Understanding UV-Vis Absorbance: Principles and Applications
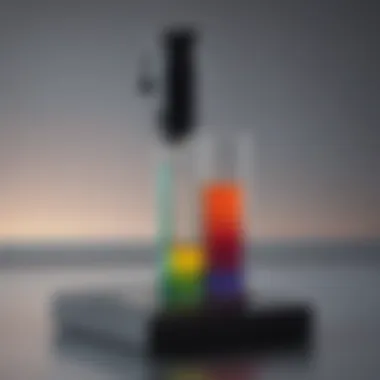
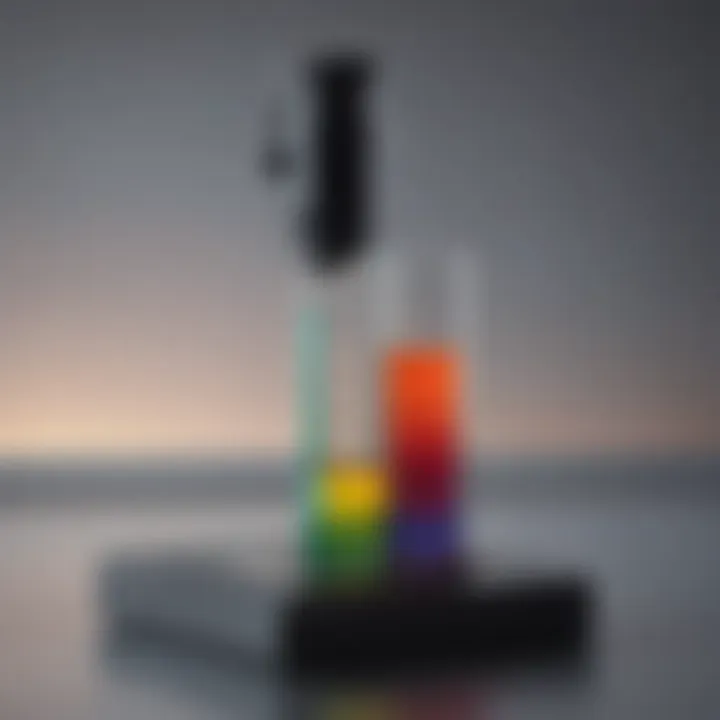
Intro
UV-Vis absorbance plays a crucial role in many scientific endeavors, ranging from chemical analyses to biological explorations. This technique assists researchers in determining the concentration of substances, identifying compounds, and understanding molecular structures. A basic grasp of the principles behind UV-Vis absorbance helps in leveraging its full potential across various applications.
Understanding how light interacts with matter is essential. When light passes through a sample, certain wavelengths are absorbed, and the intensity of the transmitted light decreases. This principle is fundamental in analytical chemistry, where it enables quantification of analytes.
Additionally, UV-Vis spectroscopy is not only limited to academic purposes; it extends its reach to industries, including pharmaceuticals and environmental monitoring. Researchers increasingly rely on it for efficiency and accuracy in their work.
In the following sections, we will explore key findings, significant connections, and practical applications of UV-Vis absorbance in detail. Each aspect will be examined thoroughly, providing a comprehensive understanding of this important scientific technique.
Prelims to UV-Vis Absorbance
UV-Vis absorbance is a critical analytical tool used across different scientific fields. Its capability to measure light absorption in the ultraviolet and visible spectrum makes it indispensable in studying chemical substances, biological processes, and material properties. Understanding UV-Vis absorbance is not merely about grasping its technicalities; it is about recognizing how it influences research outcomes and practical applications.
Definition of UV-Vis Absorbance
UV-Vis absorbance refers to the capacity of a substance to absorb light at specific wavelengths within the ultraviolet (200-400 nm) and visible (400-800 nm) range. When light passes through a sample, certain wavelengths are absorbed, leading to a measurable difference between the incident and transmitted light. This interaction is quantified using the absorbance formula, which lays the foundation for various scientific analyses.
