Exploring the Depths of DNA Mutations
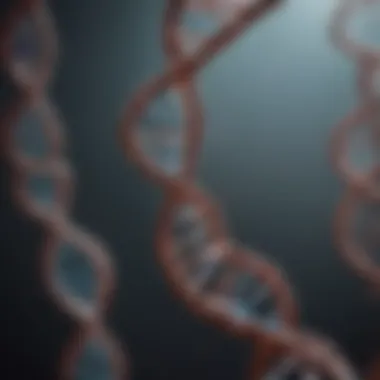
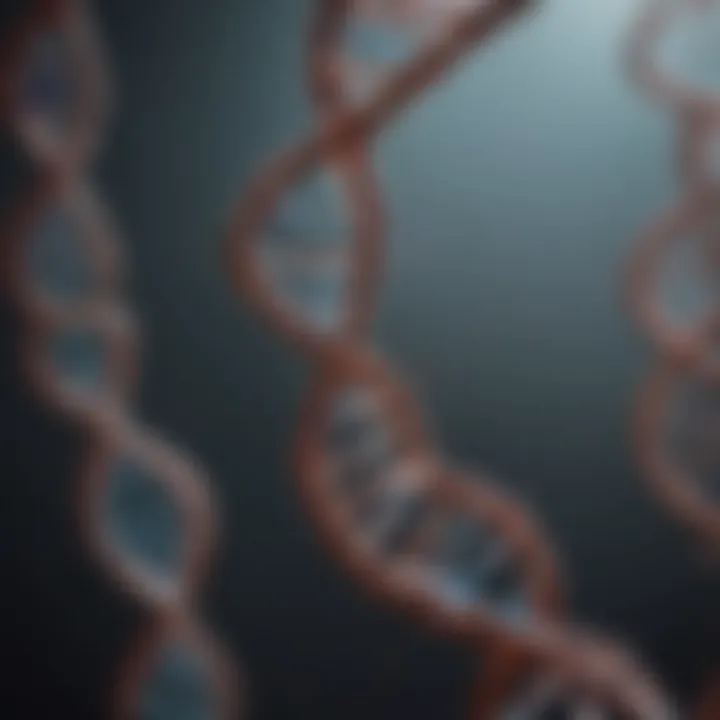
Intro
DNA mutations are essential to grasp when studying genetics and evolution. They represent alterations in the nucleotide sequences that compose an organism’s genetic code. These changes are critical. Not only do they fuel evolutionary processes, they also have profound implications for health and disease.
Types of DNA Mutations
Mutations can be categorized into various types. For instance, substitutions occur when one nucleotide is replaced by another. Insertions add extra nucleotides to a sequence, while deletions remove them. These mutations may affect just a single base pair or involve larger segments. Understanding the types aids in comprehending their effects on organisms.
Causes of DNA Mutations
The causes of DNA mutations are diverse. They can arise from external factors like radiation and chemical exposure. Additionally, internal mechanisms such as errors during DNA replication contribute to mutations. Identifying these causes helps researchers develop preventive strategies.
"Understanding the mechanisms behind DNA mutations is crucial for both evolutionary biology and medical genetics."
Impact on Health and Disease
The implications of DNA mutations extend to various genetic disorders. Some mutations are benign, while others can lead to severe health issues, including cancer. Understanding these effects is vital for developing targeted therapies and preventive measures.
DNA Repair Mechanisms
The body has evolved several DNA repair mechanisms to address mutations. These mechanisms are critical for maintaining genetic stability. Familiarity with these processes provides insight into how organisms manage genetic integrity despite constant environmental pressures.
Research Advancements
Recent advancements in research focus on understanding and correcting DNA mutations. Techniques like CRISPR-Cas9 are paving the way for potential therapies that could reverse harmful mutations. Staying updated on such research offers hope for future medical advancements.
In summary, a comprehensive understanding of DNA mutations encompasses their types, causes, effects on health, and the evolving research landscape. These elements are not just academic—they have real-world implications for life sciences and medical fields.
