Carbon Capture and Reuse for a Sustainable Future

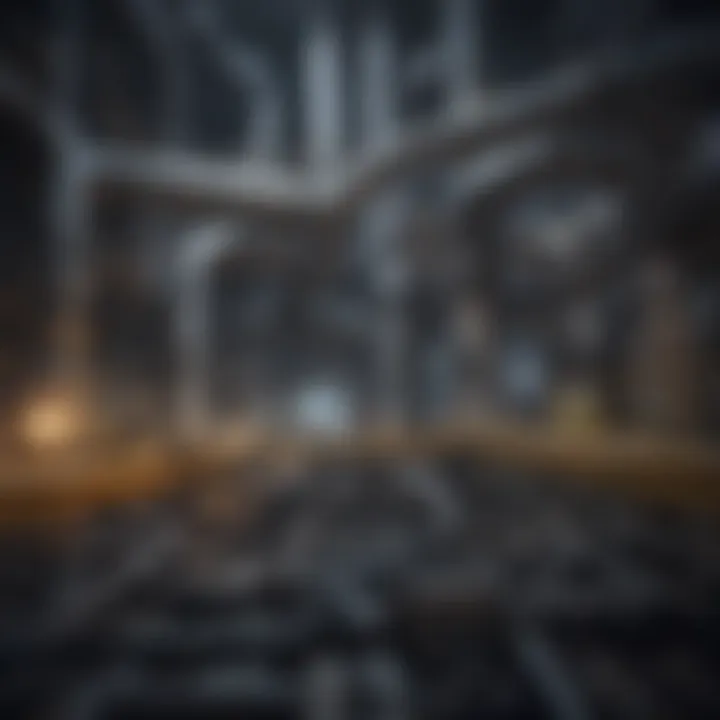
Intro
As the world grapples with the urgent need to address climate change, carbon capture and reuse technologies have emerged as a focal point in the conversation about creating sustainable solutions. Capturing carbon dioxide from the atmosphere and repurposing it not only tackles the rising levels of greenhouse gases but also opens up avenues for innovative applications that bolster environmental sustainability. The relevance of this topic cannot be understated, as it intermeshes with pressing global challenges, from pollution to resource depletion.
Given the complexity of climate dynamics, it’s essential to navigate through the mechanisms, technologies, and practical applications surrounding carbon capture. By diving into these facets, we gain a clearer understanding of how captured carbon can be effectively reused, consequently reducing its presence in the atmosphere.
Moreover, the economic viability of these methods can’t be left in the rearview mirror, as the potential benefits present a compelling case for investment and innovation. From enhancing industrial processes to developing new materials, the pathways for carbon reuse are as diverse as they are promising.
In this article, we will unpack the significant findings accumulated from ongoing research, shedding light on the state of carbon capture and reuse technologies. With insightful analyses, real-world examples, and expert contributions, we aim to cultivate a broadened perspective on a critical issue that affects us all.
Prolusion to Carbon Capture and Reuse
Carbon capture and reuse stands at the forefront of modern environmental discourse, offering remarkable potential in addressing climate change. As the scientific community and the general public alike grapple with the pressing urgency of rising greenhouse gas emissions, understanding the foundations and relevancy of carbon capture technologies has never been more critical. This section introduces the concept of carbon capture, elaborating on its mechanisms as well as establishing the need for such technologies in today’s context.
Defining Carbon Capture
At its core, carbon capture refers to the process of capturing carbon dioxide emissions—chiefly arising from industrial processes and power generation—before they enter the atmosphere. The technology aims to mitigate the environmental impact of these emissions by either storing the captured carbon or finding ways to recycle it for reuse.
This practice hinges on various mechanisms, ranging from physical separation methods to chemical reactions, which effectively trap carbon. The implementation of these techniques can lead to a significant reduction in the volume of carbon dioxide that contributes to global warming.
Key Points in Defining Carbon Capture:
- Nature of Process: Mostly targets emissions from fossil fuel sources.
