Understanding Optical Thinness: Principles and Uses
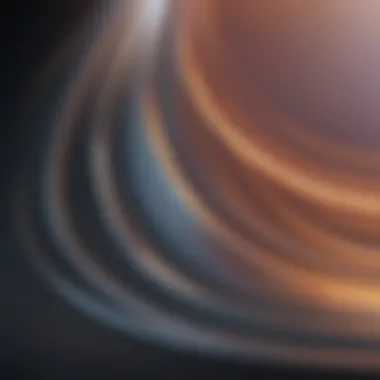
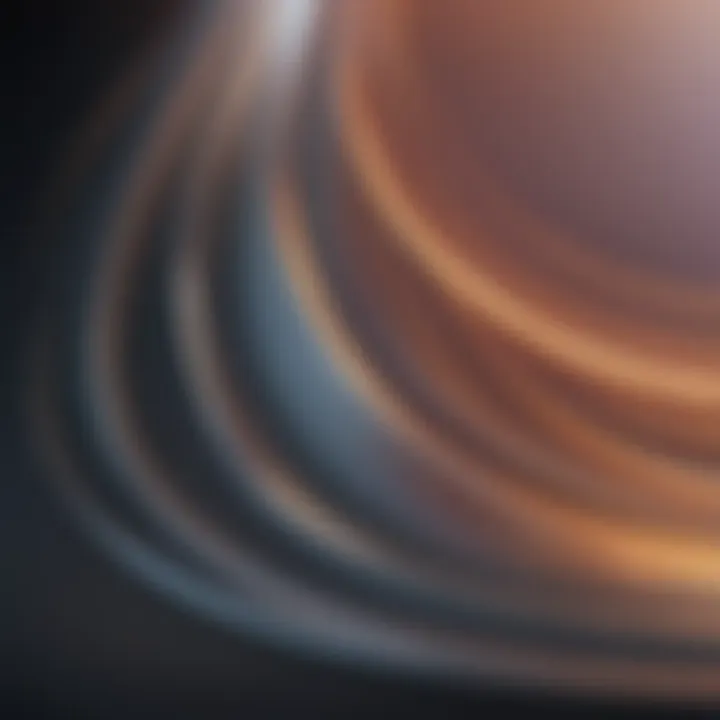
Intro
Optical thinness is a pivotal concept in the realm of optics, serving as both a theoretical underpinning and a gateway to various practical applications. At its core, this notion addresses how light interacts with materials that are, quite literally, thinner than its wavelength. It’s not just a niche topic; understanding optical thinness opens up new avenues in fields like engineering, material science, and even biological systems.
The role that optical thin films play in technology is monumental. These layers, often only a fraction of a wavelength thick, enable a wide range of functionalities—from anti-reflective coatings on glasses to the vibrant colors observed on soap bubbles. Moreover, the advances in nanotechnology have honed our ability to manipulate these thin films with remarkable precision, pushing the boundaries of what is possible in optics.
As we push forward, challenges abound. Researchers are grappling with ensuring the stability of these films under various conditions and exploring the limits of their adaptability. The lines between fundamental science and industrial application are increasingly blurry, sparking a dialogue on how best to bridge this gap.
In this exploration, we aim not only to dissect the principles underpinning optical thinness but also to elucidate the applications that spring from it. With an eye toward the future, this narrative will bring to light the significance of continued research in this field, while making the technical details accessible to both specialists and curious minds alike.
With these considerations in mind, let's turn to the key findings that illuminate the path forward.
Preamble to Optical Thinness
Understanding optical thinness is paramount in today’s scientific and technological landscape. It plays a crucial role in areas ranging from photonics to materials science. At its core, optical thinness refers to the behavior of light as it interacts with materials that are so thin that light passes through with minimal scattering and absorption. Highlighting this concept allows us to appreciate the subtleties involved in light manipulation, which has become increasingly important in the design of optical devices and systems.
A key aspect of optical thinness is optical thickness. This is a measure of the opacity of a film or medium, dictating how much light is transmitted versus reflected. In practical applications, this principle becomes significant in fields like telecommunications, where ensuring adequate light transmission can affect signal clarity.