Prologue to DNA Mutations
Definition of DNA Mutation
DNA mutations refer to permanent alterations in the nucleotide sequence of an organism's genetic material. These alterations can occur in various forms, including substitutions, insertions, or deletions of nucleotides. A mutation can affect a single nucleotide base or extended sequences, ultimately influencing how genes function. The implications of these mutations can vary widely, from benign variations to significant impacts on health and development.
Historical Background of DNA Mutations
The notion of DNA mutations and their implications have evolved over time. Early studies focused on mutations observed in organisms like fruit flies, shedding light on inheritance patterns and genetic variation. The first insights into mutation mechanisms came from the work of scientists such as Thomas Morgan in the early 20th century. As molecular biology advanced, particularly in the mid-20th century, researchers began to link mutations directly to changes in DNA sequences. The development of techniques such as DNA sequencing has since further illuminated the types and consequences of these mutations, paving the way for genetic research as we know it today.
The study of DNA mutations has significantly contributed to our understanding of genetics and evolutionary biology.
In summary, recognizing the significance of DNA mutations opens pathways to diverse discussions about genetics, health, and evolution. Understanding these aspects helps in advancing research, such as gene editing and therapeutic interventions.
Types of DNA Mutations
DNA mutations are crucial to understand due to their role in genetic variability, evolution, and disease. These alterations in the genetic code can lead to significant changes in an organism. Their classification helps researchers pinpoint how these changes affect individual health and species evolution. In this section, we will discuss the main types of DNA mutations, focusing on their characteristics and implications.
Point Mutations
Point mutations are the most common type of DNA mutation. A point mutation occurs when a single nucleotide change leads to a different amino acid in a protein or a premature stop codon. These mutations are classified into different categories: missense, nonsense, and silent mutations.
- Missense mutations change one amino acid to another, potentially altering protein function. An example can be found in sickle cell anemia, where a single nucleotide change in the hemoglobin gene causes a severe alteration of the blood's oxygen-carrying capacity.
- Nonsense mutations create a stop codon prematurely, truncating the protein and often leading to loss of function. This can have devastating effects if a critical protein is affected.
- Silent mutations do not change the protein's amino acid sequence due to the redundancy of the genetic code, generally having little effect on the organism.
Point mutations, though small in scale, can have a large impact on protein function and are key to understanding various genetic conditions.
Insertions and Deletions
Insertions and deletions, collectively called indels, are another crucial type of mutation.
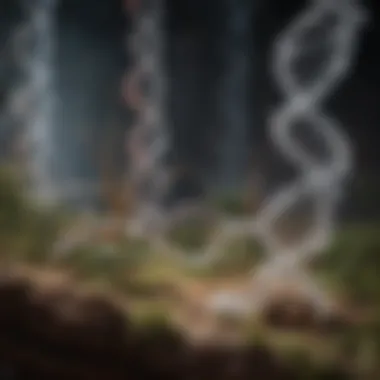
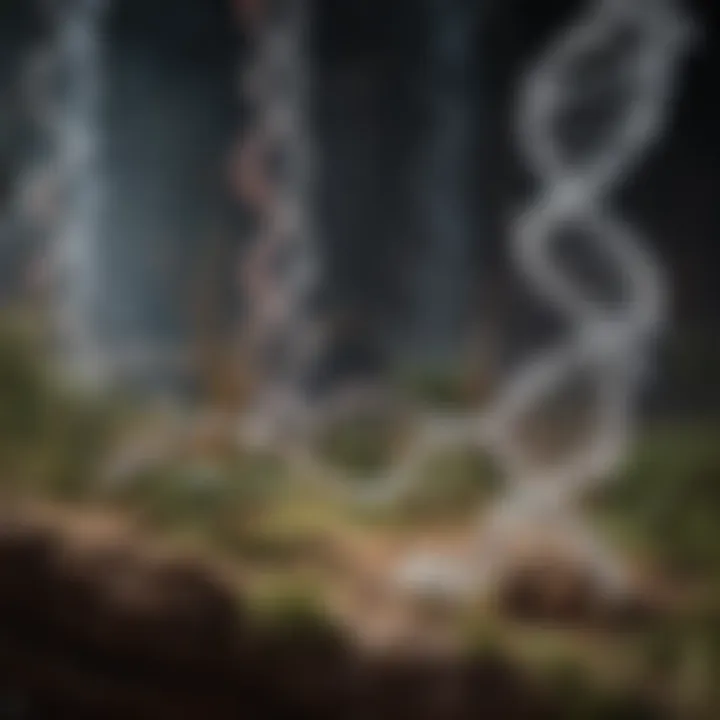
- Insertions involve the addition of one or more nucleotide bases into a DNA sequence. This could lead to alterations in the reading frame, especially when they do not occur in multiples of three.