- Method Diversity: Encompasses several technologies like direct air capture systems and pre-combustion processes.
- Global Initiative: A response to international agreements like the Paris Accord, which aim to limit global temperature rise.
Through this lens, carbon capture becomes not only a technological innovation but also a key component of a broader strategy to combat climate change, ensuring a healthier planet for generations to come.
The Need for Carbon Capture Technologies
In the current environmental landscape, the critical need for carbon capture technologies is underscored by alarming statistics. As industries ramp up production to meet global demands, emissions soar, further driving the phenomenon of climate change. The Intergovernmental Panel on Climate Change (IPCC) has indicated that curtailing carbon emissions is paramount to achieving a stable climate. Hence, carbon capture technologies emerge as non-negotiables in this transition.
However, the necessity goes beyond mere compliance. It also touches on ethical responsibilities toward future generations, and the potential to create new economic opportunities.
Elements Highlighting the Need for Carbon Capture:
- Rising Global Temperatures: The world is witnessing unprecedented weather patterns, linked directly to increased carbon emissions.
- Industrial Emissions: One of the largest culprits in climate change, requiring innovative solutions to reduce overall impact.
- Sustainable Practices: As global industries progressively seek sustainable methods, carbon capture fits seamlessly into a greener operational ethos.
"The implications of failing to address carbon emissions are stark. Without action, we risk not only ecological devastation, but societal and economic challenges as well."
In summary, the drive toward carbon capture technologies encapsulates urgency and innovation, urging a collective effort from scientists, policymakers, and industries to pivot towards sustainability. Through robust discussion and exploration detailed in this article, the necessity for advancing carbon capture technology alongside its socio-economic benefits will become increasingly evident.
Mechanisms of Carbon Capture
Understanding the mechanisms of carbon capture is fundamental in grasping how these technologies contribute to global sustainability efforts. The landscape of carbon capture technologies is diverse, offering various techniques that serve different industrial and environmental needs. Identifying the appropriate mechanism depending on the application can optimize efficiency and effectiveness in capturing carbon dioxide. Each method comes with unique benefits, but considerations like cost, implementation challenges, and energy consumption play a crucial role in determining practicality.
Physical Capture Methods
Physical capture methods rely on physical processes to separate carbon dioxide from other gases. Techniques such as adsorption and absorption are prime examples here. In adsorption, gases adhere to the surface of solid materials, while absorption involves the integration of gases into liquids. These methods are typically less energy-intensive compared to their chemical counterparts, making them an attractive option, especially for large-scale applications.
"Using physical methods can often be the first line of defense against excessive carbon emissions, allowing for quick and efficient captures before they reach the atmosphere."
The main advantage of physical capture is the efficiency of the process under certain conditions, such as high pressure and low temperatures. However, the challenge often lies in the need for extensive equipment and the requirement for continuous energy input to regenerate the materials used in the process.
Chemical Capture Methods
Chemical capture methods are known for their efficiency in extracting carbon dioxide via chemical reactions. This technique typically involves solvents that react with carbon dioxide to form stable compounds, which can then be processed or stored. For example, aqueous solutions containing amines react with CO2, leading to the formation of carbamates. This approach holds significant promise as it can handle large volumes of gas; however, it often requires more energy for solvent regeneration compared to physical methods.