The absorbance (A) can be defined mathematically as:
[ A = -\log_10(T) ]
Where ( T ) is the transmittance of the sample. This direct relationship enables researchers to determine the concentration of a sample and investigate its molecular characteristics effectively.
Importance in Various Scientific Disciplines
The significance of UV-Vis absorbance transcends individual scientific domains, integrating chemistry, biology, environmental science, and material science. Each discipline leverages this technique in unique ways:
- Analytical Chemistry: The ability to quantify concentrations precisely is vital in various chemical analyses.
- Biological Studies: UV-Vis is used in determining concentrations of biomolecules such as proteins and nucleic acids, aiding in molecular biology research.
- Environmental Monitoring: Assessment of pollutants in water and air is facilitated through UV-Vis techniques, identifying harmful substances that may not be visible to the naked eye.
- Material Science: The structural properties of new materials and compounds are analyzed, leading to advancements in technology and engineering fields.
Understanding these applications enriches one’s appreciation of UV-Vis absorbance. Its versatility is essential for advancing knowledge in different scientific areas, further emphasizing the need for careful analysis and interpretation within this framework.
"UV-Vis spectroscopy provides an essential gauge in a myriad of disciplines, showcasing its power and reliability in research and industry."
In summary, the introduction to UV-Vis absorbance lays the groundwork for appreciating the technique’s multifaceted roles in scientific exploration and innovation. Engaging with this material is crucial for students, researchers, and professionals aiming to leverage UV-Vis analysis in their respective fields.
Principles of UV-Vis Spectroscopy
Understanding the principles of UV-Vis spectroscopy provides a foundation for how this technique is applied in various scientific domains. The significance of this field lies in its ability to analyze chemical substances based on the interaction of light with matter. The UV and visible light regions of the electromagnetic spectrum are critical during absorbance measurements. This section emphasizes fundamental concepts that drive this technology, highlighting its importance in research and industry settings.
Basic Concepts of Light and Absorbance
Light, as a form of electromagnetic radiation, plays a vital role in UV-Vis spectroscopy. It consists of photons that possess energy proportional to their wavelength. Absorbance occurs when molecules in a sample absorb specific wavelengths of light, resulting in electronic transitions. These transitions can be between different energy states, mainly in the ultraviolet and visible ranges. The absorbed light corresponds to characteristic wavelengths, which can identify and quantify substances. Understanding these interactions is fundamental to effective spectroscopy practices.
Beer-Lambert Law and Its Applications
The Beer-Lambert Law is central to UV-Vis spectroscopy, establishing a quantifiable relationship between absorbance, concentration, and path length. The law states that absorbance (A) is equal to the product of molar absorptivity (ε), the concentration of the absorbing species (c), and the path length through which the light passes (l). Mathematically, this can be expressed as:
This principle underlies numerous applications, particularly in analytical chemistry, where it enables the determination of unknown concentrations in primary samples. Proper application of the Beer-Lambert Law requires adherence to certain limits, including linearity of response and appropriate sample preparation.
Types of UV-Vis Spectrophotometers
Different types of UV-Vis spectrophotometers are available, each with unique features and operational mechanisms. Understanding these types aids in selecting the right instrument for a specific application.
Single Beam Spectrophotometer
A single beam spectrophotometer utilizes one beam of light to pass through the sample and reference. This design simplifies the measurement process. Its primary characteristic is the lower cost compared to other types. It is user-friendly and suitable for many routine applications. However, a drawback is that this type may be less accurate because it does not continuously measure both the sample and a reference simultaneously, which can lead to deviations in absorbance readings.
Double Beam Spectrophotometer
The double beam spectrophotometer divides the light beam into two—one directed through the sample and the other through a reference. This dual approach allows for real-time comparison and improves accuracy. A notable characteristic is its ability to compensate for any fluctuations in light intensity. The increased precision makes it popular for laboratory studies requiring reliable data. Nevertheless, it is typically more expensive and complex than single beam models, which may not be ideal for all settings.
By grasping the principles behind these instruments, researchers can optimize their choice and application for specific scientific inquiries.
