a controlled environment. This includes understanding mutations, signaling pathways, and how different cells interact within the tumor microenvironment.
- Drug Testing: Drug efficacy can be evaluated against specific cancer cell lines. Notably, this allows for the exploration of resistance mechanisms, leading to the development of more effective treatments.
- Biomarkers Discovery: Identifying biomarkers in cultured cancer cells is crucial for early detection and monitoring treatment responses. These markers can inform prognostic evaluations.

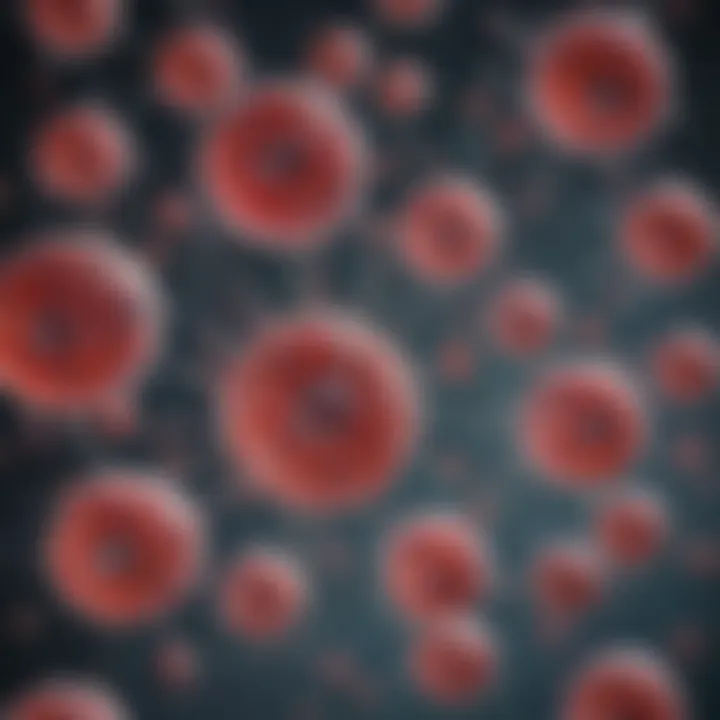
Genetic Studies
Cell culture is also significantly utilized in genetic studies, serving as a platform for gene editing, gene expression analysis, and the study of genetic disorders. The ability to manipulate cellular genetics in vitro allows researchers to investigate the effects of specific genes on cell behavior.
- CRISPR and Gene Editing: The advent of CRISPR technology has revolutionized genetic studies, enabling precise modifications to DNA sequences in cultured cells. This capability illuminates gene function and disease mechanisms.
- Modeling Genetic Disorders: Researchers can create specific cell models of genetic disorders, aiding in the understanding of pathophysiology and identifying potential therapeutic targets.
- Screening Genetic Variants: Cultures can be used to study the impact of various genetic variants on cellular function, contributing significantly to the field of genomics.
In summary, applications of cell culture form the foundation of modern biomedical research, driving innovations that have led to groundbreaking therapies and advancements in understanding human diseases. The ability to grow and manipulate cells in vitro not only contributes to the understanding of fundamental biological processes but also helps bridge the gap between scientific inquiry and clinical application.
Ethical Considerations in Cell Culture
As we navigate the complex landscape of cell culture research, it becomes imperative to address the ethical considerations that accompany these practices. This section dives deep into the responsibilities researchers bear in ensuring the humane treatment of biological materials, emphasizing the balancing act between scientific advancements and ethical integrity.
Animal Rights and Welfare
The use of animal-derived cells in research has been a cornerstone of many scientific breakthroughs. However, it raises a host of ethical issues surrounding animal rights and welfare. It's essential to recognize that every animal life is significant, and researchers have an obligation to treat subjects with respect and dignity.
In many countries, there are stringent regulations governing animal research. These laws are designed to minimize suffering and ensure that when animals are used, their treatment aligns with humane standards. Researchers often adhere to the 3Rs principle: Replace, Reduce, and Refine. This principle encourages scientists to:
- Replace animal models with alternative techniques, such as computational models or 3D cultures, whenever possible.
- Reduce the number of animals used in experiments by optimizing study designs and methodologies.
- Refine existing procedures to cause less distress or harm to animals.
Through this lens, adopting a proactive stance on animal welfare in cell culture—along with using established ethical guidelines—becomes incredibly significant not only for compliance but also for the enhancement of society's overall conscience regarding the scientists' role in animal welfare.
"Ethics is knowing the difference between what you have a right to do and what is right to do."
Use of Human Cells
Human cells in research open another ethical frontier, especially when considering their origin. The use of these cells often stems from donated tissues during medical treatments or from embryonic sources, leading to ethical debates surrounding consent and autonomy. Knowing the origin of these cells and respecting donor rights is pivotal in shaping research integrity.
When engaging with human-derived cells, several important principles come into play:
- Informed Consent: Researchers must ensure that donors are fully informed about how their cells will be used, what the research entails, and any potential implications. This applies to tissues collected from living donors as much as it does to those taken post-mortem.
- Privacy and Confidentiality: Protecting the identities of donors is paramount. Personal data connected to cell samples must be securely managed to maintain confidentiality.
- Ethical Review Boards: Most research involving human cells undergoes scrutiny by Institutional Review Boards. These groups assess protocols to ensure that ethical standards are upheld and that research doesn't compromise individuals’ rights or welfare.
