Unit of Surface Energy: Key Insights for Researchers
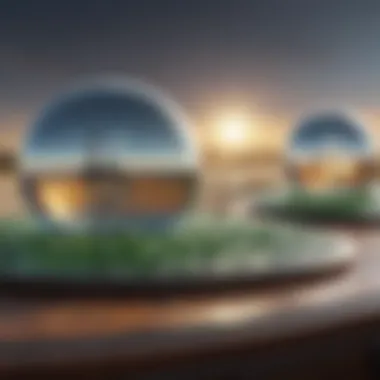
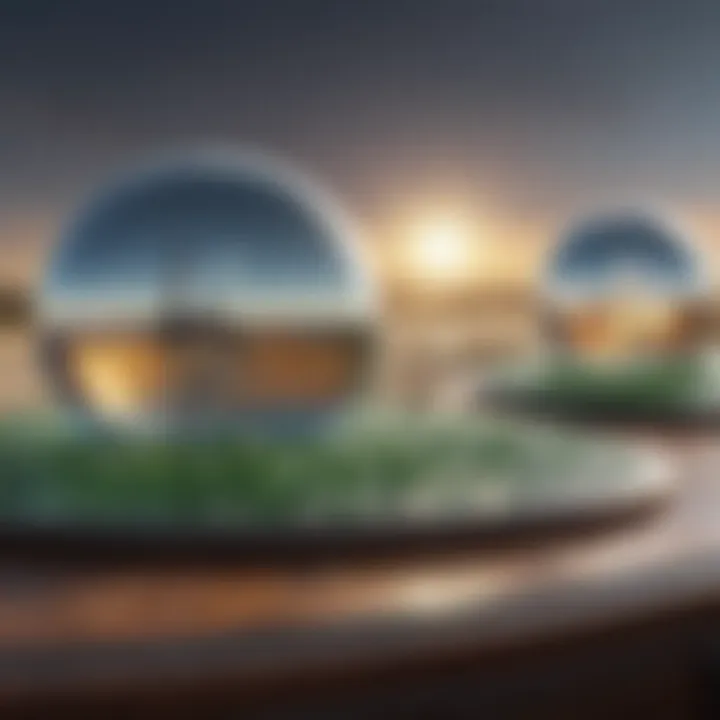
Intro
Surface energy plays a pivotal role in the realms of material science, physics, biology, and chemistry. It represents the excess energy at the surface of a material compared to its bulk, influencing numerous physical processes. Understanding this concept is crucial, especially when studying phenomena such as wetting, adhesion, and surface modifications.
This article intends to dissect the unit of surface energy, which is often measured in joules per square meter (J/m²). This measure not only allows scientists to quantify surface energy but also aids in comparing the energy characteristics of different materials. The intricate connections between surface energy and material properties are significant for various applications, making this topic essential for students and professionals in related fields.
Prelims to Surface Energy
Surface energy is a vital concept that plays a key role across diverse scientific disciplines. It underpins various phenomena, ranging from material interactions to biological functions and chemical reactions. Understanding surface energy leads to insights into how materials behave at their interfaces, which is often where many critical interactions occur. In this section, we will explore the foundational aspects of surface energy, its definition, and its relevance in scientific inquiry.
Definition of Surface Energy
Surface energy can be defined as the excess energy at the surface of a material compared to its bulk. This energy arises from the disruption of intermolecular bonds that occurs when a surface is created. For instance, when a solid is cleaved or a liquid droplet forms, the molecules at the surface experience different forces than those in the bulk. As a result, the surface energy can be quantitatively measured and expressed in various units, such as joules per square meter or ergs per square centimeter.
Surface energy is significant because it determines how materials interact with each other and their environment. In general, higher surface energy materials tend to be more reactive and universally less stable. Therefore, studying surface energy gives researchers a deeper game about a material's stability, reactivity, and affinity for other substances.
Importance in Scientific Research
The significance of surface energy extends into numerous scientific fields, including materials science, biology, and chemistry. In materials science, surface energy influences processes like adhesion, wetting, and coating applications. Understanding these principles facilitates the development of new materials with tailored properties, allowing advancements in technology and engineering.
In biology, surface energy affects cellular phenomena such as membrane stability and interactions with pathogens. Understanding these principles can aid in the development of medical treatments or strategies to inhibit microbial growth.