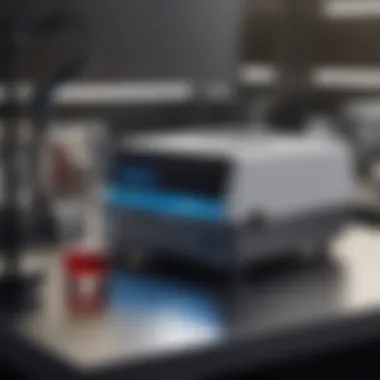
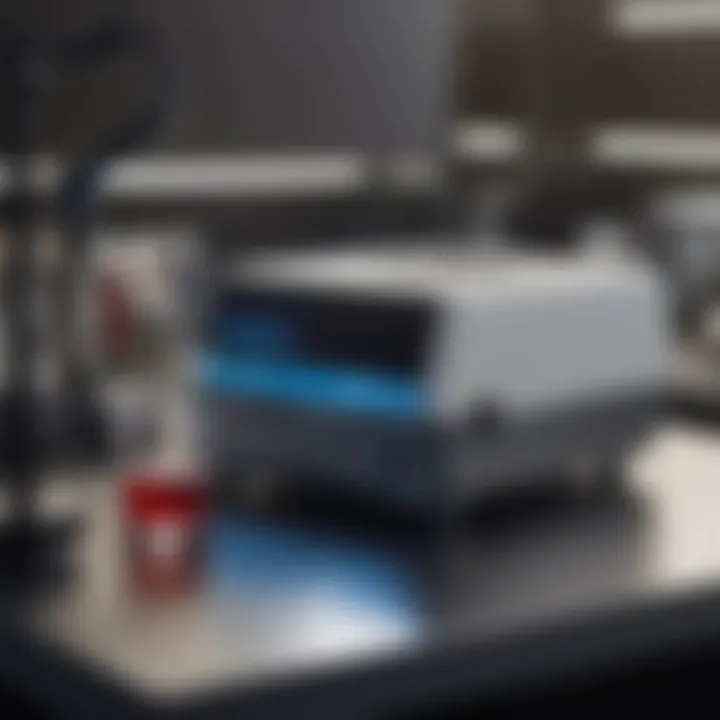
Operational Mechanics of UV-Vis Absorbance
The operational mechanics of UV-Vis absorbance are essential to understanding how this technique functions in practice. Knowledge of these elements is crucial for accurately conducting experiments and interpreting results. There are three main areas to explore: sample preparation, calibration of instruments, and data acquisition and analysis. Each of these components plays a significant role in the effectiveness and reliability of UV-Vis absorbance measurements.
Sample Preparation Techniques
Proper sample preparation is the foundation of successful UV-Vis analysis. The accuracy of absorbance measurements greatly depends on how well the sample is prepared. This involves several considerations:
- Purity of Samples: Samples must be free from contaminants. Impurities can absorb light and therefore distort the results.
- Concentration: Samples should be prepared at the correct concentration levels. This ensures that data collected falls within the linear range of the Beer-Lambert Law.
- Homogeneity: Samples should be uniformly mixed. Any particle settling or uneven distribution can lead to inconsistent results.
- Solvent Compatibility: The solvent used should not absorb strongly in the UV-Vis range of interest, preventing interference with the measurements.
In laboratory settings, using a spectroscopic cuvette made from materials like quartz or glass is important. These materials often do not absorb in the UV-Vis range, thus minimizing any baseline noise in the data.
Calibration of UV-Vis Instruments
Calibration of UV-Vis instruments is a critical step to ensure that the data obtained is accurate. When instruments are calibrated:
- Reference Standards: Known standards are used to create a calibration curve. This establishes the relationship between absorbance and concentration.
- Wavelength Calibration: The spectrophotometer must be calibrated for wavelength accuracy. This is often verified using a standard with a known absorbance peak.
- Regular Maintenance: Routine checks and maintenance are necessary to keep the instrument in good working condition. This involves cleaning optical components and ensuring alignment.
Miscalibration can lead to erroneous data, making it imperative to perform calibration before each set of experiments.