Some key points regarding chemical capture include:
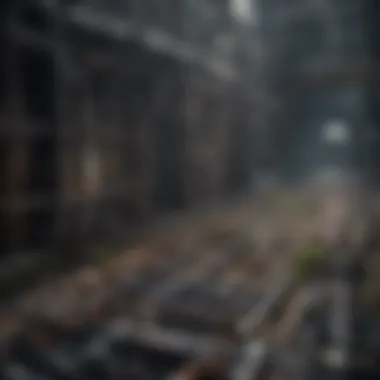
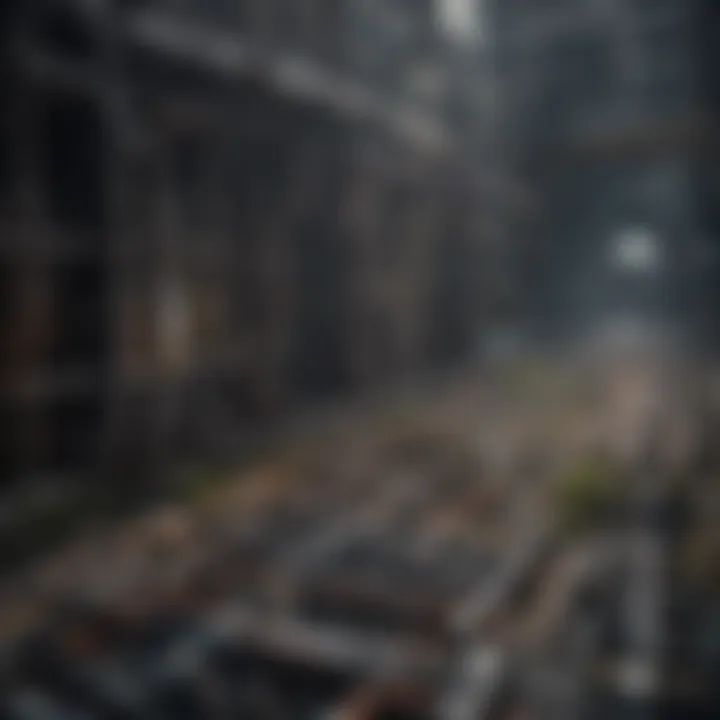
- Higher efficiency: Effectively captures higher concentrations of CO2.
- Specificity: Tailored chemical reactions can be designed for targeted applications.
- Heavier operational costs: Greater complexity results in higher costs, particularly related to energy requirements for solvent regeneration.
Biological Capture Techniques
Biological capture techniques leverage natural processes, such as photosynthesis, to sequester CO2. Plants, algae, and microorganisms play a pivotal role in this category. Algae, for example, can utilize carbon dioxide efficiently during photosynthesis, converting it into biomass. This biomass can then be harnessed for various applications, from biofuels to food products.
Benefits of biological capture techniques include:
- Environmental friendliness: Utilizing natural processes reduces reliance on artificial interventions.
- Dual benefits: Captured carbon can contribute to biomass production, offering a sustainable source of energy and materials.
- Scale limitations: While promising, these methods often face challenges in terms of scalability, which can hinder widespread implementation.
By examining these diverse mechanisms, we can gain insight into the potential pathways for capturing carbon effectively. The interplay between various approaches helps frame a more comprehensive perspective on how we might tackle the pressing challenges posed by rising atmospheric CO2 levels.
Current Technologies in Carbon Capture
Carbon capture is a vital step toward addressing the climate crisis. With the world’s reliance on fossil fuels, the need to mitigate carbon emissions has never been more pressing. Understanding current technologies in carbon capture not only reveals how we can curb greenhouse gas emissions but also illustrates the promising innovations that lie ahead. The benefits offered by these technologies, such as reducing atmospheric carbon dioxide levels, advancing sustainable practices, and driving economic growth, advocate for their importance in the ongoing battle against climate change.
Direct Air Capture Systems
Direct Air Capture (DAC) systems have emerged as a groundbreaking solution to remove CO2 directly from the atmosphere. These systems work by employing chemical processes to bind carbon dioxide in large volumes of ambient air. The captured CO2 can be either sequestered or reused in various industrial applications.
The importance of DAC lies in its versatility. It can be deployed anywhere, not limited by the proximity to emission sources, making it a flexible approach in a world of varying geographic landscapes. For instance, a project based in Switzerland known as Climeworks has made a significant impact by capturing CO2 from the air and subsequently redistributing it to greenhouses, enhancing plant growth.
Moreover, DAC represents a vital technology in achieving net-zero emissions. As it captures emissions from sources that are hard to decarbonize, it provides a complementary solution alongside reducing fossil fuel use.
