Understanding DNA Precipitation in Molecular Biology
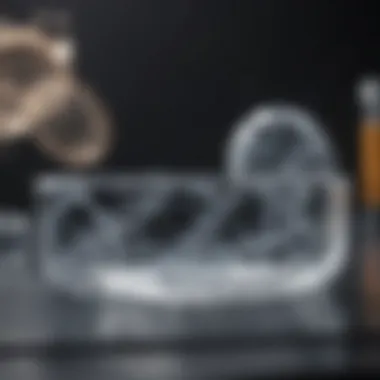
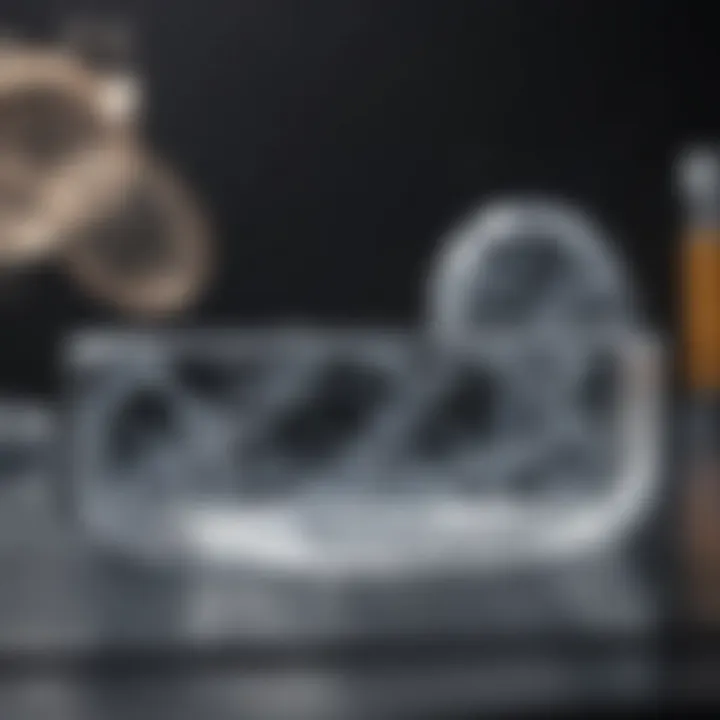
Intro
DNA precipitation is a pivotal technique in molecular biology. It serves as a primary method for the extraction and purification of deoxyribonucleic acid. By understanding the process behind DNA precipitation, researchers can effectively isolate genetic material for various applications. This article aims to demystify the intricacies of this process, covering its practical applications, the underlying chemistry, and the established protocols.
The relevance of DNA precipitation cannot be overstated. It is a backbone procedure in many laboratory settings, especially where accurate DNA quantification is essential. As the landscape of molecular biology evolves, so does the importance of mastering this technique. This comprehensive exploration will not only address the mechanics of this process but also highlight its significance in modern scientific inquiry.
Moving forth, we will analyze the different factors that affect the efficiency of DNA precipitation. Each component of this method—from the reagents used to the environmental conditions—plays a critical role in the overall outcome. Moreover, we will outline practical tips and protocols employed in various settings to ensure optimal results.
Through this examination, readers will gain valuable insights into DNA precipitation. The information presented will aid both novices and experienced professionals alike, enriching their understanding of this essential laboratory technique.
Preamble to DNA Precipitation
DNA precipitation plays a crucial role in the field of molecular biology. It is a technique traditionally employed for the extraction and purification of DNA from various sources, including cells and tissues. Understanding this process forms the foundation for more complex genetic analyses, such as sequencing, cloning, and PCR amplification. This section emphasizes the significance of DNA precipitation in both research and diagnostic environments.
The process of DNA precipitation involves isolating DNA by manipulating its solubility. This is typically achieved through the use of alcohol, which leads to the formation of a visible DNA pellet once centrifuged. While this may seem simple, the myriad of factors that affect precipitation efficiency makes mastering this technique vital for scientists.
Understanding DNA
To appreciate the importance of DNA precipitation, one must first grasp what DNA is. Deoxyribonucleic acid, or DNA, is the hereditary material found in all living organisms. It carries the genetic instructions for development, functioning, growth, and reproduction. Contained within the cellular structure, DNA's role as a blueprint for life necessitates its purification and analysis in many scientific studies. This highlights the relevance of having precise techniques to isolate DNA effectively.