Data Acquisition and Analysis
Once samples are prepared and instruments calibrated, the focus shifts to data acquisition and analysis. This involves several steps:
- Collecting Data: The UV-Vis spectrophotometer scans the sample over a selected wavelength range. The software typically records absorbance at each wavelength automatically.
- Data Processing: Raw data often needs processing to correct for baseline noise or other artifacts.
- Interpreting Results: Absorbance data must be analyzed in the context of the established calibration curve to determine the concentration of unknown samples.
- Error Assessment: Understanding potential sources of error is important for data validation. Common errors may arise from instrumental drift or improper sample handling.
In summary, effective operational mechanics in UV-Vis absorbance encompass careful sample preparation, thorough calibration, and diligent data acquisition and analysis. Mastering these aspects significantly enhances the reliability of results.
Effective preparation and calibration are the keys to successful UV-Vis absorbance measurements.
Factors Affecting UV-Vis Absorbance Measurements
In UV-Vis spectroscopy, several factors significantly influence absorbance measurements. Understanding these factors is essential for accurate data interpretation and analysis. Incorrect assessments of absorbance can lead to erroneous conclusions. Therefore, a clear grasp of the variables that affect measurement results is crucial for chemists, biologists, and environmental scientists alike.
Solution Concentration and Path Length
The Beer-Lambert Law establishes a relationship between absorbance and concentration, which implies that absorbance increases linearly with higher concentrations of the absorbing species. The relationship is defined mathematically as:
[ A = \varepsilon c l ]
where A is the absorbance, (\varepsilon) is the molar absorptivity, c is the concentration of the solution, and l is the path length of the light through the sample.
- Concentration: Variations in the concentration of an analyte will alter absorbance. High concentrations can lead to saturation, causing a deviation from linearity.
- Path Length: The path length refers to the distance the light travels through the sample. Longer path lengths increase the likelihood of photon interactions with the absorbing species, enhancing absorbance.
Understanding how concentration and path length interact is critical for method optimization and calibration.
pH and Temperature Influence
pH and temperature are pivotal in UV-Vis spectroscopy. These factors can alter the chemical state of analytes, affecting their absorbance characteristics.
- pH: The ionization state and structure of certain compounds can change with pH, resulting in varied absorbance spectra. For example, phenols at different pH levels display distinct absorbance peaks, which can be used to study biological and chemical processes.
- Temperature: In general, an increase in temperature affects molecular motion, impacting the vibrations and rotations of bonds within a molecule. This can result in alterations in the absorbance spectra, leading to potential data variability.
Consistently monitoring and controlling these parameters enables accurate and reproducible results in UV-Vis measurements.
Solvent Types and Their Impact
The choice of solvent also plays a critical role in UV-Vis absorbance measurements. Different solvents interact variably with analytes, affecting their electronic transitions and hence their absorption characteristics.
- Polarity: Solvents with varying polarities can stabilize or destabilize molecular forms, altering their ability to absorb light. For instance, polar aprotic solvents often provide different spectra compared to nonpolar solvents.
- Viscosity: The viscosity of the solvent can impact the diffusion of molecules, influencing reaction rates and interactions during analysis, which could lead to differences in absorbance measurements.
- Solvent Absorbance: Solvents absorb light in certain UV-Vis regions. This can interfere with the analyte’s absorbance, especially in low-concentration samples. Understanding solvent characteristics ensures better data integrity and correct interpretation of results.