Defining Optical Thickness
Optical thickness, contrary to physical thickness, concerns itself with the interaction of light with materials based on wavelength and material properties. Generally, it is defined mathematically as the product of the physical thickness of the layer and the refractive index of the medium through which light travels. For instance, a 500 nm thick film made of a material with a refractive index of 1.5 has an optical thickness of 750 nm.
The refractive index significantly influences optical behavior; materials with high refractive indices can lead to a greater deviation of light. In applications such as anti-reflective coatings, manipulating the refractive index within optical thickness calculations can lead to considerable enhancements in light transmission.
Historical Context and Development
To truly understand optical thinness, we must look at its historical framework. Earlier scientific inquiries into light were dominated by the likes of Newton with his particle theory, and Huygens, who advocated for waves. These differing perspectives laid the groundwork for later interpretations and experiments.
As scientific instruments advanced, researchers began to observe phenomena such as thin film interference. This phenomenon occurs when light waves reflected from the surface of thin films combine, producing vibrant colors. Such observations, notably analyzed by Thomas Young in the early 19th century, paved the way for foundational theories in optics.
The 20th century brought forth modern techniques to manipulate and measure optical thickness. With the development of lasers and other coherent light sources, industries began to apply these concepts widely, leading to innovations in mobile communication, data storage, and energy systems. Understanding the nuances of optical thinness continues to be a point of research and innovation, reaffirming its importance across multiple disciplines.
"Optical thinness is not just a concept; it's a fundamental principle that shapes our understanding of light and its applications in technology."
Grasping the foundational principles surrounding optical thinness prepares the ground for deeper discussions in the subsequent sections of this article, where we will explore the theoretical frameworks, measurement techniques, and real-world applications that make this topic both relevant and critical for future advancements.
Theoretical Framework
Understanding the theoretical framework behind optical thinness is like laying the groundwork for constructing a complex building; it gives structure to our inquiry into how light interacts with matter. This section elucidates the fundamental principles, the dichotomy between wave and particle perspectives, and the mathematical modeling that attempts to quantify these interactions. Not only does grasping these concepts enhance our comprehension of optical thinness, but it also paves the way for future innovations in various technological domains.
Fundamental Principles of Light-Matter Interaction
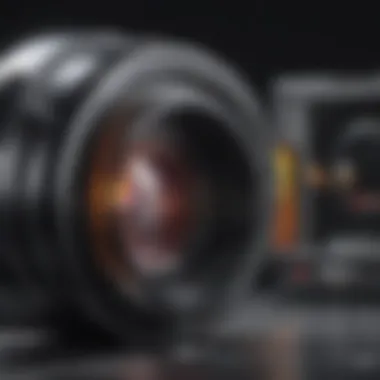
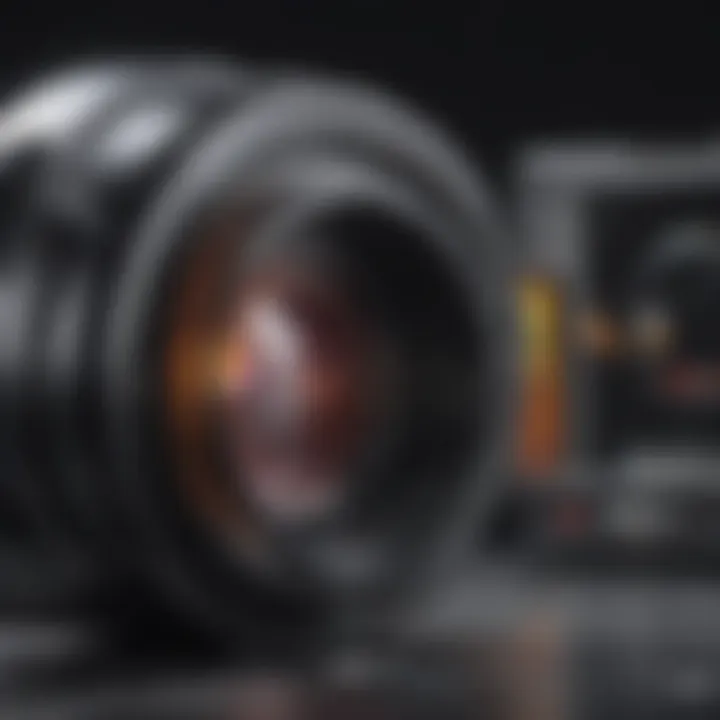
Light interaction with material systems is at the heart of optical thinness. When light strikes a thin layer—be it a coating or film—it can be reflected, absorbed, or transmitted. The way it behaves is determined by several factors:
- Material Properties: Different materials have distinct refractive indices, which significantly affect how light traverses them. For instance, a layer of titanium dioxide will interact with light quite differently than a layer of silica.