In chemistry, surface energy plays a pivotal role in catalysis. Catalysts with high surface energy can provide more active sites for chemical reactions, thus increasing reaction rates and efficiency.
In summary, surface energy is not just a theoretical concept; it has broad applications and implications that extend into practical realms. It is essential for understanding a material's characteristics and interactions, which can lead to breakthroughs in various scientific endeavors.
The study of surface energy is foundational for advancements in technology, health sciences, and environmental applications.
Physical Principles of Surface Energy
Understanding the physical principles of surface energy is essential for grasping its implications across various scientific domains. Surface energy impacts several phenomena, including adhesion, wetting, and capillarity. More significantly, it influences material properties, which are critical in fields such as material science, biology, and chemistry.
The foundation of surface energy lies in the interactions between molecules at the boundaries of different phases, be it solid, liquid, or gas. These interactions dictate how substances interact at their interfaces, which has profound consequences on applications ranging from coatings to biological systems. Moreover, the thermodynamic equilibrium at surfaces plays a key role in determining stability and reactivity. Therefore, a thorough understanding of these physical principles can help in predicting and controlling surface behavior in both theoretical and practical contexts.
Molecular Interactions at Surfaces
Molecular interactions at surfaces are primarily governed by forces such as van der Waals forces, hydrogen bonding, and electrostatic forces. These forces vary with the nature of the materials involved, and they are crucial in determining the overall surface energy of a system. Specifically, the arrangement and density of molecules at the surface can lead to different energy states.
Surfaces with higher molecular densities typically exhibit higher energy due to stronger intermolecular interactions. Conversely, materials with lower molecular density at their surfaces tend to be less stable. This is particularly relevant in applications requiring specific adhesive or wetting properties. For instance, low-energy surfaces may repel liquids, leading to poor adhesion, whereas high-energy surfaces can promote better interaction with coatings or biological materials.
Understanding these interactions can assist scientists in designing materials with desired characteristics. Applications include optimizing paints that require adherence to various substrates and creating biocompatible surfaces for medical devices.
Thermodynamics of Surface Phenomena
Thermodynamic principles underpin the behavior of surfaces and how energy is absorbed or released. The fundamental concept involves the balance of energy associated with surface formation and molecular behavior. When a surface is created, energy is required to break the intermolecular bonds in the bulk material. This energy becomes significant in driving reactions or physical changes at surfaces.
The concept of surface tension is closely linked to these thermodynamic principles. Surface tension represents the energy required to increase the surface area of a liquid, which is a result of molecular interactions at the interface. Increasing surface area reduces the stability of a liquid, making it more prone to changes such as evaporation or instability under mechanical stress.
Moreover, understanding the thermodynamics of surface phenomena has practical implications. It allows researchers and engineers to predict how materials will behave under different conditions, impacting designs in systems ranging from microfluidics to industrial coatings.
Understanding the underlying molecular interactions and thermodynamic principles of surface energy is key to unlocking advanced applications across diverse scientific fields.
Units of Surface Energy
Understanding units of surface energy is crucial for its application across various scientific fields. The measurement of surface energy quantifies the excess energy at a surface compared to the bulk of a material. This concept impacts diverse areas such as material science, biological systems, and chemical reactions. Different scientific disciplines utilize various units, which can lead to better experiments, results, and applications. Here, we discuss the prominent units used to express surface energy, each offering unique insights and practical uses.
Joules per Square Meter
The Joule per square meter (J/m²) is the SI unit for surface energy. It describes the energy required to create a new surface area of one square meter. This unit is widely accepted in scientific literature and commonly used in engineering and materials research. For example, in studying polymers or coatings, understanding surface energy in J/m² provides essential insight into how materials interact with each other and their environment.
Calculating surface energy in Joules per square meter is relatively straightforward. It involves determining the work necessary to create a surface. A high surface energy indicates that a material has a strong affinity for interaction with its surroundings, often resulting in good adhesion properties. Conversely, low surface energy materials may show resistance to wetting and adhesion.
