Understanding Neuromodulators: Definition and Function
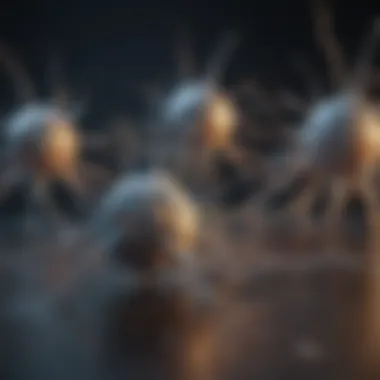
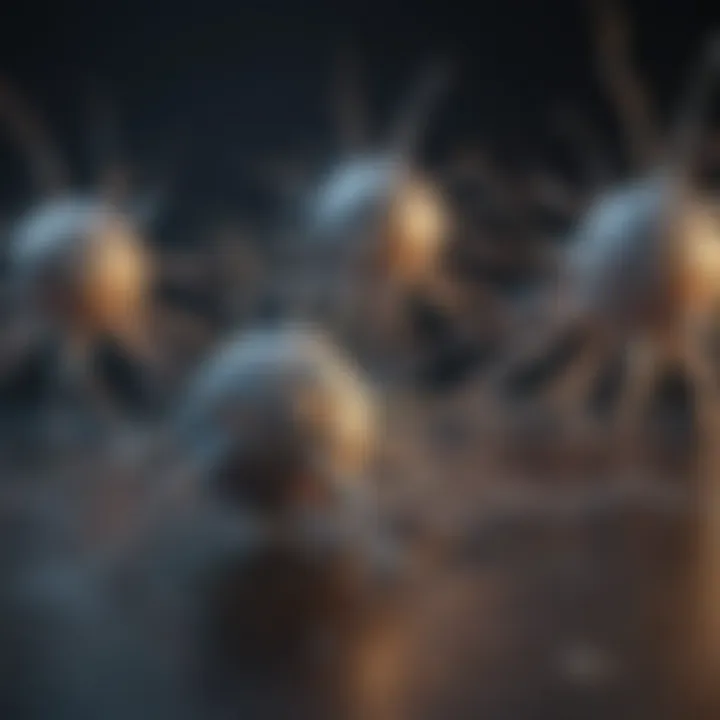
Intro
Neuromodulators are essential components of the nervous system. They play a crucial role in modulating the activities of neurons. Understanding their function is vital for various fields, including neuroscience, psychology, and medicine. This article aims to provide a comprehensive overview of neuromodulators, exploring their definitions, functions, and implications.
The concept of neuromodulation encompasses a wide range of biochemical substances. These substances do not merely transmit signals like neurotransmitters. Instead, they influence how neurons communicate and process information. Neuromodulators can enhance or inhibit synaptic transmission, thereby affecting overall neuronal activity.
In this narrative, we will examine different types of neuromodulators, their mechanisms of action, and the significance of their role in both health and disease. We will also discuss potential therapeutic applications, emphasizing the impact of these molecules on various physiological processes. Understanding the dynamics of neuromodulation can inform further research and clinical practices, making this topic increasingly relevant today.
Definition of Neuromodulators
Understanding neuromodulators is essential for comprehending the intricate workings of our nervous system. Neuromodulators are substances that modulate the activity of neural circuits, influencing how neurons communicate with each other. This definition underscores their critical function in neural communication and overall brain function.
Basic definition
A neuromodulator can be defined as a type of chemical messenger that influences a group of neurons, altering their activity without directly triggering a response. This interaction occurs when these substances bind to receptors on target neurons, leading to changes in the strength or efficacy of synaptic transmission. Unlike classical neurotransmitters, which generally exert rapid effects and operate on a point-to-point basis, neuromodulators have broader impacts and can affect multiple neurons across larger areas.
This basic definition is foundational as it sets the stage for exploring the diverse roles of neuromodulators, their types, and mechanisms of action. It is critical for anyone studying neuroscience or psychology to grasp this fundamental aspect, as it has significant implications for both health and disease.