- Film Thickness: The thickness of the film often determines which wavelengths of light are enhanced or diminished through constructive or destructive interference. Think of a musical instrument with strings of varying lengths producing different notes; optical thin films resonate similarly with light waves.
- Angle of Incidence: The angle at which light hits the surface can change the way it is scattered. A right angle may yield different outcomes than an oblique one.
These interactions collectively dictate the effectiveness of optical thin films in a variety of applications, from anti-reflective coatings on glasses to filters used in cameras.
Wave and Particle Interpretations
In the realm of optics, the dual nature of light as both a wave and a particle often generates discussions that feel like two sides of the same coin. Light as waves offers insights into phenomena such as interference and diffraction, where light bends around corners or creates patterns based on film thickness. For instance:
- Interference can create vibrant colors in soap bubbles or oil slicks, an outcome of varying film thickness leading to different path lengths for light waves.
In contrast, the particle aspect of light encapsulated in photons allows for the understanding of how light energy interacts with matter at a granular level. This is particularly crucial in the context of photoelectric effects seen in solar cells, where photons can free electrons from a material's grip. The wave-particle duality ultimately enriches our understanding of optical thinness by offering diverse lenses through which we can analyze light's behavior.
Mathematical Modeling of Optical Thickness
To quantify and predict how thin films will behave, mathematical modeling becomes essential. Several well-established models help researchers and practitioners simulate the behavior of light in thin films:
- Transfer-Matrix Method: This powerful tool lays out how light reflects and transmits through layered structures, accounting for multiple interfaces and interactions.
- Fresnel Equations: These equations allow for meticulous calculations of reflectance and transmittance at each interface based on the refractive indices of the involved materials.
- Rayleigh Scattering Theory: This model is particularly useful for understanding light scattering in thin layers and helps explain why the sky appears blue, among other phenomena.
These mathematical frameworks are more than abstract numbers; they serve practical applications in designing filters, lenses, and coatings essential in modern technology. To take it a step further, simulations based on these models help refine optical components tailored for specific purposes.
"Mathematical models are the unseen architects of the optical world, allowing us to foresee behaviors before they manifest."
A grasp of these theoretical underpinnings is vital for anyone venturing into the world of optical thinness, making the exploration not just an academic exercise but a necessary foundation for real-world applications.
Measurement Techniques
Understanding the concept of optical thinness is not complete without a keen look at measurement techniques. The precision in measuring optical thickness allows for advancements in practical applications, influencing areas such as photonic devices, surface engineering, and solar technologies. This section focuses on the core elements associated with measuring optical thin films, detailing both standard and innovative methods. The accuracy and consistency of these measurements enable researchers and professionals to effectively harness the benefits of optical thinness in their respective fields.
Standard Methods of Measurement
Standard methods of measurement provide foundational techniques that have been refined over years of research. These traditional approaches typically emphasize reproducibility and reliability, serving as a benchmark against which novel techniques are evaluated. Key methods include:
- Interferometry: A classic technique that exploits the wave nature of light, this method allows precise determination of thickness by analyzing interference patterns. By using coherent light sources, such as lasers, it measures the phase shift that occurs when light traverses a film. This shift translates directly to the optical thickness, making interferometry indispensable.