Ergs per Square Centimeter
Another useful unit for measuring surface energy is the Erg per square centimeter (erg/cm²). Though less common than Joules per square meter, it is still widely used in some branches of physics and chemistry, particularly in the context of older literature. One Joule is equivalent to 10^7 ergs. Thus, both units effectively convey the same information but target different audiences.
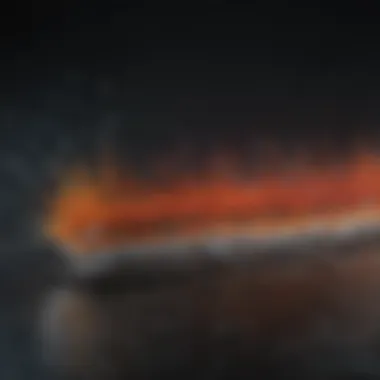
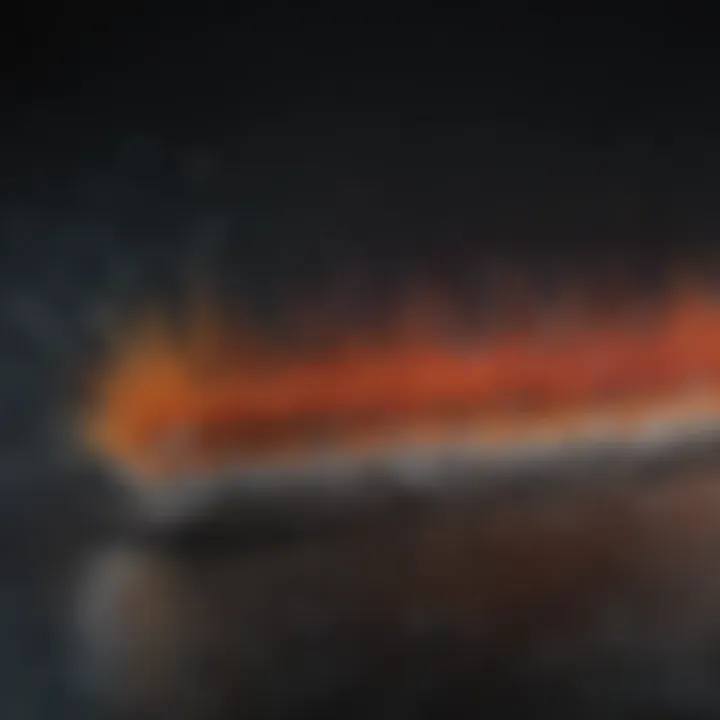
Using ergs per square centimeter can provide a more granulated perspective in some discussions, especially where traditional methods remain in use. In cases where experimental data is reported in ergs, understanding this unit enables researchers to translate and interpret findings accurately.
Laplace Pressure and Surface Tension
Surface energy is intimately related to both Laplace pressure and surface tension. Laplace's law describes the pressure difference across the interface of a curved liquid surface. This law helps explain how surface energy plays a role in phenomena like droplet formation and stability. The concept of surface tension, closely linked to surface energy, represents the energy required to increase the surface area of a liquid. Higher surface tension indicates higher surface energy.
Laplace pressure can be critical in applications involving bubbles or droplets. By understanding the relationship between pressure, surface tension, and surface energy, scientists can predict and manipulate behaviors for practical applications, such as in materials design and biological processes.
"Surface energy influences numerous physical phenomena and can redefine our understanding of interactions at the molecular level."
In summary, mastering the different units of surface energy is essential. Whether using Joules per square meter or ergs per square centimeter, recognizing how these measurements relate to physical principles aids researchers and practitioners in various fields. Understanding the influence of Laplace pressure and surface tension further highlights the interconnected nature of these concepts in real-world applications.
Measurement Techniques of Surface Energy
Understanding surface energy is crucial for a range of applications in scientific research and material science. The measurement techniques of surface energy provide insights into how materials interact with liquids and gases. By defining the surface energy accurately, researchers can predict and manipulate behaviors such as adhesion, wetting, and spreading. The reliability of these measurements significantly impacts both theoretical studies and practical applications.
Contact Angle Measurements
The contact angle is a fundamental parameter in assessing surface energy. It is determined by the angle formed between a liquid droplet and the solid surface it rests upon. The importance of contact angle measurements lies in their ability to reflect the wettability of a surface. A lower contact angle indicates better wettability and thus, higher surface energy.