- Deletions entail the loss of nucleotide bases from a DNA sequence. Like insertions, deletions can shift the reading frame, causing frameshift mutations.
Both types of mutations can affect gene function and protein synthesis significantly. Their occurrence may lead to diseases, including various cancers and genetic disorders. The understanding of indels enhances our insights into the complexity of genetics and its impact on health.
Frameshift Mutations
Frameshift mutations arise from insertions or deletions that are not in multiples of three nucleotides. This shift causes a change in how the genetic code is read by ribosomes during translation.
As a result, every amino acid downstream of the mutation can be altered, usually creating a nonfunctional protein. The consequences of frameshift mutations can be severe. They are often implicated in genetic diseases, making the study of these mutations particularly important in medical genetics and therapeutic contexts.
Large-scale Mutations
Large-scale mutations involve alterations that affect large segments of the genome, impacting one or more genes. These mutations can take the form of duplications, inversions, large deletions, or even chromosomal translocations.
- Duplications create copies of segments of DNA, potentially leading to gene dosage effects.
- Inversions rearrange the structure of chromosomes, which may disrupt gene function.
- Translocations involve the exchange of segments between non-homologous chromosomes, which can result in cancer if key regulatory genes are affected.
These mutations are particularly relevant in the context of cancers and other complex diseases. Understanding their specific patterns can provide insight into the etiology of these diseases, significantly contributing to genetic research and therapeutic strategies.
Mechanisms Causing DNA Mutations
Understanding the mechanisms that cause DNA mutations is essential for comprehending their implications in both evolution and various diseases. These mechanisms can be categorized into spontaneous and induced mutations, which are influenced by environmental factors. By grasping these elements, researchers can identify methods for mutation prevention and repair.
Spontaneous Mutations
Spontaneous mutations arise without any external influence. They typically occur during DNA replication processes when errors happen. These errors may include misincorporation of nucleotides, which can result from the natural instability of the DNA molecule. While the body has repair mechanisms in place, some mutations escape correction.
These mutations play a significant role in genetic diversity. Some spontaneous mutations can confer advantageous traits, allowing for adaptation over generations. However, not all mutations are beneficial; some can lead to detrimental effects on an organism's health. This makes the understanding of spontaneous mutations vital for both evolutionary biology and medical research.
Induced Mutations
Induced mutations arise from external factors. They occur when DNA is damaged by agents such as radiation or chemicals. Unlike spontaneous mutations, induced mutations are largely preventable, which makes this area of study crucial for public health.
Research indicates that certain conditions can exacerbate induced mutations, leading to the development of diseases like cancer. Preventing such mutations requires understanding their origins and effects. The knowledge of how environmental agents contribute to DNA mutations enables the formulation of regulatory measures to mitigate risks associated with exposure.
Environmental Factors
Environmental factors are a significant source of induced mutations. Key aspects include radiation, exposure to chemicals, and bacterial influence, which can all alter DNA by causing breaks or incorrect repairs.