Distinction from neurotransmitters
To fully appreciate neuromodulators, one must understand how they differ from neurotransmitters. Neurotransmitters such as dopamine, serotonin, and glutamate typically act at synapses, delivering fast signals that lead to immediate reactions. In contrast, neuromodulators do not adhere strictly to this model. They can modulate neuronal excitability and synaptic transmission over longer time frames and with less targeted action.
For instance, while a neurotransmitter may prompt a muscle contraction directly, a neuromodulator might adjust the overall responsiveness of the motor neurons involved. This distinction highlights the role of neuromodulators in regulating the dynamics of neural networks and their potential to work in tandem with neurotransmitters.
Role in the nervous system
Neuromodulators play a multifaceted role in the nervous system, affecting various physiological and psychological processes. They participate in the regulation of mood, arousal, motivation, and learning, illustrating their essential position within the brain's operational framework. Notably, these substances can have both excitatory and inhibitory effects, which adds to the complexity of their function.
For instance, in response to stress, the release of norepinephrine can enhance alertness and focus, while endorphins may provide pain relief and promote feelings of well-being. Understanding this duality is paramount, as the balance of these modulations can determine mental health outcomes and influence behavioral responses.
The neuromodulatory effects also extend to the realm of learning. They can help encode experiences more effectively by adjusting the strength of synapses. Consequently, this dynamic mechanism facilitates synaptic plasticity, which is crucial for memory formation and learning.
In summary, neuromodulators serve as vital players in the network of chemical communication within the nervous system. Their influence permeates numerous functions, emphasizing the importance of understanding their definition, differences with neurotransmitters, and roles in the complex interaction of neural pathways.
Types of Neuromodulators
The study of neuromodulators is essential for understanding how communication within the nervous system can be regulated and altered. This section details the various types of neuromodulators, which can be classified into three primary categories: classical, peptide, and gas neuromodulators. Each of these types plays a unique role in neural signaling, influencing several aspects of brain function and behavior.
Classical neuromodulators
Classical neuromodulators are small molecules that have a broad impact on neural activity. They include substances like dopamine, norepinephrine, serotonin, and acetylcholine. These neuromodulators typically function through synapses, where they can inhibit or stimulate neuronal activity by binding to specific receptors on target cells. This binding can result in various physiological outcomes, such as regulating mood, enhancing cognitive functions, and modulating pain perception.
Significantly, classical neuromodulators often work in tandem with neurotransmitters, enhancing or modifying their effects. For instance, dopamine is crucial for reward pathways, which influences both motivation and Pleasure. On the other hand, serotonin, often linked to mood stabilization, can impact sleep patterns and appetite. Their multifaceted roles highlight their importance in neuroscience and mental health research.
Peptide neuromodulators
Peptide neuromodulators differ from classical ones due to their larger structure and complex chemical nature. Examples include endorphins, oxytocin, and neuropeptide Y. Their synthesis occurs in neurons and requires a more extended process than classical neuromodulators.
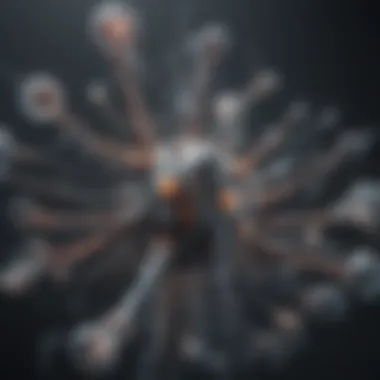
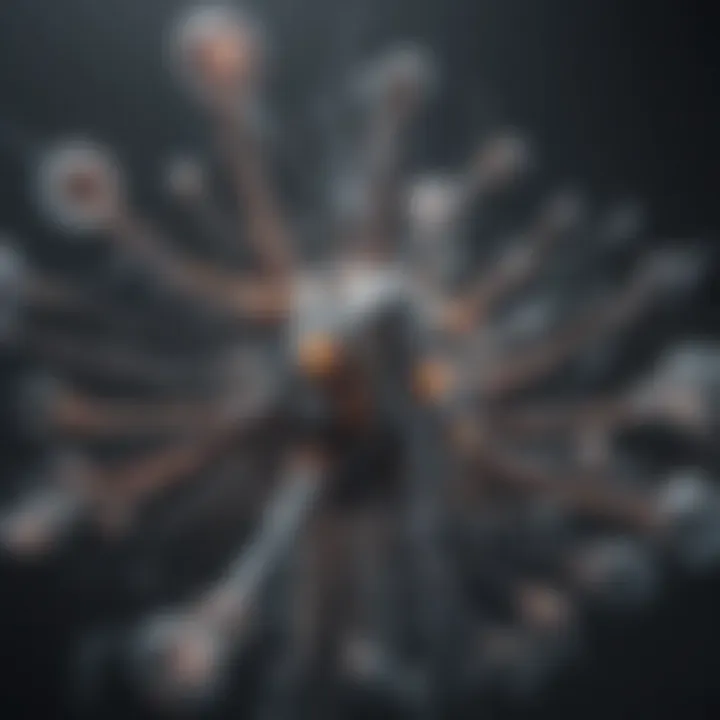
