3D Printing Cells: Innovations in Biotechnology
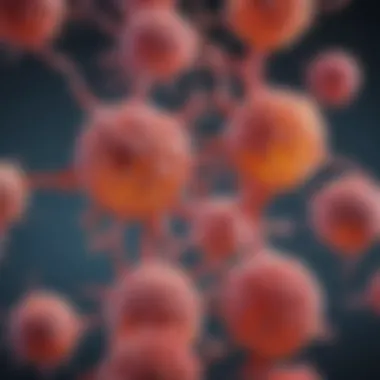
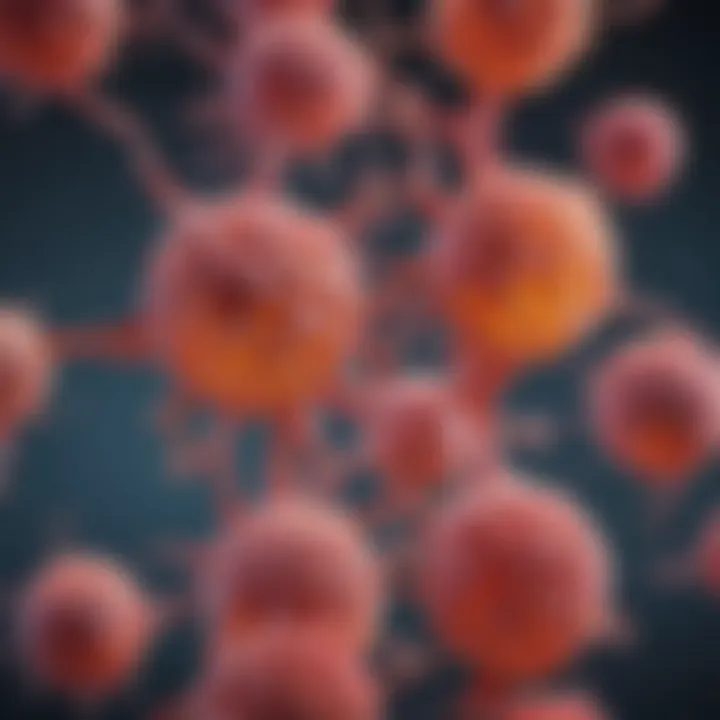
Intro
In recent years, the intersection of engineering and life sciences has witnessed significant evolutionary strides, with 3D printing of cells pushing the boundaries of what’s possible. This technology, once the realm of sci-fi, is now a vibrant area of research that holds the promise to revolutionize how we approach healthcare and biotechnology. The convergence of these fields not only enables the crafting of living tissues but also opens up new avenues in regenerative medicine and tissue engineering.
The intricate process of 3D bioprinting involves layering living cells with biomaterials, creating structures that can mimic the complexities of natural tissues. As researchers are diving deeper into this technology, they are beginning to uncover not just the immense potential it holds, but also the numerous challenges that accompany it.
Key aspects we will explore include:
- Recent innovations in cellular fabrication.
- Applications in regenerative medicine and tissue engineering.
- The multifaceted challenges that demand our attention.
- Ethical considerations surrounding the use of this technology.
As we unpack these topics, the pertinence of 3D printing in the realm of biotechnology will be made clear. By illuminating the advancements, we can gain a better grasp of how these methodologies are tailored to address the pressing needs of modern healthcare and society as a whole.
Prolusion to 3D Cell Printing
The concept of 3D cell printing marks a significant turning point in biotechnology, catalyzing a blend of engineering and biological disciplines that were traditionally separate. This slowly evolving domain involves the layer-by-layer construction of living tissue scaffolds that have applications spanning from drug testing to organ transplantation. As the healthcare sector embraces this breakthrough technology, understanding the intricacies of 3D cell printing becomes paramount for students, researchers, and professionals alike. Emerging as a cornerstone of regenerative medicine, it carries the promise of designing and fabricating tissues tailored to individual patients, thereby revolutionizing personalized healthcare.