The Importance of DNA Purification
DNA purification is essential for various reasons. First, it removes contaminants that can interfere with subsequent analyses. These contaminants could include proteins, lipids, and other nucleic acids which can alter experimental outcomes. High-quality DNA ensures accurate results in methods such as qPCR and sequencing.
Moreover, purified DNA is critical in clinical diagnostics. It aids in identifying genetic disorders and pathogens, enabling tailored treatment plans. Accessibility to well-purified DNA is equally vital in genetic research, contributing to the understanding of hereditary diseases and potential genetic therapies.
In summary, the discussion on DNA precipitation extends beyond the laboratory bench. The ramifications of this technique resonate through various fields such as medicine, forensics, and agriculture, where DNA analysis frequently arises.
"Effective DNA purification lays the groundwork for all further applications in genetic science."
Understanding DNA precipitation, therefore, is not only vital for laboratory practices but also for advancements in a broad range of scientific disciplines.
The Chemistry Behind DNA Precipitation
Understanding the chemistry behind DNA precipitation is fundamental for those involved in molecular biology. This process is essential for isolating and purifying DNA from various biological samples, making it a key technique in many laboratory protocols. Analyzing the interactions at the molecular level helps in optimizing the overall efficiency of DNA extraction.
When discussing the chemistry of DNA precipitation, we focus on three primary components: alcohol, ionic strength, and pH levels. Each of these elements plays a pivotal role in determining the success of the precipitation process. Having a solid grasp of how these factors influence DNA solubility and stability allows researchers to tailor conditions for maximal yield and purity.
Role of Alcohol in DNA Precipitation
Alcohol, particularly ethanol or isopropanol, is crucial in the DNA precipitation process. Adding alcohol decreases the solubility of DNA in solution, leading to its aggregation. This aggregation occurs because alcohol disrupts the hydration shell that surrounds the DNA molecules, reducing their solubility. Ethanol is commonly used due to its ability to efficiently precipitate nucleic acids while minimizing other contaminants.
The ratio of alcohol to the aqueous phase is significant. A common practice involves adding twice the volume of alcohol to the DNA solution. This ensures a sufficient level of alcohol to facilitate effective precipitation while still allowing some buffer components to remain in the solution. Failure to add enough alcohol may result in low yield.
Ionic Strength and its Effect
Ionic strength refers to the concentration of ions in a solution. It significantly affects the precipitation of DNA. When the ionic strength increases, the repulsive forces between negatively charged phosphate groups in the DNA backbone are reduced. This reduction encourages the DNA strands to come together and aggregate more readily.
Sodium acetate is a valuable reagent that enhances ionic strength during precipitation processes. By carefully adjusting the ionic strength, researchers can modify DNA solubility. A high ionic strength environment can result in a more efficient DNA precipitation process, leading to higher yields.
pH and Its Influence on Precipitation
The pH of a solution can have a profound effect on DNA precipitation. The phosphate backbone of DNA carries a negative charge, which can be affected by the pH level. When the pH is too low, protons can neutralize some of the negative charges, hindering the aggregation of DNA. Conversely, if the pH is too high, the stability and solubility of DNA can also be compromised.
The optimal pH for DNA precipitation usually falls between 5.0 and 7.0. During this range, the negative charges on the DNA are maximized while still maintaining solubility. Therefore, monitoring and adjusting pH are critical steps in achieving high-quality DNA yields. Proper buffers, like Tris or phosphate buffers, can help maintain stable pH levels during the precipitation.
In summary, the chemistry behind DNA precipitation involves understanding the roles of alcohol, ionic strength, and pH. Appropriate manipulation of these factors can significantly enhance DNA yield and purity.
Step-by-Step Procedure for DNA Precipitation
The procedure for DNA precipitation is vital in molecular biology, providing a structured course of action that ensures efficient extraction and purification of DNA. Each step in this process has a specific purpose and contributes to the overall success of isolating pure DNA. Understanding these steps enables researchers and students to perform the technique accurately and consistently. The following sections break down each component into key actions that need to be implemented carefully for optimal results.