Radiation
Radiation is a well-known mutagen. It can break DNA strands, creating lesions that lead to mutations if not repaired correctly. The key characteristic of radiation is its ability to penetrate tissues, causing damage at a cellular level. This makes it an important focus in studies related to cancer prevention.
A unique feature of radiation as a mutation source is that it can affect not only the directly exposed cells but also nearby cells through signaling mechanisms. While understanding its effects is vital, overexposure is clearly hazardous. Thus, knowing safe levels of radiation is essential for public health guidelines.
Chemicals
Chemicals represent another significant source of induced mutations. Numerous chemical agents can interact with DNA, resulting in measurable mutations. One characteristic that distinguishes these agents is their varied interactions with different cellular components.
This makes chemicals an adaptable choice for experimentation in mutation studies. Researchers can assess how different compounds cause alterations in genetic material. However, certain chemicals may also present risks, prompting calls for increased regulation and public awareness regarding exposure.
Bacterial Influence
Bacterial influence on DNA can lead to mutations through various mechanisms. Some bacteria can introduce their genetic material into host organisms. This unique feature contributes to genetic variation within populations, potentially leading to adaptive changes.
While the interaction between bacteria and host DNA may offer beneficial outcomes, such as the exchange of advantageous traits, it is not without risk. Bacterial-induced mutations can also result in pathogenic variations that challenge immune responses. Therefore, studying this influence helps researchers understand both evolutionary processes and disease development.
Understanding the mechanisms of DNA mutations is key to developing strategies to mitigate their harmful effects while harnessing their contributions to diversity.
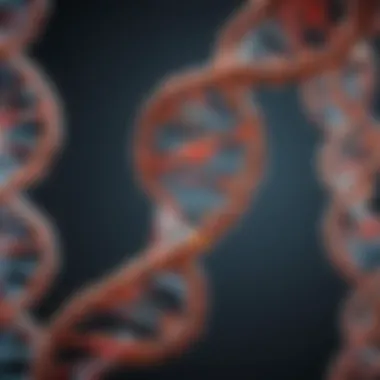
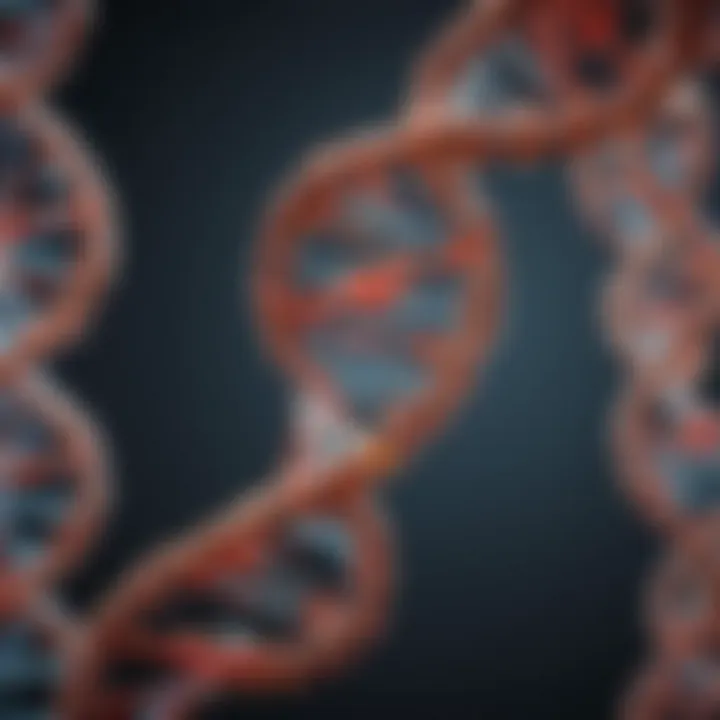
Effects of DNA Mutations
DNA mutations can have far-reaching implications for various aspects of biological functions. Understanding these effects is crucial for a comprehensive grasp of genetics, evolutionary biology, and health sciences. They contribute to the diversity of life and the adaptability of organisms but can also lead to serious health issues. This section discusses several key areas impacted by DNA mutations: their effect on protein function, their role in genetic diversity, and their association with diseases.