Defining 3D Cell Printing
To truly grasp the innovative potential of 3D cell printing, we must first define what it encompasses. At its core, 3D cell printing involves the deposition of cells, biomaterials, and various bioactive factors in a precise manner to construct three-dimensional structures that closely mimic natural tissues. This bespoke manufacturing process employs specialized printers and 'bio-inks' made from living cells and hydrogels. The result? Living tissues that can replicate the architecture and function of real organs to varying degrees, depending on the technique employed and the materials used.
Historical Context and Evolution
While the roots of 3D printing can be traced back to the 1980s with plastic and metal objects, the foray into biological materials took longer. The year 2000 saw the first inklings of 3D cell printing. With technological innovations advancing rapidly, institutions began exploring the potential to print biological materials. Over the next two decades, significant breakthroughs emerged—ranging from improved bio-ink formulations to enhanced printing methods. These advances fostered an environment where researchers could experiment, leading to notable successes in creating tissues that closely resemble those in the human body. Today, the history of 3D cell printing is a tapestry threaded with scientific discovery, innovative technology, and a vision for the future of medicine.
The Science Behind 3D Printing Technology
Diving into the science of 3D printing technology reveals a sophisticated interplay of several disciplines. Physics, materials science, cell biology, and engineering converge to facilitate the construction of complex tissue architectures. Some primary printing techniques include inkjet-based, extrusion-based, and laser-assisted technologies;
- Inkjet Printing: Utilizes thermal or piezoelectric nozzles to deposit bio-ink droplets onto a substrate.
- Extrusion-based Printing: Forces bio-ink through a nozzle, allowing for continuous material deposition.
- Laser-assisted Printing: Uses lasers to precisely position cells in a desired pattern, offering a high level of accuracy.
This technological foundation underpins the entire process of 3D printing cells. Each method has its strengths and challenges, often dictating the type of tissues that can be produced. For example, while inkjet printing provides rapid fabrication, it may restrict the types of cells that can be effectively printed. Conversely, extrusion-based techniques are more forgiving regarding the types of materials used, which can be crucial when designing complex tissues.
In summary, the interplay between various scientific fields fuels the growth of 3D cell printing, fostering remarkable innovation and exploration that are forging new pathways in biomedical applications.
"As 3D cell printing continues to evolve, it has the potential to reshape the future of medicine, addressing critical shortages in organ donation and personalization in treatment."
Embracing this complex yet thrilling technology enables researchers and healthcare professionals to visualize new solutions to age-old medical dilemmas. Understandably, as the field advances, its implications will resonate throughout the medical community, propelling discourse around ethics and application to the forefront.
Key Technologies in 3D Cell Printing
The landscape of 3D cell printing is continuously evolving, driven by key technological advancements that form the backbone of this innovative field. These technologies are not only foundational but also crucial for overcoming barriers faced in bioprinting, enhancing overall precision and efficacy. What makes this section essential is that it sheds light on the mechanisms enabling scientists to manipulate biological materials, shaping a future where customizable tissue solutions and intricate organ constructs can become a reality.
Bio-ink Development
Bio-ink serves as the lifeblood in the realm of 3D cell printing, acting as a medium that supports and sustains living cells during the printing process. The development of bio-inks is paramount as it directly affects cell viability and functionality after printing. Various materials are being explored to create bio-inks that can not only hold cells but also mimic the natural environment they thrive in.
A fundamental characteristic of bio-ink is its ability to gel and solidify post-printing, creating a stable structure for cell growth. One might say it’s like crafting a cozy nest for cells to flourish. The material composition can range from natural substances like collagen to synthetic polymers, each having advantages and drawbacks in terms of printability and biological compatibility. The ongoing research into bio-ink formulations looks promising, pushing us closer to enhancing cellular behavior and tissue functionality.
Printing Techniques and Methods
Various printing techniques are employed in 3D cell printing, each presenting distinct benefits and addressing specific needs within bioprinting.