Sample Preparation Techniques
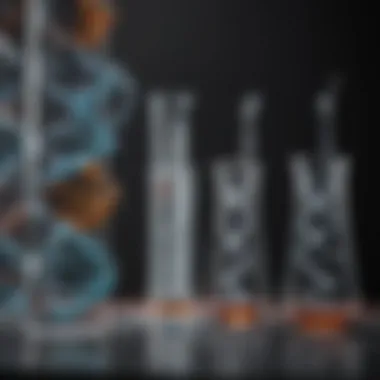
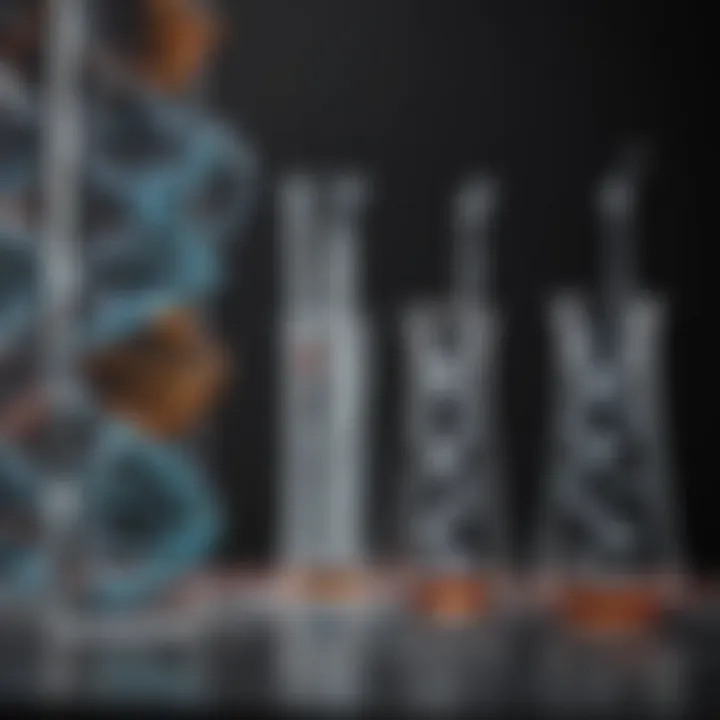
Before starting the DNA precipitation process, proper sample preparation is essential. This step involves the collection of biological samples which can vary from blood, tissues, or cell cultures. The method of extraction will depend on the source of the sample. It’s important to handle the samples with care to avoid degradation.
- Lysis of Cells: A lysis buffer containing detergents and enzymes is used to break open the cells and release the DNA into the solution. This step must be optimized to ensure that all cellular components are effectively disrupted, without damaging the DNA.
- Incubation Time: Following the addition of the lysis buffer, an incubation period at correct temperature should be maintained. This assists in enhancing the lysis efficiency, which directly influences DNA yield.
Adding Precipitation Reagents
Once the samples are prepared, the next step involves the addition of precipitation reagents, usually ethanol or isopropanol. These reagents play an important role in the DNA retrieval process.
- Alcohol Selection: Ethanol is the most commonly used, typically at 70% or 100%. Low temperatures enhance the effectiveness of this step.
- Volume Consideration: The volume of alcohol added should be at least two to three times the volume of the DNA solution. This ratio helps to ensure adequate precipitation of the DNA.
- Mixing Approach: Gentle mixing of the solutions is important. Too vigorous mixing can shear the DNA, leading to lower yields.
Centrifugation and Its Importance
Centrifugation is a crucial step in the DNA precipitation process. This technique separates DNA from the solution, allowing for collection of purified nucleic acids.
- Centrifuge Settings: It is vital to set the centrifuge at an appropriate speed and duration based on the protocols. Higher speeds can lead to more efficient sedimentation of DNA.
- Supernatant Removal: After centrifugation, the supernatant is carefully removed to avoid disturbing the DNA pellet. This ensures that the DNA remains concentrated and undiluted.
Washing and Resuspension Steps
The final stage of the DNA precipitation involves washing and resuspending the DNA pellet. Proper washing is significant to remove any residual salts or reagents that may interfere with downstream applications.
- Washing with Ethanol: Typically, 70% ethanol is used to wash the DNA pellet. This helps to precipitate out any remaining impurities. Care should be taken to avoid over-washing, as this can lead to loss of DNA.
- Resuspension Solution: Once washed, the DNA pellet must be resuspended in a suitable buffer, such as TE buffer. This buffer provides stability and protects the DNA from degradation during storage.