Impact on Protein Function
Mutations can directly influence the proteins synthesized in the body. Proteins are essential macromolecules that perform a multitude of functions such as catalyzing biochemical reactions, providing structure, and facilitating communication between cells. When a mutation occurs in a gene, it may lead to an altered or malfunctioning protein, determining whether that protein will still be functional. This outcome can vary widely. For instance, a single point mutation could replace one amino acid in a protein, potentially rendering it less effective or, in some cases, entirely dysfunctional. Such changes can disrupt normal cellular processes and lead to disease states.
In contrast, some mutations may enhance the function of a protein, leading to advantageous traits that may be selected for in certain environments.
Contribution to Genetic Diversity
Mutations serve as a fundamental source of genetic variation within populations. This genetic diversity is essential for the process of evolution. When organisms reproduce, the mutations they carry can introduce new traits in future generations. Over time, beneficial mutations may become more common, while harmful ones may be filtered out through natural selection.
The introduction of new alleles allows populations to adapt to changing environments. The interplay between mutation rate, population size, and environmental pressures can significantly shape the genetic landscape of organisms. This continuous change fuels evolutionary processes and reflects the dynamic nature of life on Earth.
Association with Diseases
DNA mutations are closely linked to an array of diseases. Understanding this association is vital to finding diagnostics and therapeutics.
Cancer
Cancer is a prominent example of how mutations can influence health. Specific mutations can lead to uncontrolled cell growth, a hallmark of cancer. Tumor cells often harbor multiple mutations that contribute to their malignant behavior. The key characteristic of cancer in this context is that it is not just one mutation causing disease but rather a series of genetic alterations that lead to a pathological state. This relevance makes cancer a significant focus in genetic research. By studying the specific mutations involved in different cancers, we can better understand tumorigenesis and develop targeted therapies.
Genetic Disorders
Genetic disorders are another crucial area impacted by DNA mutations. These disorders arise from mutations that disrupt normal gene function. Conditions such as cystic fibrosis or sickle-cell disease are direct results of specific mutations. The key characteristic here is that, unlike cancer, where mutations may result in a gain of function, genetic disorders often result in a loss of function that has significant health implications. Addressing these mutations is critical for diagnosis and management of such conditions. The unique feature of genetic disorders is their hereditary nature, influencing not just individuals but also entire families and populations.
In summary, the effects of DNA mutations span across multiple domains. From altering protein function to guiding evolution and influencing health, comprehending these implications deepens our understanding of biology and medicine. Continued research in this area promises to yield insights that could lead to innovative treatments for diseases that currently have limited options.
DNA Repair Mechanisms
DNA repair mechanisms are essential to maintaining the integrity of genetic information in all living organisms. They play a crucial role in preventing the accumulation of mutations that can lead to diseases, including cancer. In the context of this article, understanding these mechanisms aids in grasping how mutations are not just detrimental but can also be corrected to preserve cellular function and organismal health. Repair mechanisms act as a defense system against various types of DNA damage, showcasing the sophistication of biological systems. This section will delve into the types of repair mechanisms, highlighting their unique characteristics and importance.
Types of DNA Repair Mechanisms
Base Excision Repair
Base Excision Repair (BER) is vital for correcting single-base lesions in DNA. It mainly targets damaged bases that have been altered by oxidative stress or deamination. The key characteristic of BER is its ability to remove and replace damaged nucleotides efficiently. This mechanism is considered a beneficial choice for maintaining genomic stability as it addresses issues before they lead to more severe mutations.
A unique feature of BER is its multi-step process, which includes recognizing the damage, cleaving the sugar-phosphate backbone, and filling in the gap with the correct nucleotide. This meticulous process provides several advantages, such as preserving DNA integrity and ensuring accurate replication. However, one disadvantage is the potential for errors if the repair steps are misregulated, leading to mutations rather than corrections.