The effects of peptide neuromodulators are generally slower in onset but tend to be more prolonged. For instance, oxytocin is known for its role in social bonding and trust. In contrast, endorphins are often referred to as the body's natural painkillers, highlighting their relevance in pain modulation and emotional well-being. Researchers continue to explore peptide neuromodulators for their therapeutic potential, especially in understanding conditions like depression and anxiety.
Gas neuromodulators
Gas neuromodulators represent a unique class, including nitric oxide and carbon monoxide. Unlike other types, they can diffuse through cell membranes without the need for specific receptors. This characteristic allows gas neuromodulators to affect neighboring neurons and even influence blood flow in the brain. The rapid responsiveness of gas neuromodulators can lead to immediate effects on synaptic plasticity and neuronal signaling.
For example, nitric oxide is vital in regulating vascular function and neurotransmission. Its role in synaptic plasticity is essential for learning and memory. Researchers are keen to further unravel the complexities surrounding gas neuromodulators, particularly in terms of their potential implications in neurodegenerative diseases.
Understanding these three types of neuromodulators is critical for appreciating how the brain processes information and responds to stimuli. Each type offers different benefits and possesses considerable implications for research and therapeutic strategies in neuroscience and medicine.
Mechanisms of Action
Understanding the mechanisms of action of neuromodulators is crucial in comprehending how they affect the nervous system. Neuromodulators play a pivotal role through various methods of interaction and signaling processes. This section explores how these substances influence neural activity. Emphasis will be placed on receptor interactions, signal transduction pathways, and their effect on synaptic plasticity.
Receptor interactions
Neuromodulators primarily exert their effects through receptor interactions. These interactions can modify neuronal signaling, often without directly causing an excitatory or inhibitory response themselves. Instead, neuromodulators alter how neurons respond to other neurotransmitters.
For instance, dopamine acts on specific receptors, leading to changes in mood and motivation. Various types of receptors, including G-protein coupled receptors and ion channels, mediate these effects. By binding to receptors, neuromodulators can influence the overall excitability of neurons, shaping physiological outcomes such as emotional states and cognitive processes.
Signal transduction pathways
Once a neuromodulator binds to a receptor, it triggers complex intracellular processes known as signal transduction pathways. These pathways transmit signals from the receptor to the inside of the cell, often resulting in functional changes.
Key signaling molecules, like second messengers such as cyclic AMP (cAMP) and calcium ions, play a critical role here. These compounds initiate a cascade of reactions that can lead to gene expression changes, protein synthesis, and even structural changes in the neuron. Such changes can have lasting impacts, further underscoring the importance of these pathways in neuromodulation.
Neuromodulators can have widespread effects beyond the immediate area of receptor binding, influencing distant neurons and circuits.
Influence on synaptic plasticity
Additionally, neuromodulators significantly affect synaptic plasticity—the ability of synapses to strengthen or weaken over time. This plasticity underlies learning and memory processes, as certain neuromodulators can facilitate synaptic changes associated with these functions.
For instance, serotonin has been linked to long-term potentiation (LTP), which enhances synaptic transmission. Conversely, other neuromodulators may promote long-term depression (LTD), reducing synaptic efficacy. Understanding these dynamics is essential, as they provide insights into how experiences shape neuronal connections and, ultimately, behavior.