In summary, the step-by-step procedure for DNA precipitation is fundamental in molecular biology laboratories. Each step should be executed carefully to ensure effective yield and quality of DNA for further analysis.
Factors Influencing DNA Precipitation Efficiency
Understanding the factors that influence DNA precipitation efficiency is crucial for achieving optimal results in molecular biology techniques. The efficiency of DNA precipitation can be affected by various parameters, including temperature, the quality of reagents, and the volume of the sample. Each of these elements plays a significant role in determining the yield and purity of the DNA extracted.
Temperature Considerations
Temperature is a key factor in the precipitation of DNA. Generally, performing the precipitation at lower temperatures can enhance DNA recovery. When ethanol or isopropanol is added at cold temperatures, it promotes the aggregation of DNA, resulting in better precipitation. Cold temperatures help in reducing the solubility of nucleic acids, leading to a higher yield of recovered DNA.
Researchers often recommend chilling the reagents and the sample before precipitation. This practice ensures that the DNA remains in a less soluble form, thus enhancing the effectiveness of the precipitation process. Additionally, maintaining consistent temperature during the procedure is vital, as fluctuations can introduce variability in yield and purity.
Reagent Quality and Concentration
The quality and concentration of reagents are significant determinants of DNA precipitation efficiency. Using high-quality ethanol or isopropanol is essential. Impure or degraded reagents can lead to incomplete precipitation or co-precipitation of unwanted materials, which can contaminate the DNA sample.
The concentration of the alcohol used should ideally be around 70% for optimal results. At this concentration, the alcohol efficiently interacts with the DNA while minimizing the solvation effects of water. Moreover, the addition of salts, such as sodium acetate, can increase ionic strength, further facilitating DNA precipitation when combined with the appropriate alcohol concentration. Ensuring that reagents are fresh and properly stored also contributes to their effectiveness in the protocol.
Sample Volume Impacts
The volume of the sample used in DNA precipitation can greatly influence the outcome as well. When working with large volumes, the concentration of DNA may become diluted. This dilution can hinder the efficiency of precipitation, as the necessary conditions for effective aggregation may not be met. Conversely, very small sample volumes may not provide enough material to yield satisfactory results post-precipitation.
It's important to optimize the sample size to balance the concentration of DNA. Researchers typically recommend keeping sample volumes in a range that allows for effective precipitation while ensuring sufficient amounts of purified DNA for downstream applications.
In summary, understanding these factors allows researchers to refine their DNA precipitation protocols, ensuring better yields and purer samples. Effective management of temperature, reagent quality, and sample volume is essential in achieving high efficiency in DNA precipitation.
"Optimizing the conditions surrounding DNA precipitation is key for researchers looking to extract the highest quality DNA for various applications."
By paying attention to these details, both novice and experienced professionals can enhance their results in DNA extraction and purification processes.
Common Protocols in DNA Precipitation
The protocols utilized in DNA precipitation are vital in ensuring that the extraction and purification of DNA are efficient and effective. Understanding these protocols allows researchers and professionals to optimize their methodologies tailored to their specific objectives. Techniques differ primarily based on the reagents used, conditions applied, and desired output.
By implementing standardized protocols, one can achieve reproducibility and reliability in results. Protocols can also be adapted easily based on the type of sample or desired purity level. The knowledge of common protocols provides a foundation for developing advanced techniques and troubleshooting issues that may arise during DNA extraction.
Alcohol-Based Precipitation Protocols
Alcohol-based precipitation is a widely recognized method for isolating DNA from biological samples. This protocol generally involves the use of either ethanol or isopropanol, which facilitates the precipitation of DNA from the solution. The fundamental principle lies in the fact that DNA is not soluble in alcohol, particularly when salt is present, thereby enabling its selective extraction.
In terms of procedure, alcohol-based methods usually follow these steps:
- Mixing with Alcohol: The biological sample is mixed with an alcohol solution after the addition of salt. The combination allows the nucleic acids to clump together and become insoluble.
- Incubation: Incubation periods typically range from 30 minutes to overnight, depending on the protocol. This allows sufficient time for the DNA to precipitate out of the solution.
- Centrifugation: The mixture is then centrifuged to separate the precipitated DNA from the supernatant. This step is crucial for obtaining a clean pellet of DNA.
- Washing: The DNA pellet is washed with alcohol to remove any residual salts or impurities.