Nucleotide Excision Repair
Nucleotide Excision Repair (NER) is crucial for repairing bulky DNA lesions, such as those caused by ultraviolet (UV) light. Its main characteristic is the removal of a short stretch of DNA surrounding the damage before synthesis of a new segment. NER is particularly important for organisms exposed to environmental mutagens.
The unique feature of NER is that it can remove a wide range of DNA lesions, making it a versatile repair mechanism. This flexibility allows NER to address various types of DNA damage effectively, which is essential for protecting cells from potential carcinogenesis. Nonetheless, the complexity of its machinery requires a considerable amount of cellular resources, which can be a disadvantage in high-stress cellular environments.
Mismatch Repair
Mismatch Repair (MMR) focuses on correcting errors that occur during DNA replication, specifically base-pair mismatches. The main characteristic of MMR is its ability to recognize and correct replication errors, which helps maintain genetic fidelity. This mechanism is beneficial because it significantly reduces the frequency of mutations that might otherwise propagate through generations.
MMR's unique feature lies in its discrimination between parental and newly synthesized strands, allowing for accurate repair of mismatched bases. However, the process can become inefficient when there are multiple errors, potentially leading to undetected mutations, thus decreasing its overall effectiveness.
Importance of DNA Repair
DNA repair is indispensable for the survival of all organisms. The importance of these mechanisms extends beyond simple error correction; they are fundamental in preventing terminal genomic instability, which can lead to diseases. Proper functioning of repair pathways ensures that genetic variations, which may arise from mutations, result in adaptability rather than pathogenesis. Continuous research in this area promises potential therapeutic strategies, emphasizing the need for a deeper understanding of DNA repair as it relates to health, disease, and evolutionary processes.
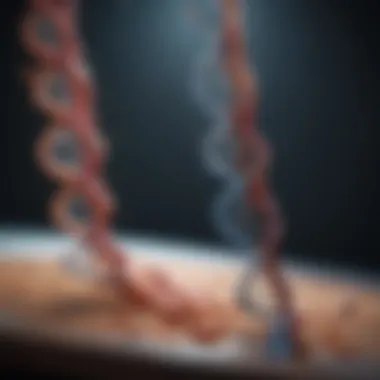
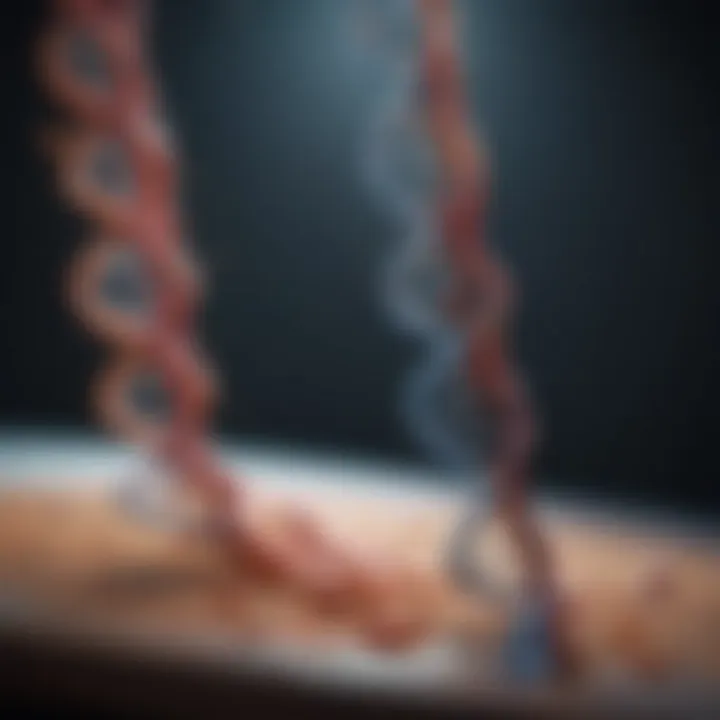
"DNA repair mechanisms exemplify the intricate balance between mutation, evolution, and maintenance of life itself."