- Procedure: To perform this measurement, a small droplet of liquid is placed on a solid surface. Using a goniometer, the angle where the liquid meets the solid is measured.
- Analysis: The data collected can be used to calculate surface energy using various models like the Young's equation.
- Implications: This technique is widely used across industries, such as coatings and ink adhesion, helping to inform product formulations and surface treatments.
Wilhelmy Plate Method
The Wilhelmy plate method offers another effective approach to measure surface energy. This technique involves immersing a thin plate made of specific materials into a liquid and recording the force exerted on the plate by the liquid surface tension. Here’s how it works:
- Setup: The plate is vertically positioned to touch the liquid surface.
- Force Measurement: As the plate goes deeper into the liquid, capillary action and surface tension create forces that can be measured with a balance.
- Calculating Surface Energy: By applying the relationship between the force and surface tension, one can derive the surface energy.
- Advantages: This method is praised for its precision and simplicity, often used in laboratory conditions to obtain consistent results.
Pendant Drop Method
The pendant drop method is another prominent technique for assessing surface energy. This technique utilizes a drop of liquid suspended from the bottom of a syringe to evaluate the surface properties.
- Drop Creation: A droplet is formed on the end of a needle while the shape of the droplet is observed.
- Analysis: The droplet shape is meticulously analyzed using advanced imaging techniques to derive the surface tension.
- Calculations: Mathematical equations, such as the Young-Laplace equation, allow for determining the interfacial tension based on the droplet curvature.
- Benefits: The pendant drop method is particularly useful for determining values at varying conditions like temperature and pressure, making it flexible for various research fields.
These measurement techniques are integral to advancing our understanding of surface energy and its applications.
Surface Energy in Material Science
Surface energy plays a pivotal role in material science, influencing the behavior and properties of materials at the molecular level. Understanding surface energy is essential for predicting how materials will interact with their environment, which impacts a broad range of applications from electronics to biomaterials.
One key aspect of surface energy is its influence on the mechanical properties of materials. Materials with high surface energy often exhibit better adhesion and wettability, which is crucial for applications such as coatings and composites. For instance, polymers with appropriate surface energy can bond well with paints and adhesives, enhancing durability and performance.
Influence on Material Properties
The relationship between surface energy and material properties is significant. High surface energy materials tend to have greater reactivity, influencing how they chemically interact with other substances. This can affect the material’s corrosion resistance, thermal stability, and even its electrical properties. Additionally, materials with lower surface energy may repel liquids, which can be advantageous or disadvantageous depending on the application.
- Wettability: High surface energy generally leads to better wettability, essential in industries where coating uniformity is critical.
- Adhesive Bonding: Surface energy affects how well adhesives bond to substrates, especially for varied surfaces like metals and plastics.
Applications in Coating Technologies
In coating technologies, understanding surface energy becomes crucial for designing effective coatings. Surface energy dictates how coatings spread, adhere, and perform over time. In this context, an appropriate balance of surface energy can determine the long-term effectiveness of protective coatings against environmental stresses.
For example, hydrophobic coatings designed to repel water rely on low surface energy materials, while hydrophilic coatings require a different approach, often utilizing high surface energy substances to promote adhesion and spreading.
Key Considerations in Coating Development:
- Material Compatibility: The surfaces to be coated must align in terms of surface energy to ensure proper adhesion.
- Application Methods: Spray, dip, or roll application methods can be influenced by surface energy; the right technique can enhance the quality of the coating.
- Durability and Resistance: High surface energy coatings may provide better resistance against degradation, while low energy coatings can offer self-cleaning properties.
Achieving the desired surface energy is a critical step in coating technology. It helps in maximizing the performance of coatings in various environments.
Role of Surface Energy in Biology
Surface energy plays a critical role in numerous biological processes. This relevance can be seen clearly through two primary aspects: cell membrane dynamics and microbial adhesion mechanisms. Understanding these areas not only enhances our grasp of fundamental biology but also aids in fields like biomedical engineering and materials science.
Cell Membrane Dynamics
The cell membrane is essential for maintaining cellular integrity and regulating interactions with the environment. Surface energy influences the fluidity and flexibility of cellular membranes. Several factors determine these properties, including lipid composition and the presence of proteins.