- Resuspension: Finally, the DNA is resuspended in an appropriate buffer for subsequent applications.
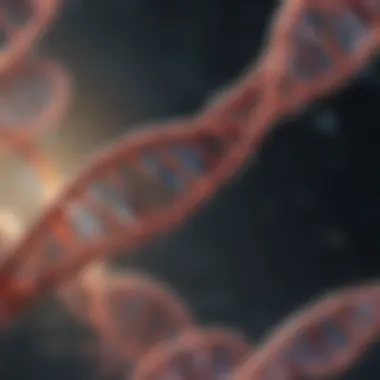
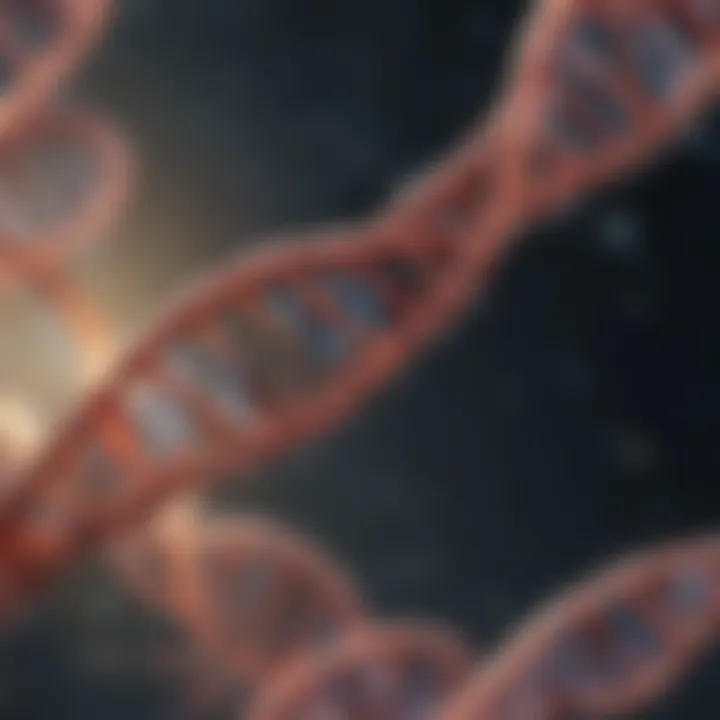
"The choice between ethanol and isopropanol can influence yield and purity; thus, the desired application should guide the selection of alcohol."
This method is favored for its simplicity and effectiveness. However, careful attention must be paid to the quality of reagents, as impurities can negatively affect DNA integrity.
Salt-Enhanced Precipitation Methods
Salt-enhanced precipitation methods bolster alcohol-based protocols, often using sodium acetate or ammonium acetate. The addition of salt assists in neutralizing the charge on the DNA molecules, facilitating their aggregation and sedimentation when alcohol is added.
The procedure includes:
- Preparation of Solution: Start by dissolving sample with water along with an appropriate concentration of salt.
- Addition of Alcohol: Add cold alcohol to the saline solution. This prompts the DNA to precipitate out because of decreased solubility in the presence of salts.
- Centrifugation: Similar to alcohol protocols, centrifugation is used to gather the precipitated DNA.
- Washing and Resuspension: As before, the DNA pellet is washed and then reconstituted in a buffer solution.
This method is particularly effective when dealing with challenging samples or instances where higher purity is desired. Salt enhances the yield and quality of the DNA extracted, making it a valuable consideration in DNA precipitation protocols.
Both alcohol-based and salt-enhanced methods play crucial roles in molecular biology, each offering unique strengths tailored to specific research needs.
Applications of DNA Precipitation
The utilization of DNA precipitation in molecular biology extends far beyond simple extraction methods. This technique plays a crucial role in various scientific fields, offering operational advantages and providing reliable data essential for further research. The applications of DNA precipitation are wide-ranging, emphasizing its importance in clinical diagnostics, genetic research, and forensic science.
Clinical Diagnostics
In clinical diagnostics, DNA precipitation is central to obtaining pure DNA samples from blood, saliva, or other biological fluids. The quality and purity of the DNA are essentials factors that affect downstream applications such as PCR (polymerase chain reaction) or sequencing. Precise DNA extraction allows for accurate genetic testing, which has implications in diagnosing genetic disorders, identifying infectious diseases, and tailoring personalized treatment options. The process ensures that contaminants do not interfere with assays, reducing the risk of false positives or negatives.