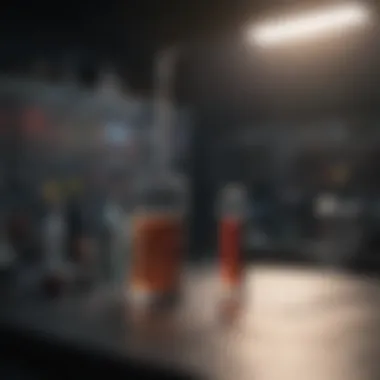
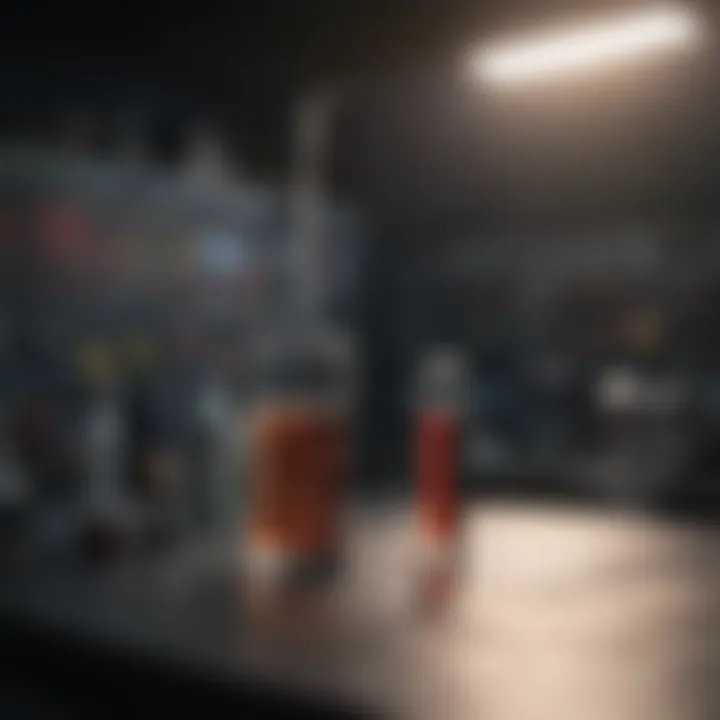
It is imperative to use appropriate controls when analyzing absorbance to account for matrix effects or interference from solvents.
Applications of UV-Vis Absorbance
The applications of UV-Vis absorbance are invaluable across diverse scientific fields. This technique serves as a cornerstone in various domains, encompassing analytical chemistry, biology, environmental science, and material science. Understanding its applications is essential for those pursuing research and practical work in these areas. Assessments and evaluations made through UV-Vis Absorbance offer significant insights, leading to advancements in both theory and practical applications.
Analytical Chemistry Applications
In the realm of analytical chemistry, UV-Vis absorbance plays a critical role. It is applied for quantifying the concentration of analytes in solutions, a task central to many chemical analyses. The method is preferred because of its simplicity, speed, and cost-effectiveness. Common applications include:
- Determination of Concentration: Using the Beer-Lambert Law, chemists can calculate the concentration of an unknown sample by comparing its absorbance to standard solutions.
- Quality Control: Industries utilize UV-Vis spectroscopy to ensure product consistency. It measures the purity of compounds, monitoring unwanted contaminants.
- Kinetics Studies: This technique allows for the observation of reaction rates by monitoring changes in absorbance over time.
Understanding these applications is essential for implementing successful analytical techniques in laboratories.
Biological and Medical Diagnostics
In biological and medical fields, UV-Vis absorbance functions as a pivotal diagnostic tool. It enables the detection and quantification of biomolecules such as proteins, nucleic acids, and metabolites. Key uses include:
- DNA and RNA Quantification: Absorbance at specific wavelengths allows for accurate assessment of nucleic acid concentrations, facilitating genetic research and diagnostics.
- Protein Analysis: UV-Vis spectroscopy helps in determining protein concentration, using absorbance at 280 nm as a standard.
- Clinical Diagnostics: UV-Vis is instrumental for various assays in clinical laboratories, enabling the detection of biomarkers in patient samples.
Through these applications, UV-Vis absorbance contributes significantly to understanding biological processes and disease mechanisms.
Environmental Monitoring
Environmental science also greatly benefits from UV-Vis absorbance techniques. Monitoring pollutants in air and water helps in assessing environmental health and compliance with regulations. Important applications include:
- Water Quality Testing: UV-Vis spectroscopy assesses water samples for contaminant levels, such as heavy metals and organic compounds.
- Air Quality Analysis: This method aids in detecting airborne pollutants by analyzing gas samples, crucial for environmental safety.
- Study of Lens and Watershed Health: Analyses of UV-Vis spectra from natural waters reveal information about the ecosystem, such as algal blooms and other indicators of ecosystem health.