- Spectroscopic Ellipsometry: This technique utilizes polarized light to assess film thickness. By measuring the change in polarization upon reflection, it provides information about the optical properties of the thin film. It’s particularly valuable for materials where thickness is on the order of nanometers, resulting in high sensitivity and accuracy.
- Transmission and Reflection Spectroscopy: This method studies how light transmits through or reflects off thin films. By analyzing the intensities and wavelengths of transmitted or reflected light, one can infer the film's optical thickness and its refractive index.
While these techniques have proven effective, they come with considerations that need addressing. For instance, environmental factors and material properties can influence measurements, requiring meticulous calibration and control during experiments.
Innovative Approaches in Characterization
As technology progresses, new measurement techniques are emerging to complement traditional methods, helping researchers gain deeper insights into optical thinness. Some innovative approaches include:
- Nanotechnology-Based Sensors: Recent developments in nanotechnology have paved the way for sensors that can measure optical properties at the nanoscale. These sensors can provide real-time data and allow for measurements in situ, which is crucial for dynamic applications such as coatings in variable conditions.
- Plasmonic Biosensors: These sensors leverage surface plasmon resonance to achieve high sensitivity in determining film thickness. They are primarily used in biosensing applications, where detecting minor changes in thickness can indicate the presence of specific biomolecules.
- Optical Coherence Tomography (OCT): This non-invasive imaging technique delivers high-resolution cross-sectional images, enabling an in-depth analysis of optical properties in layers of thin films. By utilizing interference patterns, OCT can provide data that is essential for understanding multilayer thin films.
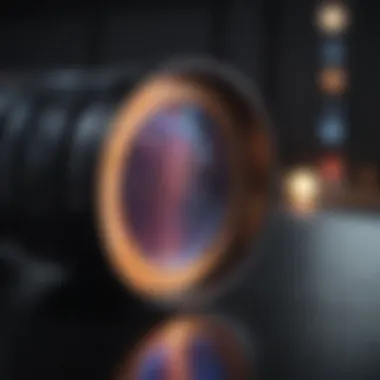
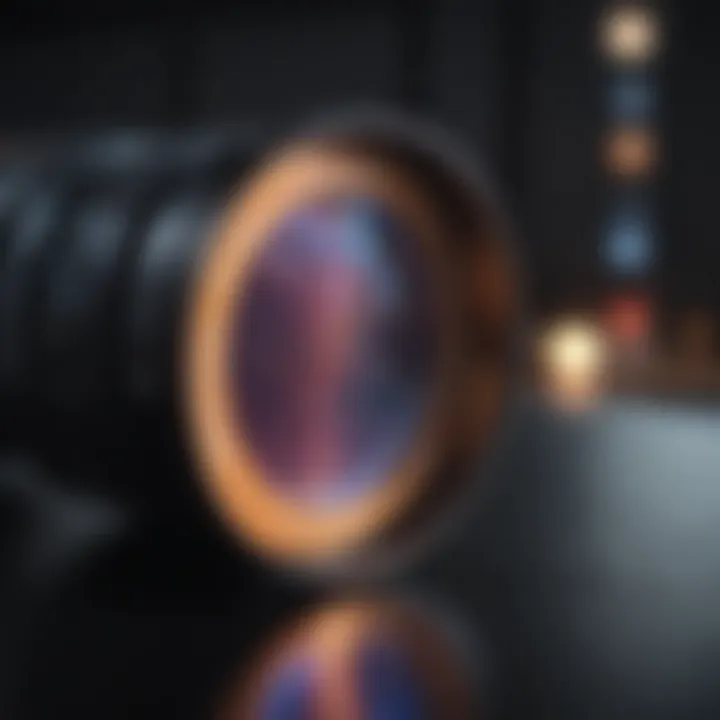
"Measurement techniques not only determine the properties of optical thin films but also drive innovation across technology applications."
Applications of Optical Thin Films
Optical thin films have found a foothold in numerous domains, influencing not only how we use technology today but also how we envision future innovations. These films, characterized by their ability to manipulate light with minimal physical thickness, serve as critical components in many devices, enhancing functionality while meeting certain design constraints. Their importance stems from a mix of properties including interference effects, reflection and transmission control, and the capability for tunable optical characteristics.