Physiological Effects
The physiological effects of neuromodulators are fundamental to understanding their importance in the nervous system. Neuromodulators impact various functions within the brain and body, influencing mood, memory, and pain perception. By examining these effects, we can better appreciate the mechanisms through which neuromodulators contribute to both health and disease.
Regulation of mood and behavior
Neuromodulators play a critical role in the regulation of mood and behavior. Chemical substances such as serotonin, dopamine, and norepinephrine serve as key neuromodulators that influence emotional states.
- Serotonin: This neuromodulator is often linked to feelings of well-being. Low levels can lead to disorders such as depression or anxiety.
- Dopamine: Associated with pleasure and reward, dopamine levels can substantially impact motivation and addiction.
- Norepinephrine: It affects alertness and arousal, playing a role in attention and focus.
Researchers suggest that imbalances in these neuromodulators can lead to mood disorders, making them crucial targets for therapeutic interventions. By understanding neuromodulation’s effects on mood, they are exploring new treatment strategies for mental health conditions.
Impact on learning and memory
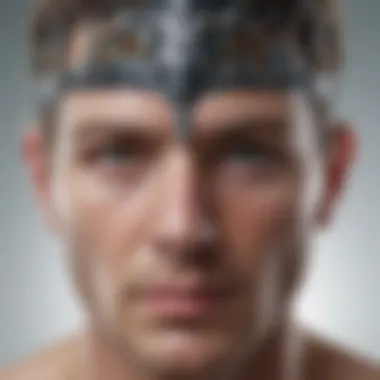
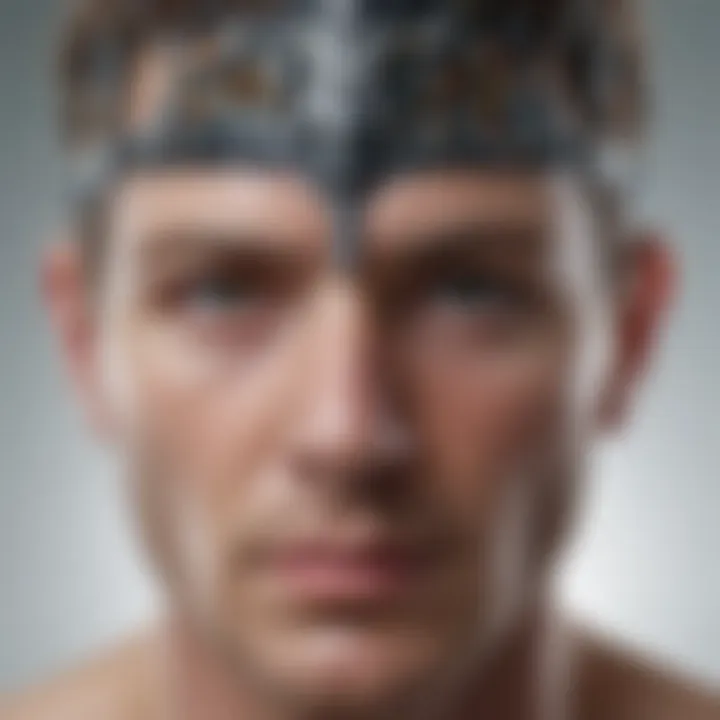
Neuromodulators significantly affect learning and memory processes. These substances modulate synaptic plasticity, which is the ability of synapses to strengthen or weaken over time. For instance:
- Acetylcholine: This neuromodulator is essential for attention and memory formation. It is involved in enhancing the encoding of information.
- Glutamate: As a primary excitatory neurotransmitter, it plays a crucial role in long-term potentiation, a mechanism underlying learning and memory.
Studies indicate that effective neuromodulation can improve cognitive functions, suggesting that therapies targeting these pathways could enhance learning capabilities or address cognitive impairments in various conditions.
Role in pain modulation
Neuromodulators are also pivotal in pain modulation. They help in processing and perceiving pain signals in the nervous system. Several key neuromodulators have been identified in this context:
- Endorphins: These are natural pain relief compounds, operating similarly to opioids. They reduce the perception of pain and can induce feelings of euphoria.