Genetic Research
Genetic research is another area where DNA precipitation is notably significant. Researchers rely on high-quality DNA for studies involving genome sequencing, gene expression analysis, and cloning. When isolating DNA via precipitation, researchers can maintain the structural integrity of the nucleic acids. This is vital for studies that seek to understand genetic variations or mutations within populations. Additionally, the ability to fine-tune precipitation conditions allows for the recovery of DNA from diverse sources, promoting versatility in research methodologies.
Forensic Science
In forensic science, DNA precipitation serves a critical function in evidence analysis. The ability to extract DNA from biological samples collected at crime scenes is a key component of modern forensic investigations. These samples, which may include blood or hair, require effective purification to yield reliable results for analysis. The methods used in DNA precipitation enable forensic scientists to retrieve usable DNA, which is essential for matching suspects to evidence or exonerating the innocent. With the advancement of technology, exploiting DNA precipitation in forensic science continues to enhance the accuracy and reliability of forensic evidence.
"DNA precipitation is not just a technique; it is a gateway to understanding life at a molecular level, impacting diverse fields such as medicine, research, and justice."
Advanced Techniques in DNA Precipitation
In the realm of molecular biology, advanced techniques in DNA precipitation represent a significant evolutionary step in DNA recovery methods. These innovations not only streamline the process but also improve the yield and purity of extracted DNA. By incorporating these techniques, researchers can achieve enhanced results in their experiments and applications.
Magnetic Bead-Based DNA Precipitation
Magnetic bead-based DNA precipitation has gained traction in recent years. This method uses specially coated beads that bind to DNA in the presence of specific conditions, facilitating an efficient recovery process. The advantages of this technique include:\n
- Speed: Magnetic beads allow for rapid binding and separation. This minimizes the time required for DNA extraction compared to traditional centrifugation methods.
- Efficiency: The surface area of the beads enhances the binding capacity, often leading to higher yields of DNA.
- Purity: Because of the specific coatings on the beads, researchers can achieve a higher level of purity in the final DNA product.
In this approach, after lysing the cells and adding the appropriate buffers, magnetic beads are introduced into the solution. The mixture is subjected to a magnet, causing the beads to aggregate while the unwanted contaminants remain in the supernatant. After several washing steps, the DNA-bound beads can be resuspended in a suitable elution buffer, ready for downstream applications.
Optimizing Precipitation Conditions
Optimizing precipitation conditions is crucial for enhancing the effectiveness of DNA recovery. Several factors contribute to the overall success of this process. Key considerations include:
- Temperature: Conducting precipitation at low temperatures, such as -20 °C, can increase DNA yield. Cold conditions help to reduce solubility and encourage DNA aggregation.
- Ionic concentration: The ionic strength of the solution significantly affects DNA precipitation. Optimizing the concentration of salts, such as sodium acetate or ammonium acetate, can enhance the precipitation efficiency.
- pH Levels: Maintaining the correct pH is essential for effective DNA precipitation. Typically, a slightly alkaline pH can improve yield.
- Time: Allowing sufficient time for DNA to precipitate also plays a role. Too brief a waiting period may lead to lower recovery rates.
To achieve optimized conditions, trial and error may be necessary, involving adjustments to the above factors. Some methods might require fine-tuning to find the best conditions for a specific sample type or application.
Optimizing precipitation conditions can significantly impact the yield and purity of extracted DNA, thus influencing the success of subsequent experiments.
Troubleshooting DNA Precipitation
Troubleshooting DNA precipitation is an essential aspect of the DNA extraction process. Achieving high-quality DNA often requires careful handling and attention to detail. When problems arise, it is crucial to identify their root causes and develop effective solutions. This section aims to unravel common issues that may occur during the precipitation process, alongside recommendations for overcoming these challenges. Understanding these hurdles not only boosts overall success rates but also enhances reproducibility in experiments.