Role in Photonic Devices
Photonics has emerged as a field pivotal to modern communication, sensing, and imaging technologies. Optical thin films are integral to various photonic devices, playing a crucial role in the processing and management of light. For instance, these films can create optical filters that selectively transmit certain wavelengths while blocking others. Such capability proves essential in devices like laser systems, where maintaining a particular wavelength can be the difference between success and failure.
Moreover, thin-film coatings on lenses in cameras and microscopes are designed to minimize reflections. By using layers of materials with varying refractive indices, engineers can enhance light transmission and ensure that the captured images are clear and true to color.
In telecommunications, the application of thin-film technology enhances the performance of optical fibers, augmenting data transmission speeds. Researchers are also exploring ways to develop photonic devices that harness non-linear optical processes within thin films. The potential here is vast, from advanced signal processing to novel communication strategies.
Impact on Coatings and Surface Engineering
The influence of optical thin films extends significantly into coatings and surface engineering. Optical coatings, varying from anti-reflective to reflective layers, are fundamental in ensuring the safety and durability of surfaces in industries ranging from automotive to aerospace.
- Anti-Reflective Coatings: These coatings optimize the usability of display screens on smartphones and televisions. They minimize glare, improving visibility under different lighting conditions, enhancing user experience.
- Reflective Coatings: In mirrors and lenses, reflective coatings are crucial for maximizing light efficiency. For example, mirrors used in telescopes or other scientific instruments rely on thin films to ensure that as much light is gathered as possible, contributing to clearer and more detailed observations.
- Protective Coatings: Besides optical properties, optical thin films also provide protective qualities. They safeguard electronic components and solar panels from environmental hazards such as moisture and particulate matter, thus extending the lifetime and reliability of critical devices.
"The role of thin-film technology in coatings bridges the gap between optical performance and practical engineering, leading to innovations that reshape industries."
Significance in Solar Technologies
In the quest for sustainable energy, optical thin films play a transformative role in solar technologies. The efficiency of photovoltaic cells can be drastically improved through the use of thin films that enhance light absorption and minimize reflection losses.
For instance, anti-reflective coatings are paramount in solar panels, allowing more sunlight to penetrate the cell structure, thus generating more electricity. Additionally, the development of multifunctional thin-film coatings is paving the way for innovative solar solutions. These films can filter UV rays, reduce heat build-up, and protect against weather impacts, leading to prolonged operational lifespans for solar panels.
Also, research into tandem solar cells, where multiple layers of different thin-film materials work together, has shown promising results in pushing efficiency boundaries higher than traditional single-junction panels. This underscores the value of optical thin films, not just as passive components, but as active participants in advancing solar energy technology.
Challenges in Understanding Optical Thinness
In the realm of optical thinness, challenges abound that can trip up even seasoned experts. This topic deserves meticulous attention as it uncovers the hidden intricacies and nuances that stand in the way of fully grasping how optical thin films operate. Understanding these challenges not only enriches our comprehension of optical thinness but also paves the way for innovation and refinement within this field.
Limitations in Existing Models
Existing theoretical models of optical thinness, while providing a valuable starting point, often fall short in capturing the multifaceted behaviors exhibited by various materials. One prominent limitation is the reliance on the wave or particle duality of light under specific conditions, neglecting how these aspects coalesce in practical scenarios.
- Simplistic Assumptions: Many models assume that materials behave uniformly. In reality, the optical properties can change depending on various intrinsic and extrinsic factors. For instance, variations in temperature or humidity can subtly alter how light interacts with a film, leading to discrepancies between predicted and observed behaviors.
- Neglect of Nonlinear Effects: Current frameworks often overlook nonlinear optical effects, which come into play at high intensities. This can result in misleading calculations for applications, particularly in photonics and telecommunications, where precision is crucial.
- Limited Scope of Materials: Most existing models are developed with a narrow focus, often omitting a range of materials that can exhibit unique optical responses. As materials science advances, there’s an increasing necessity to integrate unconventional materials into our understanding of optical thinness.