- Substance P: In contrast, Substance P is associated with the transmission of pain signals, thus playing a dual role in the pain experience.
Understanding how neuromodulators influence pain can lead to the development of better pain management strategies. For instance, targeting specific neuromodulatory pathways may lead to new treatments for chronic pain syndromes.
Recent studies suggest neuromodulatory interventions may shift pain perception and improve quality of life for individuals suffering from chronic pain conditions.
In summary, the physiological effects of neuromodulators are vast, affecting mood, learning, and pain. This understanding contributes to the ongoing research and development of therapeutic applications aimed at improving mental health and pain management.
Neuromodulators and Disease
The relationship between neuromodulators and disease is critical in understanding both normal nervous system function and the pathophysiology of many conditions. Neuromodulators influence various neurological processes. This influence can often become disrupted in disease states. By studying these dynamics, researchers can gain insights into potential treatments and interventions. Neuromodulators can alter the course of diseases and highlight targets for therapeutic strategies. Thus, this section covers three main areas: neurological disorders, psychiatric conditions, and chronic pain syndromes. Each segment will explain how neuromodulators operate in these contexts, providing a more comprehensive understanding of their roles.
Neurological disorders
Neurological disorders such as Parkinson's disease, Alzheimer's disease, and multiple sclerosis are deeply influenced by neuromodulators. These disorders cause significant alterations in brain function, often linked to dysregulation of neuromodulatory systems. For instance, in Parkinson's disease, the depletion of dopamine affects motor function, while neuromodulator imbalances may also contribute to cognitive decline. Understanding the specific roles of neuromodulators, such as glutamate and GABA, is paramount.
- Dopamine: In diseases like Parkinson's, loss of dopamine leads to issues such as tremors and rigidity.
- Glutamate: Overactivity of glutamate can cause excitotoxicity, leading to neuronal damage in disorders like Alzheimer's.
- GABA: Low levels of GABA are associated with increased neuronal excitability, contributing to seizures and anxiety in certain disorders.
Effective treatment strategies often involve targeting these neuromodulating systems. This targeted approach can improve patient outcomes and enhance disease management.
Psychiatric conditions
Psychiatric conditions, including depression, anxiety disorders, and schizophrenia, also show significant associations with neuromodulation. Imbalances in neurotransmitters, which are often influenced by neuromodulators, are critical in these conditions. For instance, serotonin and dopamine levels are heavily modulated by various factors, often representing the underlying causes of mood disorders.
- Serotonin: Its modulation is essential in treating depression and anxiety. Selective serotonin reuptake inhibitors (SSRIs) are standard in managing these disorders.
- Dopamine: Abnormalities in dopamine levels are linked to schizophrenia, making it a target for antipsychotic medications.
- Norepinephrine: This modulator plays a role in the stress response and affects mood stability in various conditions.
The understanding of how neuromodulators function can lead to novel therapeutic approaches. Psychotherapies and medications can be adjusted based on neuromodulatory pathways, offering more personalized treatment options.
Chronic pain syndromes
Chronic pain syndromes, such as fibromyalgia and neuropathic pain, exhibit complex interactions with neuromodulators. Neuromodulators regulate pain pathways and can either enhance or inhibit pain perception. For instance, norepinephrine and serotonin are crucial in how pain is perceived in the brain.
- Substance P: Increases pain perception. Its modulation is significant in alleviating chronic pain symptoms.
- Endorphins: These natural painkillers can modulate the perception of pain. Their enhancement can lead to better pain management strategies.
- Cannabinoids: This modulator pathway shows promise in treating various chronic pain conditions.
Studying the role of neuromodulators in pain syndromes can highlight new approaches to treating chronic pain. Identifying how these modulators function can contribute to better pain management strategies.
"Neuromodulators play a pivotal role in disease dynamics, offering insights into both pathology and potential therapy."