Identifying Common Issues
Identifying issues during DNA precipitation begins with careful observation. Here are some prevalent problems that can arise:
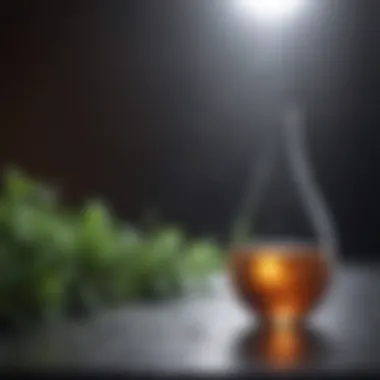
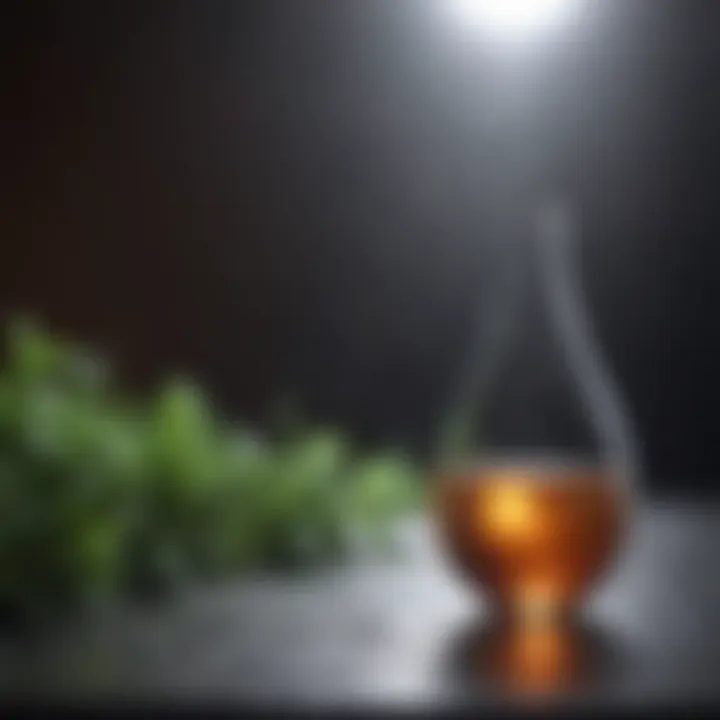
- Low DNA Yield: This is often the most significant concern. Several factors contribute to low yield, including insufficient reagent volume or inadequate sample processing.
- Contaminated Samples: Presence of contaminants like RNA or proteins can affect the purity of DNA. Analyzing sample integrity before precipitation is essential.
- Poor DNA Quality: This may manifest as degraded DNA or low absorbance ratios, indicative of impurities. Suboptimal conditions during extraction, such as temperature fluctuations, can lead to this.
- Incomplete Precipitation: This results in residual DNA remaining in the solution. Inefficient mixing or improper volume ratios of alcohol and salt can be culprits here.
Recognizing these issues lays the foundation for effective problem-solving. It allows researchers to take a proactive approach to their DNA extraction methods.
Solutions and Recommendations
Once common problems have been identified, it is essential to implement targeted solutions. Here are some recommendations to enhance DNA precipitation outcomes:
- Optimize Reagent Ratios: Always use the appropriate ratios of alcohol and salt according to the selected protocol. Optimal concentration enhances DNA yield significantly.
- Sample Preparation: Rigorously purifying your samples before DNA precipitation can minimize contamination. Techniques such as phenol-chloroform extraction can improve sample integrity.
- Conduct Control Experiments: Running control samples can help you determine whether observed issues stem from the reagents, samples, or procedural execution.
- Maintain Constant Temperature: Conduct reactions at optimal temperatures. For instance, cooling the mixture during precipitation often improves yield and purity.
- Incorporate Extended Washing Steps: Improper washing post-precipitation can leave behind contaminants. Careful washing with ethanol or isopropanol can help achieve greater purity.
As problems emerge, remaining adaptable and ready to troubleshoot is vital. Ultimately, incorporating these practices can yield higher-quality results in DNA precipitation, folding the gaps when you face challenges.
Comparative Analysis of DNA Precipitation Methods
Understanding the various methods of DNA precipitation is crucial for optimizing results in molecular biology. This section will explore both traditional and modern techniques, emphasizing their advantages, limitations, and the contexts in which they are most effective. A comparative analysis allows researchers and practitioners to make informed decisions that can significantly impact their work.
Traditional vs. Modern Techniques
Traditional DNA precipitation techniques primarily involve the use of alcohol, usually ethanol or isopropanol, along with salt to precipitate DNA from solution. This method has been a cornerstone in laboratories for decades and is known for its cost-effectiveness and simplicity.