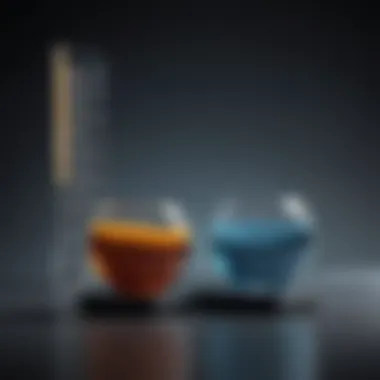
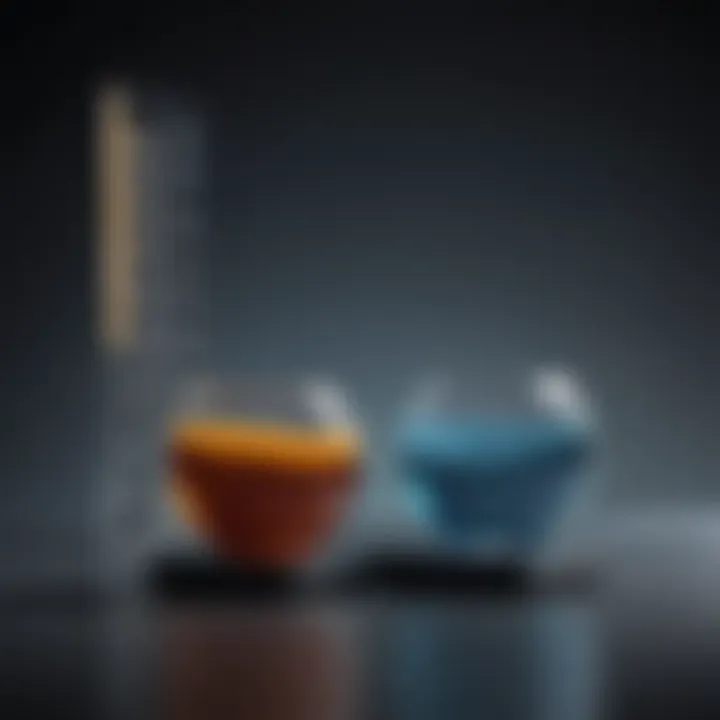
The alignment of lipid molecules in the bilayer affects surface energy directly. A higher surface energy can lead to increased tension, which may disrupt the membrane structure. On the other hand, lower surface energy can facilitate membrane fluidity, promoting various cellular functions such as signaling, transport, and communication.
Key Considerations of Cell Membrane Dynamics:
- Lipid Composition: The types of lipids affect both surface energy and membrane behavior.
- Temperature: Temperature variations influence fluidity and stability of the membrane.
- Protein Functionality: Proteins embedded within the membrane contribute to surface interactions and functionality.
"Understanding the biophysics of cell membranes provides insights into many cellular processes."
Microbial Adhesion Mechanisms
Microbial adhesion is fundamental to processes such as colonization, biofilm formation, and pathogenesis. Surface energy plays a vital role in how microorganisms interact with surfaces, affecting their attachment abilities. Microbes tend to adhere more strongly to surfaces with high surface energy.
The factors that influence microbial adhesion include surface roughness, hydrophobicity, and surface functional groups. For instance, bacteria typically prefer to attach to hydrophobic surfaces, as these facilitate stronger van der Waals forces. Thus, surface energy can be a deciding factor in the efficacy of antimicrobial treatments and material design in medical devices.
Key Elements Influencing Microbial Adhesion:
- Surface Roughness: Affects the degree of adhesion and biofilm development.
- Hydrophobicity: Typically enhances microbial retention on surfaces.
- Chemical Composition: Surface chemistry impacts initial attachment and biofilm stability.
Addressing microbial adhesion through surface energy manipulation offers promising avenues in infection control and material development. This interplay between surface energy and biological processes illustrates the extensive impact of surface energy within biological systems.
Applications of Surface Energy in Chemistry
Surface energy plays a significant role in various chemical applications. Its importance is highlighted in areas such as catalysis and nanotechnology. Understanding how surface energy affects chemical reactions enables researchers to optimize processes and materials. Here, we explore how these applications manifest in practical scenarios.