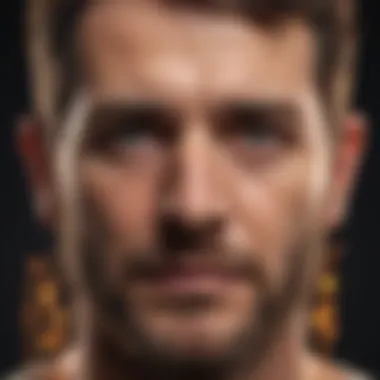
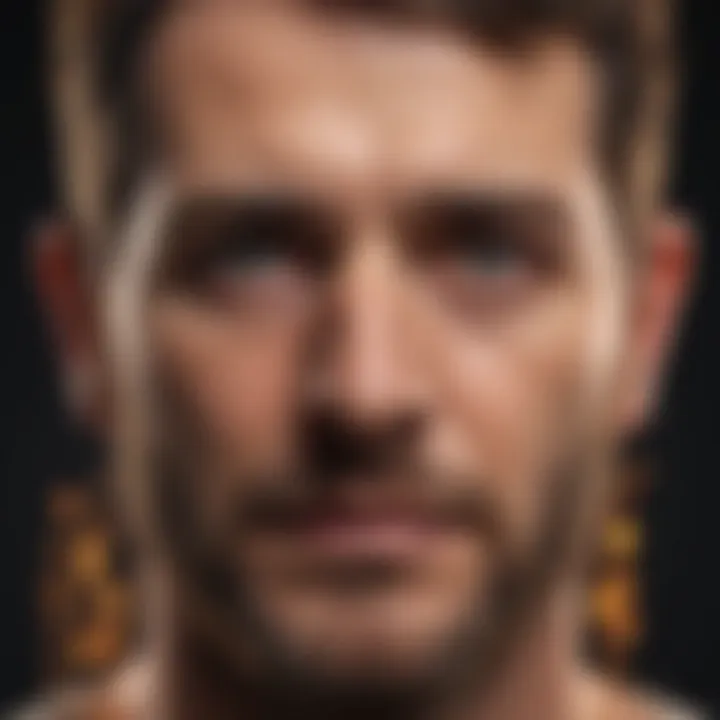
In essence, neuromodulators are deeply intertwined with various diseases, presenting opportunities for advancing understanding in therapeutic applications.
Therapeutic Applications
Therapeutic applications of neuromodulators represent a critical area of exploration within neuroscience and medicine. Understanding how these chemicals influence neuronal function can guide the development of innovative strategies to treat various disorders. Neuromodulators offer potential benefits due to their ability to adjust the activity of neurotransmitters and modify synaptic efficacy. The therapeutic significance lies in their capacity to ameliorate symptoms and address underlying mechanisms of disease.
Drug development
The process of drug development involving neuromodulators is gaining momentum. Neuromodulators can lead to new pharmacological agents that target specific pathways within the brain. This process typically begins with preclinical research, where potential compounds are evaluated for their modulatory effects on neural circuits. Established medications like lithium and certain antidepressants illustrate the successful utilization of neuromodulators in clinical settings. However, challenges persist in identifying selective neuromodulator targets while minimizing side effects.
Neuromodulator-based therapies
Neuromodulator-based therapies encompass a wide range of treatments that aim to restore normal function in the nervous system. Examples include deep brain stimulation and transcranial magnetic stimulation, both of which leverage the principles of neuromodulation. These methods can alleviate symptoms in conditions such as depression, epilepsy, and Parkinson's disease. Such therapies are not without limitations; customization for individual patients is essential for optimizing outcomes. Recent studies show promising results in combining neuromodulatory therapies with cognitive behavioral approaches, which may enhance treatment efficacy.
Future of neuromodulation in medicine
Looking ahead, the future of neuromodulation in medicine appears promising. Continued research into the specific roles and mechanisms of various neuromodulators will lead to more targeted approaches. The integration of technology, such as brain-computer interfaces, could enhance the precision of neuromodulatory therapies. With the recognition of individual variability in treatment response, personalized medicine is also becoming an essential aspect of future applications. The ongoing exploration in this dynamic field has the potential to transform our understanding of brain function and revolutionize therapeutic interventions.
"The integration of neuromodulators in treatment opens new avenues in clinical practice, providing targeted approaches to complex neuropsychiatric disorders."