Inkjet Printing
Inkjet printing is one of the most widely recognized methods in this field, appreciated for its precision and adaptability. This technique involves the deposition of tiny droplets of bio-ink onto a substrate. A significant contribution of inkjet printing lies in its capability to generate high-resolution structures with well-defined geometries.
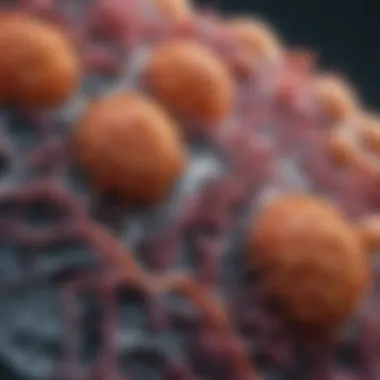
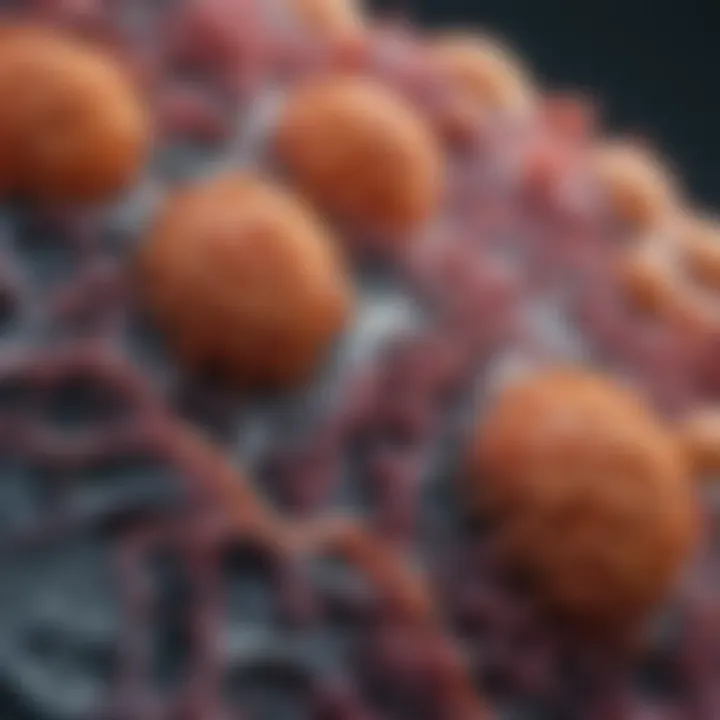
The key characteristic of inkjet printing is its non-contact process, which reduces shear stress on cells, thereby enhancing cell viability after printing. This method is particularly beneficial as it allows for the simultaneous printing of different types of cells, creating more complex tissue architectures. However, while it excels in resolution, inkjet printing may have limitations in the types of bio-inks it can handle, particularly those with high viscosity.
Extrusion-based Printing
Extrusion-based printing leverages a continuous flow of bio-ink through a nozzle, creating structures layer by layer. This technique plays a critical role in ensuring that larger structures can be printed more quickly compared to other methods. The relentless advancement in extrusion technology is pushing the boundaries of what is possible in 3D cell printing.
A notable advantage of extrusion-based printing is its ability to use a wide range of materials, including those with varying viscosities. This flexibility allows for the incorporation of cells within complex scaffolds, leading to better tissue strength and functionality. However, a unique challenge here lies in the control of shear stress; excessive force could damage delicate cells while printing, which necessitates careful calibration to maintain cell integrity.
Laser-assisted Printing
Laser-assisted printing is a cutting-edge technique that uses lasers to facilitate the precise placement of bio-ink on substrates. This process is particularly distinctive because it enables the formation of cell patterns with high spatial resolution, reflecting a significant innovation in the world of 3D printing.