Advantages of traditional methods include:
- Low cost due to the availability of reagents.
- Well-documented protocols that are easy to follow.
- Reliable results for small-scale projects.
However, traditional methods also have their drawbacks. They can be less efficient with very small or degraded DNA samples. Furthermore, the quality of the final DNA extract may vary based on the sample type and reagent quality.
Modern techniques, on the other hand, have introduced improvements such as magnetic bead-based DNA precipitation. This method utilizes magnetic beads coated with DNA-binding agents. As a result, it offers enhanced specificity and can efficiently purify DNA from a wider range of sample types.
Some notable advantages of modern methods include:
- Higher yield and purity of extracted DNA.
- Reduced risk of contamination during the process.
- Automation capabilities available in many modern protocols.
Nevertheless, modern techniques may come with higher costs for reagents and equipment. The complexity of some procedures could also require specialized training, which is a consideration for many labs.
In summary, the choice between traditional and modern techniques will depend on various factors such as cost, sample type, and desired yield.
Efficiency and Yield Comparisons
Efficiency and yield are critical indicators when evaluating DNA precipitation methods. Generally, efficiency refers to the ability of a method to recover DNA from a sample, while yield indicates the quantity of DNA obtained after the process.
In traditional techniques, efficiency may not be as high, especially when dealing with extremely diluted or degraded samples. For example, when isolating DNA from processed samples or certain tissues, some DNA might remain soluble and not precipitate efficiently, leading to loss. In contrast, modern processes have shown significantly improved efficiencies, particularly with their ability to adapt to different sample conditions.
According to various studies,
- Traditional Techniques:
- Modern Techniques:
- Yields can range around 50-70% of available DNA.
- Efficiency is low in challenging samples, often needing multiple rounds for adequate recovery.
- Yields often exceed 90%, particularly for high-quality samples.
- Efficiency remains consistently high across different sample types including those that are difficult to process.
Ultimately, the selection of method influences not just the yield but also the downstream applications of the DNA. Ensuring that the chosen technique provides the best fit for the research or diagnostic purpose is fundamental for success in any molecular biology study.
Future of DNA Precipitation Techniques
The future of DNA precipitation techniques holds significant importance in advancing molecular biology and genetics research. As laboratories around the world seek to enhance the efficiency, reproducibility, and scalability of DNA extraction and purification processes, new technologies and methodologies are emerging. This evolution is not just about meeting existing demands but also about addressing potential limitations of current protocols.
Emerging Technologies
Among the new technologies, developers are focusing on enhancing automation and increasing adaptability in the laboratory environment. Technologies such as microfluidics are gaining traction. This approach allows researchers to conduct reactions in miniature channels with reduced reagent use, which can bolster both efficiency and accuracy. Additionally, advancements in bioinformatics and machine learning are being integrated into the design of precipitation methods. They can potentially predict optimal conditions for DNA recovery, making protocols more customizable to specific samples.
Moreover, novel materials like graphene-based formulations and other nanomaterials are being studied. These can improve selective capture and recovery of DNA during precipitation, making the process less reliant on traditional reagents, which can vary in quality.
Another noteworthy innovation is the use of magnetic beads for precipitation. This technique simplifies the separation of DNA from contaminants, making it easier to handle smaller sample volumes while achieving higher purity and yield.
Potential Improvements in Protocols
Protocols for DNA precipitation continue to evolve. Efforts are underway to simplify and streamline these methods. For instance, techniques aimed at minimizing the number of steps in DNA extraction are becoming more commonplace. This could have the potential to reduce the time and resources spent in laboratories.
There is also a strong focus on developing optimized reagents that can function across diverse types of samples and conditions. Increasing the robustness of protocols may lead to standardized results regardless of environmental variations, such as different reagent qualities or sample characteristics.
In addition, improving the accessibility of protocols through user-friendly kits is a growing trend. These kits may involve pre-measured reagents and clear instructions, helping to democratize access to quality research outputs across a variety of laboratory settings—ranging from academic institutions to biotech firms.
"The key to future developments in DNA precipitation will be focusing on efficiency, simplicity, and adaptability to meet the needs of diverse research scenarios."
As these advances unfold, it is essential for researchers and professionals in the field to stay informed about the new tools and techniques in DNA precipitation. This vigilance will ensure they can leverage changes that can positively impact their work and research outcomes.