Research Implications of DNA Mutations
Understanding the implications of DNA mutations is critical in various fields of biological research. These mutations can influence evolutionary processes, impact health, and lead to genetic disorders. Therefore, researching DNA mutations is pertinent for developing therapeutic strategies and understanding fundamental biological mechanisms. Moreover, by focusing on the mutation implications, researchers can identify potential areas for intervention in diseases linked to genetic changes.
In the context of research, the implications of DNA mutations extend to improving our approach to gene therapy, cancer research, and personalized medicine. Each of these areas aims to provide better outcomes for patients through targeted treatments based on specific genetic profiles. The investigations into DNA mutations also help in understanding the mechanisms of mutation, which is crucial for advancing scientific knowledge and technology in genetics.
Current Research Trends
New frontiers continue to open in the field of DNA mutations. Recent trends focus on the genetic basis of diseases, the role of epigenetics in mutation expression, and how environmental factors contribute to mutation rates. Studies also investigate the long-term effects of specific mutations on individual health and population diversity. Using tools like genome sequencing and bioinformatics, researchers can gather valuable data, identifying patterns and establishing correlations with disease mechanisms.
These insights play a significant role in driving innovations in medical treatments, underscoring the need for continuous exploration of the genetic landscape and its implications for health.
Gene Editing Technologies
Technological advancements have allowed researchers to manipulate DNA with a high degree of precision. Among these advancements, several gene editing technologies stand out. Tools like CRISPR, TALEN, and Zinc Finger Nucleases offer various approaches to modifying genetic sequences, making them invaluable in studying and potentially correcting mutations.
CRISPR
CRISPR (Clustered Regularly Interspaced Short Palindromic Repeats) is a groundbreaking tool that enables precise edits in the DNA sequence. Its most prominent contribution is simplifying the process of targeting and altering genes. The key characteristic of CRISPR is its use of a guide RNA to direct the Cas9 enzyme to the specific location in the genome. This specificity makes CRISPR a popular choice for genetic research, especially in studying gene functions and potential therapies for genetic disorders. The unique feature of CRISPR lies in its efficiency and versatility, allowing multiple genes to be targeted simultaneously. However, its disadvantages include off-target effects, which might result in unintended edits.
TALEN
TALEN (Transcription Activator-Like Effector Nucleases) offers a customizable method for gene editing. This technology is based on the principle of using DNA-binding proteins fused with a nuclease to make cuts at specific sites in the genome. Its key characteristic is the capability to design TALENs for almost any target sequence, making it a flexible option for researchers. TALENs are effective in generating knockout models and studying gene functions. However, the assembly of TALEN constructs can be complex and time-consuming, which is a limitation compared to more straightforward options like CRISPR.
Zinc Finger Nucleases
Zinc Finger Nucleases (ZFNs) combine zinc finger DNA-binding domains with a nuclease. They allow targeted editing of the genome, contributing to research in gene therapy and genetic correction. The key characteristic of ZFNs is their modular design, enabling researchers to create custom-designed nucleases tailored to specific sequences. Although they are beneficial for detailed genetic modifications, they are less commonly used today due to the complexity and cost involved in developing ZFN constructs. Additionally, they may have off-target effects, similar to CRISPR.
Overall, gene editing technologies represent pivotal advancements in the understanding and manipulation of DNA mutations. They not only provide insights into genetic diseases but also open new avenues for therapeutic interventions.
The study of DNA mutations continues to evolve. With advancements in molecular biology and genetics, researchers are moving toward promising therapeutic approaches and novel technologies aimed at understanding and navigating these genetic alterations. This section outlines key developments and considerations that are crucial for future exploration in the field of DNA mutations.