The key feature of laser-assisted printing is its ability to create structures with extremely fine detail, which is crucial in replicating complex tissue architectures. This method can also allow for the incorporation of a variety of cell types, providing a versatile platform for biofabrication. However, a critical consideration is that the intense energy from lasers can potentially harm sensitive cellular structures, making it essential for continued advancements in this area to mitigate such risks.
Materials Used in 3D Cell Printing
The material selection for 3D cell printing is as essential as the technologies employed, with different classes offering unique benefits that tailor to specific applications.
Natural Polymers
Natural polymers, derived from biological sources, are vital in 3D cell printing due to their biocompatibility and inherent bioactivity. A primary aspect of natural polymers is their ability to encourage cell attachment and proliferation, mimicking the extracellular matrix found in tissues.
A key benefit is their biodegradability; materials such as alginate can be broken down by the body, making them ideal for temporary scaffolding in applications like tissue engineering. However, while they offer superb biological characteristics, natural polymers can have limitations in mechanical strength and stability, calling for a careful balance when selecting materials.
Synthetic Polymers
Synthetic polymers are engineered materials that provide a different set of advantages in 3D cell printing. The control over their physical and mechanical properties is one of their defining features. This allows researchers to tailor properties like elasticity and degradation rates, making them versatile for various applications.
A characteristic benefit of synthetic polymers is their ability to create stiffer structures, which can be beneficial for applications such as bone tissue engineering. However, concerns regarding biocompatibility often arise, necessitating the development of blends that can enhance both performance and biological interaction.
Hydrogels
Hydrogels have gained traction in 3D cell printing due to their high water content and ability to create a tissue-like environment. They offer a unique feature of mimicking natural tissue hydrophilicity, promoting cellular activities such as migration and proliferation within their matrix.
The key characteristic of hydrogels is their suitability for encapsulating living cells, making them indispensable in tissue engineering. While they boast excellent biocompatibility, the challenge remains with their mechanical strength, which often requires supplementary materials or additives to improve structural integrity.
By understanding these key technologies and materials, we can begin to appreciate the multi-faceted application and potential advancements in the field of 3D cell printing.
Applications of 3D Cell Printing
Understanding the applications of 3D cell printing serves as a crucial element in recognizing its significance to contemporary biotechnology. This innovative technology finds utility in various fields, especially in medicine, where its impact is notably profound. As researchers tap into the capacity of 3D printing, they open up novel avenues for medical treatments, enhance drug testing procedures, and even address pressing issues surrounding organ transplants. Thus, this section will explore three main areas where 3D cell printing not only shines but reshapes the future of health and healing.
Tissue Engineering
Tissue engineering stands out as one of the most transformative applications of 3D cell printing. By creating three-dimensional biological structures that mimic real tissue, this technology allows for advanced medical interventions.
Skin Regeneration
Skin regeneration is a prime example, as it showcases how 3D printing can enhance wound healing and repair. Traditional methods often leave scars or fail to integrate with native tissue. However, 3D-printed skin grafts can be tailored specifically to the patient's needs and characteristics.
The key characteristic of skin regeneration through 3D printing lies in the ability to produce constructs that closely resemble the natural layers of human skin. This specificity makes it a valuable choice in the field, as it paves the way for better integration and functional outcomes in patients. The unique feature of skin regeneration via 3D printing is its precision in layering skin cells, which supports better blood vessel formation and tissue perfusion. One clear advantage of this method is its potential to reduce recovery time and improve aesthetic results while minimizing graft rejection rates.
Cartilage Reconstruction
Cartilage reconstruction is another significant aspect of tissue engineering utilizing 3D cell printing. Cartilage is notoriously difficult to heal due to its avascular nature, making traditional repair methods often inadequate.