Pre-Combustion and Post-Combustion Capture
In the energy and industrial sectors, pre-combustion and post-combustion capture methods play crucial roles in carbon management. Pre-combustion capture involves removing CO2 before the combustion of fossil fuels. This is often done by converting fossil fuels into hydrogen and CO2, separating them, and storing the CO2.
On the other hand, post-combustion capture takes place after fossil fuels have been burned. This technique utilizes solvents to absorb CO2 from flue gases produced during combustion. For instance, the Petra Nova project in Texas uses post-combustion methods to capture approximately 90% of CO2 emissions from a coal-fired power plant, showcasing how effective this approach can be.
The selection between these methods generally comes down to economic viability and technical feasibility. Pre-combustion may yield higher efficiencies, but the existing infrastructure might favor post-combustion solutions due to lower initial costs.
Emerging Technologies in Carbon Capture
The field of carbon capture is rapidly advancing, with exciting emerging technologies on the horizon. Innovations such as membrane technologies are being researched for their potential to improve capture efficiency while reducing energy costs. Membranes can selectively allow CO2 to pass through while blocking other gases, providing a compact solution for carbon capture systems.
Another avenue is the utilization of artificial intelligence (AI) in optimizing capture techniques. By leveraging AI, stakeholders can predict and manage operational efficiencies and maintenance needs, ultimately making carbon capture technologies more effective and accessible.
Furthermore, mineralization techniques, which convert CO2 into solid minerals, are gaining traction. This process not only captures carbon but also locks it away permanently, thereby reducing the amount of CO2 in the atmosphere considerably.
"Emerging technologies pave the way for a future where carbon capture isn't just a necessary evil, but an integrated part of our clean energy ecosystem."
In summary, understanding and investing in current technologies for carbon capture is paramount to combating climate change. Each of these innovations, from Direct Air Capture systems to the various advanced methods on the horizon, highlights the diverse pathways available for harnessing CO2 and rethinking our relationship with emissions.
By fostering innovation and embracing these technologies, we take significant strides toward a more sustainable future.
Reusing Captured Carbon
Reusing captured carbon is not just a buzzword in the realm of climate action; it’s a pivotal component in the journey towards sustainable solutions. The very essence of this practice nurtures the notion that waste can be transformed into a resource. At its core, carbon reuse seeks to mitigate the environmental footprint of carbon dioxide emissions by repurposing CO2 for beneficial uses. Given the pressing challenges posed by climate change, this approach offers significant advantages.
One of the primary benefits is the potential reduction in reliance on fossil fuels. Transforming captured carbon into fuels can assist in creating a circular economy, which thrives on sustainability. This reduces greenhouse gas emissions while providing alternatives to conventional energy sources. Additionally, effective carbon reuse can bolster industries by providing new avenues for innovation and economic growth.
So, what exactly does carbon reuse entail? It encompasses various processes that convert CO2 into usable products, thereby securing its place in a more sustainable future. The path towards reusing carbon is multi-faceted, and understanding each avenue is crucial.
Conversion to Fuels
The conversion of captured carbon into fuels represents a significant stride towards a cleaner energy landscape. Various methods exist to achieve this transformation, with the most notable ones being chemical conversion and biological processes.
- Chemical Conversion: This method converts CO2 into hydrocarbons through processes like Fischer-Tropsch synthesis. It effectively re-engineers captured carbon into synthetic fuels, such as gasoline and diesel. As a result, not only do we reduce atmospheric CO2, but we also produce fuels that can power existing infrastructure with minimal adjustments.
- Biological Processes: Microorganisms can also play a role in this conversion. Specific strains of algae can use CO2 in the process of photosynthesis, producing biofuels in return. This pathway not only captures carbon but promotes new biotechnological innovations.