Catalysis and Reaction Rates
In catalysis, surface energy is crucial. Catalysts often operate at interfaces where chemical reactions occur. The rate of a reaction can depend on the surface energy of the catalyst material. Higher surface energy typically leads to a larger number of active sites available for reactions.
- Active Sites: The number of active sites on a catalyst directly influences its efficiency. Increased surface energy can increase the availability of these sites, enhancing the overall reaction rate.
- Adsorption: Surface energy also governs how reactants adsorb onto a catalyst's surface. A suitable surface energy allows molecules to adhere better, fostering interaction and promoting the reaction.
- Selectivity: Different materials can provide different surface energies, influencing selectivity during reactions. Researchers can tailor surface properties to favor specific reaction pathways, thus improving product yields.
In summary, understanding surfac energy is essential for optimizing catalytic processes, enhancing reaction rates, and achieving better selectivity in chemical transformations.
Nanotechnology and Surface Functionalization
In the field of nanotechnology, surface energy dictates how materials behave at the nanoscale. Engineers and scientists manipulate surface energy to functionalize surfaces, which leads to numerous applications in various domains.
- Surface Modification: By altering surface energy, scientists can enhance or diminish wettability. This is crucial in applications like coatings, where properties such as durability and corrosion resistance are developed.
- Nanoparticle Stabilization: Surface energy plays a pivotal role in forming stable nanoparticle dispersions. High surface energy can lead to agglomeration, while lower surface energy can prevent this, ensuring nanoparticles remain uniformly dispersed in a medium.
- Drug Delivery Systems: In pharmaceutical applications, effective drug delivery often relies on functionalized surfaces, which can improve interactions with biological systems. Adjusting surface energy impacts drug adsorption and release rates, hence optimizing therapeutic effectiveness.
By focusing on surface energy, significant advancements can be achieved in nanotechnology. This allows for better design of materials tailored to specific functions, furthering innovation in the chemical industry and beyond.
Calculating Surface Energy
Calculating surface energy is pivotal for understanding how materials interact at interfaces. These calculations inform us about the physical and chemical properties of materials. This can significantly impact fields such as material science, nanotechnology, and coatings. Accurate measurements of surface energy help predict behaviors such as wetting, adhesion, and the overall stability of materials in various environments.
Several methods are utilized to calculate surface energy, with each method offering unique insights into different aspects of material interactions. Among these methods, the Owens-Wendt method and the Fowkes method stand out for their efficiency and reliability in providing surface energy values. Let us explore these calculation methods in detail to uncover their principles and applications.
The Owens-Wendt Method
The Owens-Wendt method is a widely accepted approach for calculating surface energy based on contact angle measurements. It combines the concepts of dispersive and polar components. Dispersive forces arise from van der Waals interactions, while polar forces relate to dipole interactions.
In practical terms, the method requires the measurement of contact angles of various test liquids on a solid surface. The surface energy is obtained by analyzing the relationship between these contact angles and the known properties of the test liquids.
Key points of the Owens-Wendt method include:
- Two-component model: The total surface energy is divided into dispersive and polar parts.
- Contact angles: Measuring angles from multiple liquids allows better estimation of the surface energy components.
- Equation utilization: The method uses specific equations linking contact angles to surface energy contributions, facilitating accurate calculations.
This method is particularly useful for materials that may have complex interaction behaviors, such as polymers and coatings. Also, being a relatively straightforward approach means that it can be applied in many laboratory settings.
The Fowkes Method
The Fowkes method offers another approach to calculating surface energy, focusing on the concept of work of adhesion, specifically using a similar contact angle methodology.
This method builds on the idea that the interfacial tensions between two different phases can be related to their respective surface energies. It emphasizes the calculation of the total surface energy as a summation of the individual contributions from dispersive and polar components.