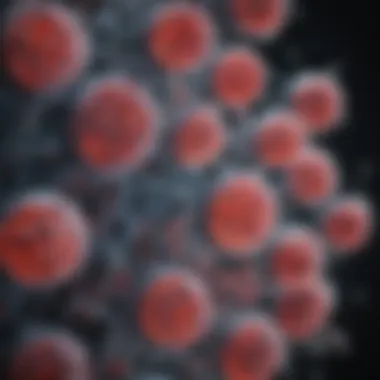
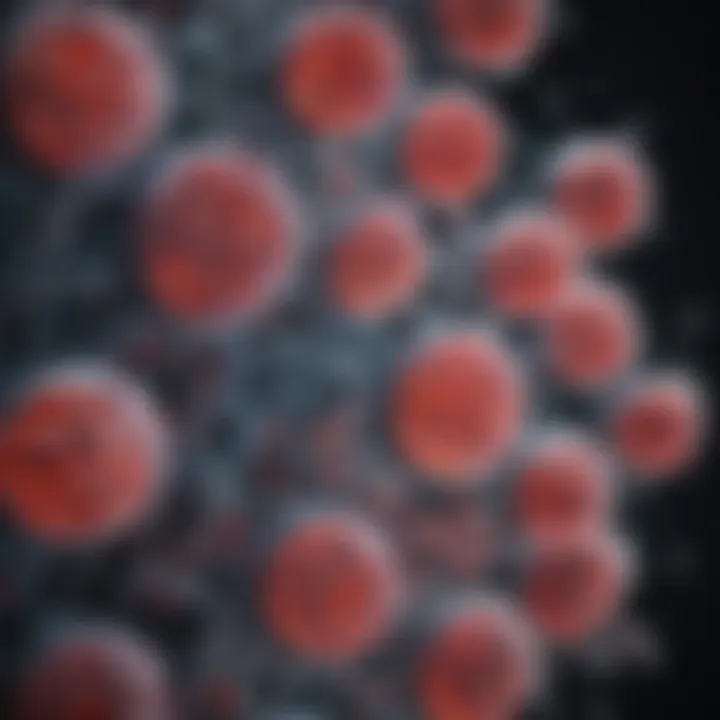
The key characteristic here is the intricate structure that can be achieved through 3D printing, which allows for the reproduction of the mechanical properties of cartilage. It becomes a beneficial option for treating injuries or degenerative diseases such as osteoarthritis. The unique feature of cartilage reconstruction through 3D printing lies in its customization, enabling the development of tailored grafts that match the patient's anatomical needs. Once again, the advantages are twofold: improved outcomes and the reduced risk of transplant rejection, enhancing patient safety.
Bone Tissue Engineering
Finally, bone tissue engineering via 3D printing offers remarkable promise. Traditional bone grafting techniques often encounter complications, such as limited availability of donor tissues or immune responses.
The key characteristic of this application is its ability to create bio-compatible scaffolds that support the growth of new bone cells. It becomes a popular choice because it has the potential to revolutionize orthopedic and reconstructive surgery. An advantage of using 3D printing in bone tissue engineering is the reduction of surgery times, as custom-made implants can be produced quickly, tailored to the specific dimensions required for each patient. However, one disadvantage could be the long-term stability and mechanical integrity needed to endure the daily stresses experienced by bone.
Pharmaceutical Testing and Drug Development
Moving beyond tissue engineering, the use of 3D cell printing dramatically influences pharmaceutical testing and drug development. By fabricating intricate cellular models, researchers can simulate human responses to drugs more accurately than ever before. This innovation in drug testing means that medications can be assessed for efficacy and safety on these printed models before reaching trial stages in human subjects. The advantages here are substantive: reduced drug development costs, more relevant testing environments, and ultimately a more efficient path to new therapies.
Organ Printing and Transplantation
Organ printing, particularly when it comes to transplantation, is one of the most exciting frontiers in this field.
Current Status
As of now, the current status of organ printing remains ambitious yet hopeful. While scientists have made strides in developing small organs and organoids, such as livers or kidneys, full-scale organ printing is still in the early stages of experimentation. The key characteristic of this application is the potential to combat transplant shortages. It's a beneficial aspect of this technology, promising to reduce wait times for patients in need. The unique feature of current organ printing efforts is the combination of advanced biomaterials and cellular precision to create functional tissues. Nevertheless, challenges abound, including vascularization and long-term functionality post-implementation, which could hinder practical applications.