Potential Therapeutic Approaches
In recent years, scientists have begun to explore a variety of therapeutic strategies designed to address the consequences of DNA mutations. These approaches can potentially correct or mitigate the effects of harmful mutations, which is particularly relevant in the context of genetic disorders and cancers. Some of the most notable methods include:
- Gene Therapy: This method involves introducing, removing, or altering genetic material within a patient’s cells to treat or prevent disease. Gene therapy aims to target the underlying genetic defects that contribute to various conditions.
- CRISPR-Cas9 Technology: CRISPR is a groundbreaking technology that allows for precise editing of DNA. It has generated significant excitement due to its potential for correcting mutations at specific sites in the genome. By utilizing guide RNAs, researchers can cut the DNA at desired locations, which facilitates gene editing.
- Base Editing: A more advanced form of gene editing, base editing, allows scientists to directly convert one DNA base into another without causing double-strand breaks in the DNA. This method has shown promise for correcting point mutations linked to genetic diseases.
- RNA Interference: This technique utilizes small RNA molecules to inhibit the expression of specific genes that are mutated or expressed abnormally. By selectively silencing these genes, RNA interference can potentially reverse disease processes.
Ethical Considerations
As technologies advance, the ethical implications surrounding DNA mutations also intensify. The ability to manipulate genetic material raises profound questions regarding consent, equity, and the long-term consequences of such interventions. Some primary concerns include:
- Gene Editing Ethics: Editing the human genome could lead to unexpected side effects or changes in future generations. This raises questions about who has the authority to make such changes and the potential for unintended consequences.
- Accessibility of Treatments: There is a risk that advanced therapies will be accessible only to affluent populations, exacerbating existing inequalities in healthcare. Ensuring that these groundbreaking treatments are equitably available is critical.
- Regulation and Oversight: As with any new technology, regulatory frameworks must be developed to govern the use of gene editing. These regulations must balance innovation with patient safety and ethical considerations.
- Germline Vs. Somatic Editing: The decision to edit germline cells—which could pass changes to future generations—versus somatic cells poses ethical dilemmas. Germline editing may prevent certain hereditary diseases, but also leads to debates over "designer babies" and genetic enhancement.
"The role of ethics in genetic research cannot be understated, as it guides responsible implementation of new scientific advancements."
Finale
The exploration of DNA mutations is essential for understanding various biological processes that affect all forms of life. This article has delved into the nature and significance of DNA mutations, touching upon their types, causes, consequences, and repair mechanisms. Each aspect discussed turns out to be interlinked, illustrating how mutations form the foundation of genetic variability, which is crucial for evolution. Furthermore, the relationship between mutations and diseases, particularly cancers and genetic disorders, highlights the importance of this area of study in medical research.
Summary of Key Points
In summary, the key points discussed in this article include:
- Definition and Types of Mutations: Understanding point mutations, insertions, deletions, frameshift mutations, and large-scale mutations sheds light on the complexity of genetic changes. Each type can have different implications on an organism's traits.
- Mechanisms Leading to Mutations: Both spontaneous and induced mutations occur through natural processes and environmental influences, respectively. This reveals how external factors like radiation or chemicals can alter genetic material.
- Effects on Health: The role mutations play in protein function can lead to various cellular outcomes. Understanding these effects is vital for grasping the mechanisms behind diseases and conditions.
- DNA Repair Mechanisms: Highlighting the various repair processes underscores nature’s own systems of correcting errors, emphasizing the significance of these mechanisms in maintaining genome integrity.
- Research Trends: Current advancements in gene editing technologies, such as CRISPR, present exciting opportunities to potentially correct harmful mutations in the future.
The Importance of Ongoing Research
Ongoing research in the field of DNA mutations is critical. As scientists make strides in understanding the mechanisms behind mutations and their consequences, they also open new pathways for medical intervention.
- Potential Therapeutic Approaches: By further elucidating the connection between specific mutations and diseases, targeted therapies may arise. This could lead to personalized medicine approaches that improve treatment outcomes for those affected by genetic disorders.
- Ethical Considerations: Alongside scientific advancements come ethical dilemmas. Research must consider the implications of editing genetic material and the long-term effects it may have on future generations.