In summary, the therapeutic applications of neuromodulators highlight their importance in advancing both our understanding of the nervous system and the treatment of various diseases. As research progresses, the potential benefits of these applications will continue to expand, reinforcing the need for a nuanced approach in both research and clinical practice.
Research Frontiers
Research into neuromodulators is crucial for various reasons. It opens new avenues for understanding the intricacies of the nervous system. Also, it sheds light on the ways these chemical messengers influence behavior, cognition, and emotional regulation. Ongoing studies focus on how neuromodulators can be harnessed to develop new treatments for neurological and psychiatric disorders. This field holds promise for enhancing therapeutic options, thus improving quality of life for many individuals.
Recent findings in neuromodulation
Recent studies have uncovered significant insights into the specific roles neuromodulators play in brain function. For instance, emerging research reveals how serotonin influences mood regulation during emotional stimuli. Other studies demonstrate that dopamine is critical in reward processing and motivation. These findings not only deepen our understanding of mental health but also raise new questions about potential treatment targets. Understanding the variability in neuromodulator levels among individuals may explain different responses to therapies, suggesting a more personalized approach could be beneficial.
Technological advancements in research
Technological advancements significantly impact the field of neuromodulation research. Novel imaging techniques, such as functional magnetic resonance imaging (fMRI), allow researchers to visualize neuromodulator activity in real time. This enhances our capability to study dynamic brain processes. Furthermore, optogenetics provides researchers with tools to manipulate neuromodulator systems with precision. Such advancements not only facilitate exploration of fundamental principles but also help in the development of innovative therapeutic strategies. They make it possible to explore how specific circuits in the brain respond to various stimuli influenced by neuromodulators.
Challenges in neuromodulator research
Despite the advancements, neuromodulator research faces numerous challenges. One primary issue is the complexity of the signaling pathways. Neuromodulators often interact with various receptor types, leading to different outcomes depending on the context. This complexity makes it difficult to develop targeted therapies. Additionally, the ethical considerations in studying these substances, especially in human subjects, pose significant hurdles. Research funding limitations can also restrict the scope of studies undertaken. Overcoming these challenges is vital for translating research findings into effective clinical applications.
"Advancements in neuromodulation research will not only enhance understanding of the brain but also revolutionize therapies available for mental health disorders."
Enhanced awareness of these research frontiers can guide future investigations into neuromodulation. Thus, addressing existing challenges and leveraging new technologies is essential for unlocking the full potential of neuromodulators in both health and disease.
Closure
The conclusion of this article emphasizes the integral role of neuromodulators within the nervous system. Neuromodulators are not merely facilitators of communication; they shape the very framework of neuronal interactions and influence both physiological and psychological processes. Understanding their functions provides critical insights into mental health disorders and other biological conditions. This knowledge can lead to more tailored therapeutic approaches, optimizing patient care and outcomes.
Summary of key points
In summary, the following key points about neuromodulators have been discussed:
- Definition: Neuromodulators are substances that alter neuronal activity and influence synaptic transmissions, distinct from neurotransmitters.
- Types: Various types of neuromodulators, including classical, peptide, and gas neuromodulators, each serve unique functions in the nervous system.
- Mechanisms: Their action involves receptor interactions, signal transduction pathways, and synaptic plasticity regulation.
- Physiological effects: Neuromodulators affect mood, learning, memory, and pain modulation.
- Relevance in disease: They play significant roles in neurological and psychiatric disorders and chronic pain syndromes.
- Therapeutic applications: Emerging strategies in drug development leverage insights about neuromodulators to create innovative treatment methodologies.
- Research frontiers: Ongoing studies are unveiling complex interactions and technological advancements aimed at understanding neuromodulation better.
Implications for the future
Neuromodulation holds vast potential for the future of medicine and psychological health. Understanding these molecules can guide the development of targeted therapies that address specific dysfunctions in the nervous system. As research progresses, there is a promise of personalized neuromodulator therapies that could revolutionize treatment paradigms. Moreover, advances in technology will likely enhance our comprehension of how these substances operate at finer levels, contributing to a more profound understanding of human behavior and physiology. Furthermore, recognizing the intricate balance neuromodulators maintain can inform preventive strategies for various disorders, ultimately leading to healthier outcomes and improved quality of life.