Future Prospects
Looking ahead, the future prospects of organ printing are tantalizing. Researchers are actively exploring the integration of 3D-printed organs with existing biological systems, aiming for true functionality. The key characteristic of this direction encompasses scalability and personalization. This growth trajectory is beneficial as it could lead to mass production of organs tailored specifically for patients. The potential for advancements in regenerative medicine rises, yet the path also faces hurdles such as high production costs and ethical questions surrounding organ generation.
In summary, the applications of 3D cell printing are vast and varied, provoking thought and admiration in equal measure. With continuous developments, the trajectory clear: this technology holds the keys to policy-shifting advancements in healthcare, drug development, and beyond.
Challenges in 3D Cell Printing
The journey of 3D cell printing is not all smooth sailing. While the advancements in this field have spurred excitement, they come with a labyrinth of challenges that can hinder progress and application. Understanding these challenges is vital, as they shape the very framework of what can be achieved in bioprinting. This section fleshes out the technical limitations, biological hurdles, and the regulatory and ethical concerns that loom over the technology.
Technical and Engineering Limits
When it comes to the technical side, the limitations can often feel like a double-edged sword. The very nature of biological material can present a myriad of difficulties during the printing process. One main issue is resolution. Printing with cells requires precision; too coarse a resolution can inhibit cellular behavior, leading to structures that don't function as intended.
Moreover, maintaining the structural integrity of printed cells is a tall order. As much as engineers have made strides in this area, the stability and durability of complex living tissues during and post-printing remains a challenge. This instability can thwart deployments in clinical settings where reliability is paramount. Engineers are continually innovating, but as progress marches on, these constraints remind us that we have miles to go.
Biological Challenges
Cell Viability
Delving into biological challenges, cell viability stands out as a crucial aspect. This refers to the proportion of live cells within a printed structure. Aiming for high cell viability is not just an afterthought; it is the linchpin of successful tissue engineering. If the cells do not survive the printing process, then all efforts are in vain.
The key characteristic of cell viability is its direct impact on functionality. High cell viability typically results in better tissue integration and performance. This trait is particularly beneficial for applications aiming at regenerative medicine, where the entire goal revolves around reintegrating living tissues back into the human body.
However, achieving this level of cell viability comes with its unique set of hurdles. For one, cells can be sensitive to their environment; factors like temperature and shear stress during printing can adversely affect their ability to thrive. Thus, enhancing cell viability while navigating these environmental pitfalls is a critical area of research and innovation.
Functional Integration
Functional integration is another cornerstone of biological challenges in cell printing. This concept pertains to how well the printed cells integrate with surrounding tissues and systems. A key characteristic here is its influence on the overall effectiveness of the bioprinted structure. The more seamlessly a printed tissue integrates with existing biological systems, the higher the odds it will serve its intended purpose—be it regenerating a damaged area or enhancing drug delivery.
One unique feature of functional integration is its variability depending on the types of cells and biomaterials used. Generally, using native-like extracellular matrices enhances integration but can complicate the printing process. This balancing act can lead to advantages such as improved patient outcomes, but if not handled well, it could result in significant setbacks.
Regulatory and Ethical Considerations
In the realm of 3D cell printing, regulatory and ethical considerations play a pivotal role in shaping how the technology is adopted and integrated into healthcare. The question of how to govern this innovation is complex, stemming from concerns around safety, efficacy, and long-term repercussions. Current frameworks often lag behind technological advancements, leading to ambiguity and uncertainty.