Important aspects of the Fowkes method are:
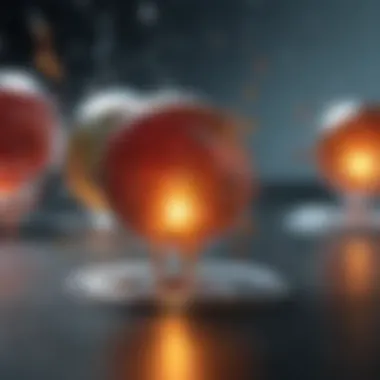
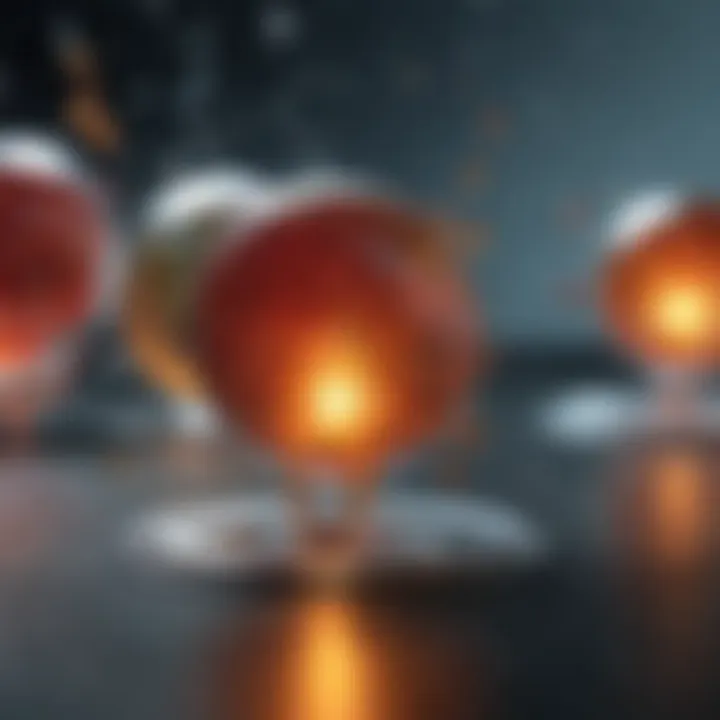
- Single component approach: It simplifies the calculations by deriving relationships for individual components rather than compound interactions.
- Consistency and compatibility: It provides results consistent with Owens-Wendt, thus offering a cross-verification of surface energy values.
- General applicability: Useful for a broad range of materials, making it favorable in both academic and industrial settings.
Impact of Surface Energy on Contact Phenomena
The examination of surface energy is crucial when analyzing contact phenomena. This area explores how different substances interact at their interfaces and how these interactions influence various processes. The implications of surface energy on contact phenomena extend to fields like material science, biology, and chemical engineering, making it vital to understand this topic clearly. Analyzing how surface energy affects adhesion, spreading, and stability can lead to better designs in devices and materials.
Wetting and Spreading Behavior
Wetting is the ability of a liquid to maintain contact with a solid surface. The degree of wetting is primarily determined by the surface energies of both the liquid and the solid involved. When a droplet is placed on a surface, the liquid will spread depending on its surface tension relative to the surface energy of the solid. If the surface energy of the solid is high compared to the liquid's surface tension, the liquid will spread more, resulting in better wetting. Conversely, a typical example is when water droplets bead up on a waxy surface due to lower energy interactions.
Factors influencing wetting behavior include:
- Surface Cleanliness: Contaminants alter surface energy, impacting wetting.
- Surface Roughness: Increased roughness enhances the wettability in many cases, potentially creating superhydrophobic or omniphobic surfaces when designed correctly.
- Temperature: It can affect the viscosity of liquids and the mobility of the molecules at the solid-liquid interface, influencing wetting behavior.
Understanding these nuances is essential for applications such as paint formulation, ink jet printing, and developing waterproof materials. Accurate knowledge of surface energy helps in optimizing these processes.
Bubble Formation and Stability
Bubble dynamics exemplify the role of surface energy in contact phenomena. The formation of bubbles is a classic demonstration of surface tension, which is a manifestation of surface energy. High surface energy surfaces tend to create stronger bubbles due to increased resistance to deformation. The balance between gravitational and surface forces dictates bubble stability.
Stability in bubbles can be affected by factors like:
- Liquid Properties: Viscosity and surface tension directly influence how long a bubble can maintain its shape.
- Interface Interactions: Surfactants can modify surface energy, affecting bubble stability significantly. Surfactants lower surface tension, encouraging bubble formation but can also lead to quicker collapse if not balanced correctly.
- Environmental Conditions: Surrounding pressure, temperature, and concentration of other substances in the solution greatly affect bubble behavior.