Such monitoring opportunites are vital for ensuring public health and environmental sustainability.
Applications in Material Science
In material science, UV-Vis absorbance is applied to assess material properties and development. It is instrumental in processes related to nanomaterials, polymers, and coatings. Notable applications include:
- Characterization of Materials: Spectroscopic data helps characterize optical properties of new materials, which is essential in research and product development.
- Thin Film Analysis: This technique analyzes thin films in terms of thickness and uniformity, critical for electronics and coatings.
- Nanoparticle Studies: UV-Vis absorbance is employed to investigate the optical properties of nanomaterials, enabling advancements in photonics and nanotechnology.
These applications reflect the versatility and significance of UV-Vis spectroscopy in material innovation.
UV-Vis absorbance serves as a crucial tool across multiple fields, shaping advancements in analytical methods, diagnostics, environmental studies, and material development.
Interpretation of UV-Vis Spectra
Interpretation of UV-Vis spectra forms a crucial aspect of employing UV-Vis absorbance in scientific investigations. This process allows researchers to unveil valuable information about sample composition, concentration, and molecular structures. To extract maximum benefit from UV-Vis absorbance data, understanding the characteristics and implications of the spectra is essential.
Understanding Peaks and Absorbance Values
In UV-Vis spectroscopy, the absorbance spectrum reveals peaks that correspond to specific wavelengths of light absorbed by a sample. Each peak signifies the presence of a molecule absorbing light at a particular energy level, related to electronic transitions within the molecule. Typically, absorbance (A) is measured on the y-axis, while the wavelength (λ) is plotted on the x-axis.
Analyzing these peaks provides critical insights:
- Position of Peaks: The wavelengths at which peaks occur are indicative of the electronic structure of the absorbing species. Different molecular configurations yield distinct absorbance maxima, offering a fingerprint for identification.
- Height of Peaks: The height or intensity of the peaks reflects the concentration of the absorbing molecule. Using the Beer-Lambert law, it is possible to relate absorbance directly to concentration.
- Area under the Peaks: This integral can sometimes provide more useful information than a simple measurement of intensity, especially in complex mixtures where overlapping peaks might occur.
Careful examination of these elements allows for qualitative and quantitative analyses, helping to draw conclusions about materials or biological samples under study.
Common Errors in Data Interpretation
While interpreting UV-Vis spectra can yield profound insights, challenges often arise that can lead to misinterpretations. Being aware of common errors is crucial:
- Baseline Drift: Fluctuations in the baseline can obscure peaks and distort readings. Ensuring a stable reference before taking measurements is vital.
- Overlapping Peaks: Compounds that absorb at similar wavelengths can lead to overlapping peaks, complicating identification and quantification. This may require deconvolution techniques or the use of other analytical methods.
- Neglecting Instrument Calibration: Failure to regularly calibrate the spectrophotometer can introduce systematic errors in absorbance readings. Regular checks with standard solutions help maintain accuracy.
- Ignoring Environmental Factors: Factors such as temperature, pH, or solvent effects can influence absorbance measurements. Being mindful of these variables during the experiment is key to obtaining reliable results.