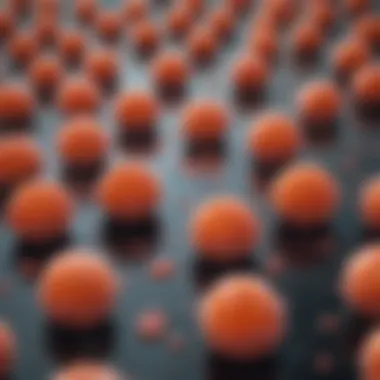
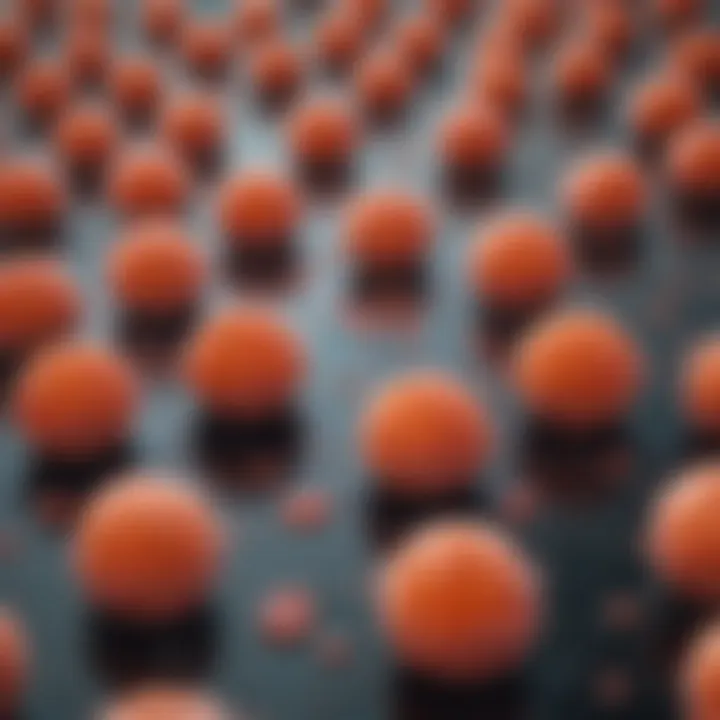
Ethically, the ability to create living tissues raises profound philosophical questions. For instance, where do we draw the line in tissue creation? The implications of creating organs or tissues for transplant purposes, while exciting, also bring about ethical dilemmas regarding consent, equity, and access to such life-saving technologies. As the technology progresses, addressing these hurdles will be essential in ensuring responsible and equitable use.
"Navigating the labyrinth of challenges in 3D cell printing is essential for unlocking its full potential in revolutionizing healthcare."
In summary, while the road ahead is filled with intricate challenges, the continual exploration of these issues sets the stage for future breakthroughs in 3D cell printing. Understanding and addressing these hurdles serves not only the scientific community but also the broader fabric of society impacted by these innovations.
Future Directions in 3D Cell Printing
The trajectory of 3D cell printing is poised for remarkable transformations, driven by technological advancements and theoretical explorations. This section aims to highlight the emerging trends that shape the future, underscore the significance of interdisciplinary approaches, and propose a long-term vision for the integration of 3D printing in the biomedical arena.
Emerging Trends
As the field of 3D cell printing matures, distinct trends are surfacing. Personalized medicine is gaining traction, enabling tailor-made treatments for individuals based on their unique biological makeup. This movement signifies a shift from the one-size-fits-all approach to more individualized therapies, particularly in oncology and regenerative medicine.
Additionally, the incorporation of biomaterials with enhanced properties is becoming increasingly prevalent. Materials that closely mimic the natural extracellular matrix can significantly improve cell attachment and growth, thus creating more realistic tissue structures. This trend not only improves the fidelity of printed tissues but also amplifies their functionality in clinical applications.
Moreover, there's a growing emphasis on sustainability within 3D printing practices. Researchers are investigating biodegradable materials that reduce environmental impact, while also ensuring that these materials effectively support cellular activities. This intertwining of ecological considerations is becoming a driving force in the development of future 3D cell printing technologies.