These limitations highlight the necessity for a more robust theoretical framework that factors in these complexities. By acknowledging the shortcomings of existing models, researchers can better pursue innovative solutions and refine their approaches.
Environmental Influences on Measurements
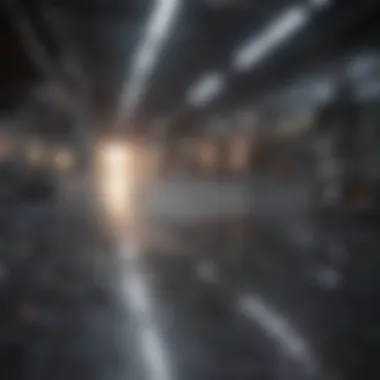
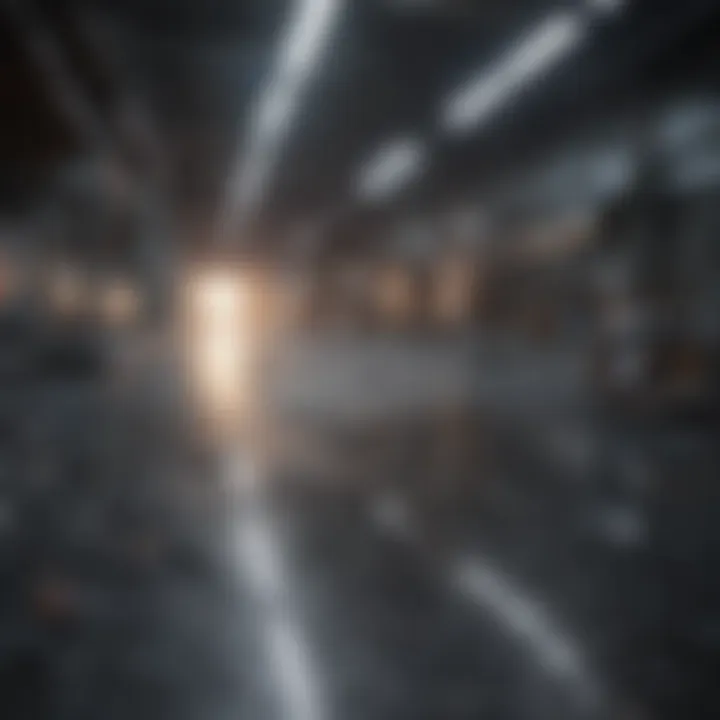
The measurement of optical thickness is not immune to the whims of its environment. Numerous external factors can skew results, presenting challenges that researchers must navigate carefully.
- Atmospheric Conditions: Variations in temperature and pressure can cause significant fluctuations in measurement accuracy. For example, high humidity can lead to the condensation of moisture on surfaces, affecting how light interacts with the film. This necessitates careful control and consideration of environmental conditions before conducting experiments.
- Pollution and Contaminants: Dust and chemical pollutants can form thin films on optical surfaces, altering their intended properties. These unexpected contaminants might lead to erroneous interpretations, ultimately affecting the performance of devices that depend on precise optical characteristics.
- Measurement Techniques: Different techniques employed to gauge optical thickness can yield varied results based on their underlying principles and operational contexts. Selecting an inappropriate method can introduce substantial errors into the data being evaluated, further complicating the challenge at hand.
The interplay of environmental factors with measurement techniques is a delicate balance. Establishing standardized protocols can mitigate some of these influences, allowing for more consistent and reliable data, although it may not be wholly feasible in all scenarios.
"Understanding the challenges tied to optical thinness is instrumental in unlocking its potential across varied applications."
Future Directions in Optical Thin Research
The exploration of optical thinness is poised on the brink of groundbreaking advancements. As we inch deeper into the 21st century, the importance of this topic cannot be overstated. Optical thin films are not just passive layers in optical systems; they are active participants in the evolution of technology across various fields. Understanding future directions in optical thin research holds the key to unlocking innovations that could reshape industries such as telecommunications, medicine, and renewable energy.