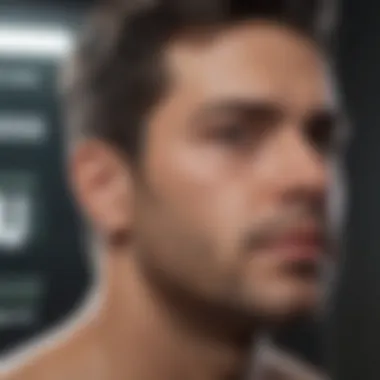
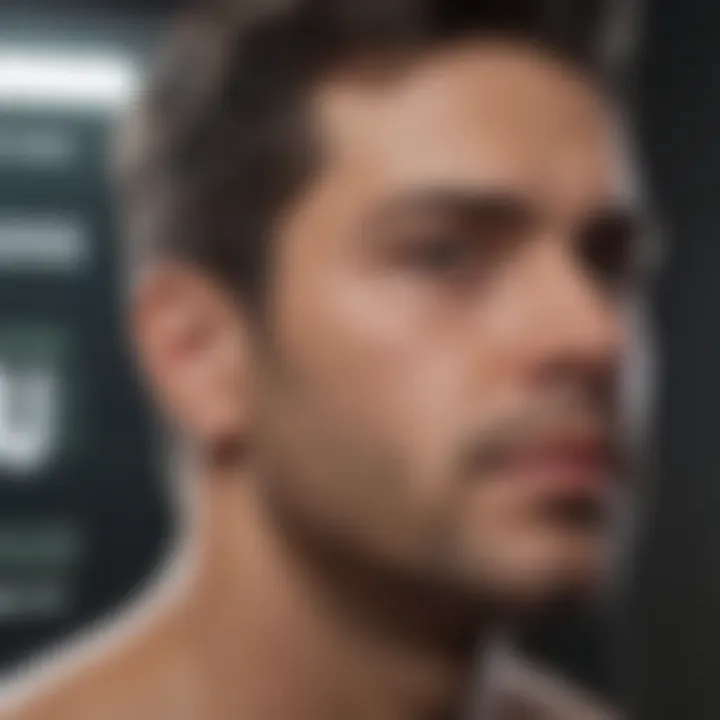
The interpretation of UV-Vis spectra is not just technical but requires a deep commitment to understanding underlying principles to avoid critical mistakes.
Recent Advances in UV-Vis Spectroscopy
Recent advancements in UV-Vis spectroscopy have expanded the capabilities and applications of this critical analytical technique. Researchers and industry professionals are continuously seeking to enhance the precision, sensitivity, and applicability of UV-Vis absorbance measurements. As technology evolves, new methodologies and integrations emerge, allowing for more comprehensive analyses in a range of fields.
Integration with Other Spectroscopic Techniques
The integration of UV-Vis spectroscopy with other spectroscopic techniques has led to significant enhancements in data interpretation and analysis. For example, combining UV-Vis with techniques such as infrared (IR) spectroscopy or nuclear magnetic resonance (NMR) spectroscopy can provide deeper insights into the molecular structure and behavior of substances. This synergy aids in the identification of molecular components, providing complementary information that single techniques may overlook.
Furthermore, methods like two-dimensional (2D) NMR extend the capacity of UV-Vis spectroscopy to elucidate complex mixtures. This integrated approach enables scientists to better analyze complex samples in varied contexts including pharmaceuticals, biochemical analysis, and materials science.
"The convergence of multiple spectroscopic techniques offers a powerful tool for analytical chemists aiming to obtain deeper insights into molecular dynamics."
Emerging Technologies in UV-Vis Analysis
Emerging technologies in UV-Vis analysis continue to push the boundaries of what is possible. Innovations such as miniaturized spectrometers allow for portability without sacrificing accuracy. These devices are suitable for fieldwork, enabling real-time monitoring and analysis in environmental studies and healthcare settings.
Another prominent advancement is the development of ultra-fast UV-Vis spectroscopy. This technique enables scientists to observe rapid processes in chemical reactions or biological systems. By capturing data on millisecond or even picosecond timescales, ultra-fast spectroscopy opens new frontiers in understanding dynamic systems.
Additionally, machine learning and artificial intelligence are being integrated into UV-Vis data analysis. These methods can process vast amounts of data more quickly and accurately than traditional techniques. Leveraging data from diverse samples facilitates predictive modeling and enhances the overall analytical capabilities of UV-Vis spectroscopy.
In summary, the advances in the integration of UV-Vis with other techniques and the emergence of new technologies highlight the ongoing evolution within this field. Understanding these trends is vital for researchers and professionals aiming to apply UV-Vis spectroscopy effectively in their work.
Challenges and Limitations
Understanding the challenges and limitations of UV-Vis absorbance is crucial for accurate scientific research. While this technique is powerful, it has inherent difficulties that can compromise results. Recognizing these challenges allows researchers to make informed decisions and ensures the integrity of data analysis. This section will explore specific elements related to matrix effects and the limitations of the Beer-Lambert Law, providing insights into their implications.