Interdisciplinary Approaches
Collaboration Across Fields
Collaboration across different scientific domains stands as a cornerstone for advancing 3D printing technologies. Professionals in biology, engineering, material science, and computer science are merging their expertise to tackle complex challenges in the field. This fusion of knowledge leads to innovative solutions that wouldn't be possible within siloed approaches.
One specific aspect of this collaboration is the development of specialized bioreactors that provide ideal conditions for printed tissues to thrive. By leveraging mechanical engineering principles, researchers can design environments that mimic physiological conditions, promoting cell viability and functionality. This interdisciplinary commitment enriches the field and catalyzes breakthroughs that pave the way toward practical applications in medicine.
Impact of Artificial Intelligence
Artificial intelligence is rapidly finding its role in optimizing 3D cell printing processes. Through machine learning algorithms, researchers can analyze vast datasets to identify patterns and predict optimal printing conditions for various cells and materials. AI's predictive capabilities offer a pathway to streamline the design process of bioinks and improve the overall efficiency of 3D printing.
The adaptability of AI in clinical settings also presents exciting opportunities. For instance, AI can assist in the real-time monitoring of cell behavior post-printing, facilitating immediate adjustments to enhance outcomes. However, while AI brings numerous advantages such as precision and speed, it introduces challenges concerning ethical considerations, particularly concerning data privacy and the need for transparent algorithmic decision-making.
Long-term Vision
Looking ahead, the vision for 3D cell printing includes not only achieving functional human tissues that can integrate with existing biological systems but also revolutionizing organ transplantation. The ultimate goal is the capacity to print complex organs on-demand, drastically reducing waiting lists and the risks associated with transplant rejection.
In order to realize this vision, continued investment in research and development is crucial. Stakeholders across sectors must come together, forging partnerships that encourage innovation while addressing ethical concerns associated with the application of such technologies in healthcare.
"The future of 3D cell printing is not merely a technological advancement but a profound shift in how we think about treatment and healing."
As society grapples with the implications of these advancements, ongoing dialogue among stakeholders will ensure that the benefits of 3D printing are harnessed in a responsible way, enhancing both capabilities in biomedicine and overall patient care.
Finale
The exploration of 3D printing of cells sheds light on a frontier of biotechnology that is not just about creating structures but also about reshaping the very fabric of medicine and health. This journey into the complex interplay between technology and biology reveals how innovations in cell printing are opening new avenues for treatment and care.
Summarizing Insights
As we navigate through the layers of 3D printing technology, a few key insights emerge. First, it’s clear that this realm is multi-faceted, drawing on a blend of engineering prowess and biological understanding. From bio-ink development to the intricate printing methods, each element plays a pivotal role in ensuring that the printed cells not only replicate expected structures but also function as living tissues.
Moreover, the implications of these advances stretch far and wide. We see potential applications in skin regeneration for burn victims, the creation of cartilage for those suffering from joint issues, and even the ambitious goal of organ printing. Each small success in this space is a step toward revolutionizing how we perceive medical treatments.
The Role of 3D Cell Printing in Advancing Biotechnology
It cannot be understated: 3D cell printing stands at the crossroads of innovation and practical application. The method offers a robust platform for scientists and researchers to prototype and extend biological structures in ways that were previously thought to be relics of science fiction. For instance, the development of fully functional organs may soon transition from dream to reality.
The integration of artificial intelligence adds a further layer of sophistication, allowing for more precise design and fabrication processes. By simulating biological responses within printed tissues, we can refine our approaches and tailor therapies to individual needs, enhancing patient outcomes dramatically.
“3D cell printing represents more than a mere technological advancement; it embodies a shift in our understanding of biological potential.”
In essence, as we synthesize the knowledge shared within this article, it becomes evident that 3D cell printing is not merely a tool. It is an evolving paradigm that may alter treatments, enhance understanding of biological processes, and profoundly influence the trajectory of healthcare. The road ahead is full of challenges, but the promise it holds is unmistakably bright.