An understanding of bubble behavior is vital in various industries, including pharmaceuticals, food & beverages, and petrochemicals. Improved manipulation of surface energy can lead to enhanced product quality and manufacturing efficiency.
"Surface energy plays a decisive role in how materials interact and function in both natural and engineered systems."
Recognizing the impact of surface energy on contact phenomena can lead to innovative solutions across multiple scientific disciplines, refining our approaches to research and development.
Future Research Directions in Surface Energy
The exploration of surface energy remains a vital area of scientific investigation, with numerous implications across various fields. As we advance, the focus on future research directions can illuminate new applications and enhance existing technologies. This section highlights two significant aspects of upcoming research: emerging technologies and interdisciplinary approaches.
Emerging Technologies
Emerging technologies hold great promise for enhancing our understanding of surface energy. These innovations could revolutionize how we measure, manipulate and apply surface energy in practical contexts. For instance, advancements in nanotechnology and material fabrication techniques allow for precise control at the molecular level. Increased understanding of surface properties can lead to the development of more efficient catalysts, improved sensors, and novel materials with tailored characteristics.
Key emerging technologies include:
- Advanced Imaging Techniques: Innovations in microscopy, such as atomic force microscopy (AFM) and scanning electron microscopy (SEM), provide detailed views of surface structures. These tools are essential for understanding how surface energy influences material behavior.
- Smart Coatings: New materials are being designed to change properties in response to environmental triggers. These coatings can offer self-cleaning capabilities, anti-fogging features, and enhanced durability, all of which are influenced significantly by surface energy.
- 3D Printing: This technology is evolving to incorporate surface energy considerations, enabling the fabrication of complex geometries that achieve desired interactions and performance in applications ranging from medicine to electronics.
These advancements not only illustrate the potential of technology in studying surface energy but also suggest practical applications that can impact industries ranging from healthcare to aerospace.
Interdisciplinary Approaches
Interdisciplinary collaboration is critical for fostering profound insights into surface energy. By integrating knowledge from various scientific domains, researchers can enhance their understanding of surface phenomena and their broader implications.
Some key interdisciplinary areas to consider are:
- Material Science and Biology: Studying how surface energy interacts with biological systems can unveil insights into cell adhesion, drug delivery mechanisms, and tissue engineering. This can lead to biomedical innovations based on surface interactions.
- Chemistry and Physics: These fields can merge to explore how molecular dynamics or thermal properties relate to surface energy. Such studies can deepen our understanding of catalytic processes and energy efficiencies.
- Environmental Science: Investigating surface energy's effects on phenomena like wetting and erosion can inform ecological responses to environmental changes. This knowledge is crucial for sustainable materials and technologies.
Culmination
The conclusion section emphasizes the significance of understanding surface energy across various scientific fields. Surface energy plays a vital role in phenomena such as wetting, adhesion, and stability of bubbles. These factors are crucial in applications ranging from material science to biological systems and chemical reactions.
Summary of Key Points
In this article, several fundamental concepts regarding surface energy have been explored:
- Definition and Importance: Surface energy is a significant property impacting physical and chemical interactions at interfaces.
- Units of Measurement: Common units include Joules per square meter and ergs per square centimeter, crucial for quantifying surface interactions.
- Measurement Techniques: Methods like contact angle measurements and the Wilhelmy plate approach provide insights into surface energy values.
- Applications Across Disciplines: From influencing material properties in engineering to its role in cell membrane dynamics in biology, surface energy is integral to advancements in multiple fields.
Focusing on these key areas, we affirm that a thorough understanding of surface energy leads to enhancements in technology and scientific knowledge.
The Importance of Surface Energy in Science
Surface energy is not merely a theoretical concept; its practical implications are far-reaching. In material science, understanding surface energy influences how materials interact with each other and their environment. This is vital for the development of coatings and composites that are durable and efficient.
In biological research, surface energy is fundamental in understanding cell interactions and microbial behavior. This knowledge can lead to breakthroughs in medical treatments and bioengineering.
Moreover, in chemistry, surface energy is crucial for catalytic processes. It affects reaction rates and the efficiency of catalysts.
Overall, surface energy is an essential parameter that intersects various scientific disciplines, influencing research, innovation, and practical applications.