Emerging Technologies and Innovations
The interplay between optics and technology is fostering an era of innovation that is not to be ignored. Recent developments in nanotechnology are paving the way for the creation of ultra-thin films with unique optical properties. One noteworthy area is plasmonics, where light interacts with metal nanoparticles, giving rise to enhanced light absorption and transmission. Such advancements are setting the stage for new applications in sensors, imaging systems, and energy harvesting devices.
- Emerging technologies can revolutionize many sectors, including:
- Telecommunications: Ultra-thin optical films are being integrated into communication networks for better signal transmission, minimizing losses over long distances.
- Renewable Energy: The integration of optical thin films in solar cells is crucial for enhancing light absorption, leading to higher energy conversion efficiencies.
- Biomedical Applications: Optical coatings are being utilized for advanced imaging techniques, improving diagnostic capabilities in healthcare.
*Moreover, the use of computation to predict the behavior of these films is becoming standard. These predictive models can lead to the optimization of films before they are even synthesized. As technologies continue to emerge, interdisciplinary collaboration becomes necessary for effectively implementing these innovations.
Interdisciplinary Approaches
To truly harness the potential of optical thinness, an interdisciplinary approach is essential. Bringing together physicists, material scientists, engineers, and biologists can facilitate a robust understanding of the complexities involved in optical thin films. Each discipline contributes unique perspectives that can enhance both theoretical and practical applications. For instance, while physicists examine light-matter interactions, engineers can focus on integration techniques for different devices.
Listening to diverse voices fosters a culture of innovation. A couple of examples include:
- Cross-Pollination of Ideas: When solar physicists collaborate with material scientists, they might develop new optical coatings that vastly improve solar panel efficiency, thus making renewable energy sources more accessible.
- Innovative Problem Solving: Combining knowledge from biophysics and materials science could lead to the creation of medical imaging systems that are less invasive and more effective.
Culmination
The exploration of optical thinness is not just a niche endeavor; it finds itself at the crossroads of various scientific and technological domains, shedding light on intricate mechanisms that govern our interaction with light and materials. In this article, we have ventured through the foundational principles, the diverse applications, and the mounting challenges that define this fascinating field.
Summary of Key Insights
Throughout our journey, several key insights have emerged regarding optical thinness:
- Defining Characteristics: We established that optical thinness is characterized by the interaction of light with materials that possess minimal thickness relative to the wavelength of light. This fundamental definition serves as the backbone for many applications and theories in the realm of optics.
- Interdisciplinary Significance: The implications of optical thin films extend beyond basic scientific understanding. Their roles in enhancing photonic devices, improving solar technologies, and influencing surface coatings are prime examples of their multifaceted nature.
- Measurement Techniques: Innovative measurement methods have advanced our understanding of optical thinness. Techniques have evolved from standard, established practices to more sophisticated approaches that account for environmental factors, thus improving accuracy and reliability.
- Future Research Directions: Identifying the limitations of current models paves the way for future research. As new technologies emerge, interdisciplinary approaches may provide fresh lenses through which optical thinness can be understood and utilized.
The insights garnered throughout this article highlight the necessity for ongoing exploration in the field of optical thinness. As new challenges arise, so too do potentials for innovation and discovery.
Implications for Future Research
Moving forward, the study of optical thinness holds several implications that can profoundly impact various fields:
- Technological Advancements: As technologies evolve, so will the needs and applications of optical thin films. Investing in research can lead to breakthroughs in areas like energy efficiency and data transmission.
- Enhanced Measurement Methodologies: There is a pressing need to develop even more refined techniques for the characterization of optical thinness under different environmental conditions. Improving measurement accuracy can drastically change how materials are utilized across industry sectors.
- Collaboration Across Disciplines: Interdisciplinary collaboration is essential. Scientists from physics, materials science, and engineering should work hand-in-hand, fostering a blend of insights and techniques that could unveil new functionalities of optical thin films.
A concerted focus on these areas will not only facilitate deeper understanding but will also foster advancements that hinge upon the principles of optical thinness. In summary, as the scientific community delves deeper into this subject, the potential applications are as limitless as the light that governs them.