Matrix Effects on Results
Matrix effects refer to the influence of other substances present in a sample on the absorbance measurements. These substances can either enhance or suppress the absorbance of the target analyte. The complexity of real-world samples often leads to unexpected difficulties in interpretation. Therefore, proper consideration of the matrix is vital when designing experiments.
Common sources of matrix effects include solvents, additives, and other components in the sample mixture. For instance, a biological sample might contain proteins or salts that interfere with the absorbance spectrum. Understanding the nature of these matrix interactions is essential. Researchers can conduct tests to determine how various matrices affect the analyte response. This approach helps to mitigate the risk of erroneous conclusions based on altered absorbance values.
To ensure reliable results, it is advisable to use matrix-matched standards during calibration. This practice can significantly improve the accuracy of the measurements and aid in obtaining consistent results across varying sample conditions.
Limitations of Beer-Lambert Law
While the Beer-Lambert Law serves as the foundation for UV-Vis spectrophotometry, it has limitations that must be recognized. The law states that absorbance is directly proportional to concentration and path length. However, this linear relationship only holds true under certain conditions.
One major limitation arises when the absorbance values become very high. At high concentrations, deviations from linearity can occur due to factors such as light scattering or molecular interactions. This results in non-linear absorbance behaviors that the Beer-Lambert Law cannot accurately predict.
Another limitation pertains to the assumption of homogeneous samples. The Beer-Lambert Law presumes that the sample is uniformly mixed, which is not always the case. Inhomogeneous samples can lead to variations in local concentration and, subsequently, in absorbance readings.
To address these limitations, researchers often employ dilution strategies to bring samples within the linear range of the Beer-Lambert Law. Additionally, alternative analytical methods such as chemometrics can be used to analyze complex data more effectively. By being aware of these limitations, scientists can better design their experiments and interpret their results with a critical eye.
"Understanding the limitations of UV-Vis methods enhances the robustness of analytical results and counters potential errors during experiments."
Future Directions in UV-Vis Research
The advance of UV-Vis spectroscopy is crucial in understanding the material and biological world. Researchers continue to uncover new ways to utilize this technique, broadening its scope and impact across various fields. Future directions encompass potential new applications and advancements in instrumentation that promise to enhance both its capability and accuracy.
Potential New Applications
The potential for new applications within UV-Vis spectroscopy is vast. The integration of this technique into areas such as food quality assurance is emerging. It can analyze the presence of contaminants or assess nutritional components, ensuring safety and quality for consumers.
Moreover, using UV-Vis absorbance can be beneficial in pharmaceutical research. The technique allows real-time monitoring of drug formulations, vital for quality control and consistency in production. Another exciting application lies within eco-friendly practices, where UV-Vis spectroscopy can assess water quality. It enables the detection of pollutants quickly and efficiently, supporting environmental conservation efforts.
Recent studies have also suggested that integrating UV-Vis spectroscopy with artificial intelligence could enhance data interpretation. AI can analyze complex data sets and identify patterns that may not be evident to the naked eye. This opens the door for applications in personalized medicine or for tailoring treatment plans based on a patient's specific biological profile.
Advancements in Instrumentation
Technological advancement brings improvements in UV-Vis instrumentation. Miniaturized and portable spectrophotometers are being developed, allowing for field-based analysis in real-time. This can significantly benefit industries such as agricultural monitoring or on-site environmental research, where immediate results drive critical decision-making processes.
High-throughput systems that can measure multiple samples quickly also gain popularity. These systems can optimize workflows in clinical laboratories, accelerating drug testing or toxicology studies. Furthermore, the development of multivariate analysis in conjunction with UV-Vis data is a promising area. This analytical approach allows researchers to consider many variables at once, enhancing the depth of analysis.
In summary, the future of UV-Vis research looks promising. Through potential new applications and advances in instrumentation, this technique will likely see an expansion in its role across various scientific and industrial domains. The ongoing exploration of its capabilities is fundamental for future scientific endeavors, potentially leading to groundbreaking discoveries.