Incorporating these values not only aligns research with ethical standards but also fosters public trust in the scientific community. It reflects the understanding that while the pursuit of knowledge is essential, it should never come at the cost of human dignity.
Future Directions in Cell Culture Research
Research in cell culture is at a pivotal juncture. As we advance in our understanding of cellular mechanisms, the future of cell culture research holds immense promise. Innovations are not just around the corner; they are here, reshaping how we cultivate and analyze cells. Analysing future directions in this field can offer significant benefits, like improved efficiency in research and better replication of biological phenomena.
Customization of Cell Culture Environments
One of the most intriguing advancements coming down the pipeline is the customization of cell culture environments. It’s like tailoring a suit to fit one perfectly, only in this case, it’s about optimizing factors such as temperature, pH, and nutrient levels for specific cell types.
Custom environments can vastly improve cell behavior and function, allowing scientists to mimic in vivo conditions more closely. For instance:
- Tailored Media Composition: Adjusting the nutrient formulations can enhance growth rates and increase yields for specific cells.
- Microenvironment Control: Utilizing biocompatible materials allows researchers to simulate the extracellular matrix, helping to guide cell differentiation and function.
- Dynamic Culture Conditions: Implementation of responsive materials can regulate microenvironment changes in response to cell activity, essentially creating a feedback loop to improve cell growth.
These customizable setups can be particularly beneficial in drug screening and tissue engineering, where precise conditions are pivotal for efficacy and success. By accommodating the specific requirements of each cell type, scientists can push the boundaries of what is possible in research and therapeutic applications.
Integration of 3D Culture Systems
Another significant trend is the growing use of 3D culture systems. Traditional two-dimensional cultures are somewhat limited, often failing to accurately represent real-life biological environments. The move to three-dimensional models offers a much more accurate simulation of tissues in the human body.
3D culture systems stand out for several reasons:
- Enhanced Cell Interaction: Cells in a 3D structure interact more like they do in tissues, influencing functions such as migration and differentiation.
- Better Drug Response Modeling: This morphology allows for a more realistic assessment of how drugs will perform in the human body. Some studies have even shown that tissue-derived 3D cultures provide better predictive power for drug treatment outcomes.
- Scalability for Bioprinting: Integration with bioprinting technologies enables the creation of complex tissue constructs for regenerative medicine and transplantation. This could pave the way for personalized organ replacement therapies.
Ending and Implications
The exploration of cell culture growth is not merely a technical endeavor; it is a cornerstone of modern biological and medical research. Understanding the dynamics involved presents numerous advantages, enhancing the efficacy of research efforts across various fields. From drug development to regenerative medicine, the implications of well-executed cell culture are far-reaching.
In this article, we discussed several essential elements underscoring the importance of cell culture growth:
- Enhanced Reproducibility: Reliable cell cultures provide consistent results. By establishing standardized protocols, researchers can reproduce experiments, ensuring validity across the board.
- Cost-effectiveness: Compared to animal models, cell cultures significantly reduce expenses while maintaining a high level of control and precision.
- Personalized Medicine: Tailoring cell culture systems to individual patient cells opens new avenues for customized treatment.
Great attention was given to various considerations related to cell culture practices. Maintaining sterile environments and avoiding contamination are crucial. A lapse in these practices can derail even the most promising studies, leading to misguided conclusions and wasted resources.
Moreover, the ethical considerations regarding the use of human and animal cells cannot be overlooked. Scientists must strike a balance between advancing biotechnological innovations and adhering to ethical standards that prioritize animal welfare and human rights.
Ultimately, the future of cell culture growth holds immense promise. As researchers continue to refine techniques and embrace innovations like 3D culture systems, the potential for breakthroughs in understanding complex biological processes grows. As this field advances, it will undoubtedly impact numerous avenues of science and medicine.
Summation of Key Points
The key takeaways from our exploration of cell culture dynamics include:
- The significance of robust methodologies and protocols to enhance reproducibility.
- The critical role of ethical considerations in this domain, especially concerning human and animal welfare.
- Innovations in technology, such as automated systems and bioreactors, further enabling large-scale culturing.
These points encapsulate the essence of what makes the field of cell culture crucial to contemporary research.
Impact on Scientific Advancements
The advances in cell culture techniques have catalyzed significant progress in various scientific disciplines. Here are several notable impacts on scientific advancements:
- Innovative Treatment Strategies: Researchers are developing new approaches to disease treatment that were once deemed unattainable. For instance, cancer therapies now often begin with drug testing on cultured cells, allowing for quicker iterations.
- Understanding Disease Mechanisms: Cultured cells serve as model systems to study diseases, enabling scientists to dissect complex pathways and identify potential therapeutic targets. This work is crucial in diseases such as Alzheimer's or diabetes.
- Biotechnology Developments: Cell culture is at the heart of biopharmaceuticals production. Companies utilize cell lines to produce vaccines, therapeutic proteins, and other biologics that are essential for patient treatment.
"The evolution of cell culture technology has transformed the landscape of biological research, making previously impractical solutions attainable."
In summary, cell culture growth not only facilitates scientific inquiry but drives advancements that have a tangible effect on healthcare and disease management. The continued investment in this area promises innovative solutions to some of life's most pressing challenges.