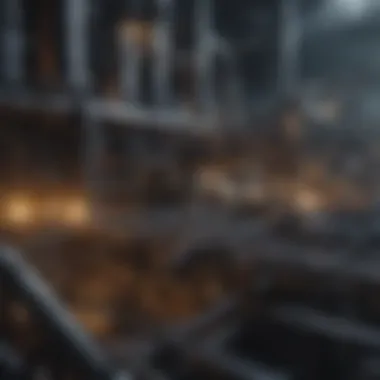
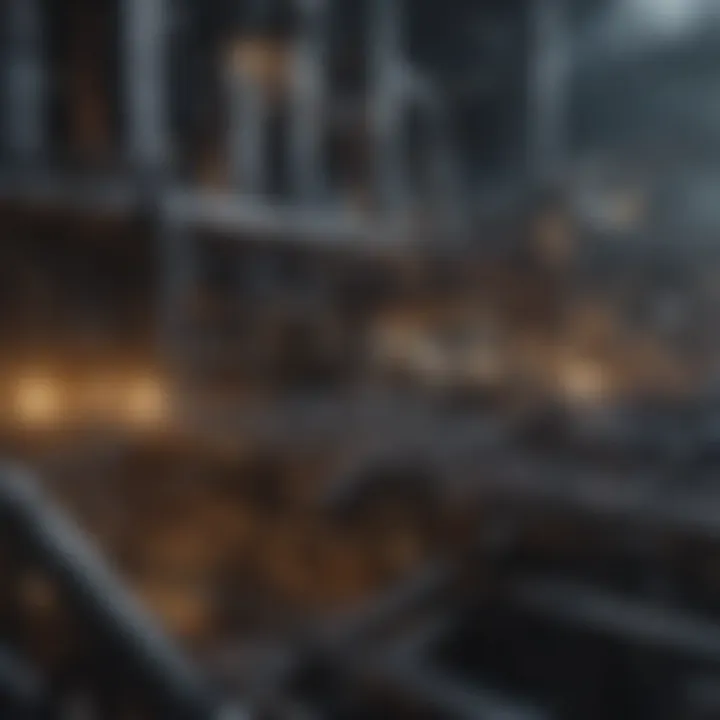
Ultimately, these avenues reshape how we perceive energy production and open doors for the widespread adoption of cleaner technologies.
Utilization in Chemical Processes
Another compelling avenue for reused carbon lies in its application in chemical processes. Various industries can leverage CO2 as a feedstock to manufacture a range of valuable chemicals, thereby turning what was once considered a pollutant into a commodity.
Take, for instance, the creation of methanol from CO2. Methanol is a versatile chemical used in many products, from plastics to antifreeze. By utilizing carbon as a raw material, companies can diminish their carbon footprint while supporting the transition to more sustainable practices.
Moreover, industries are increasingly investing in carbon capture technologies that enhance their processes further. The production of plastics using CO2 not only benefits manufacturers but can also result in reduced raw material costs.
The utilization of captured carbon thus serves as an industry game-changer, aligning economic incentives with environmental sustainability.
Stored Carbon in Industrial Applications
Finally, the concept of stored carbon in industrial applications leads to fascinating opportunities. Here, carbon is not reused immediately but rather sequestered in durable products. For instance, concrete and cement production can incorporate CO2, enhancing the strength of the materials while locking away carbon in the process.
- Concrete Production: Injecting CO2 into the curing process of concrete can produce stronger and more durable bricks, which is a crucial element in sustainable construction.
- Mineralization: Some firms are exploring ways to use captured carbon in mineralization processes, where CO2 reacts with naturally occurring minerals to form stable carbonates. This not only channels carbon into a stable, solid state but also creates products that can be used in construction, paving the way for a greener infrastructure.
In summary, reusing captured carbon hinges on innovation and strategic applications across various fields. The transformation of waste into resources illustrates a promising path to sustainability, not only benefiting the environment but also boosting economic potential in a rapidly evolving landscape.
Economic Implications of Carbon Capture and Reuse
The economic implications of carbon capture and reuse are not just a matter of environmental impact but also a key consideration for industries looking to align with sustainability goals while maintaining profitability. As global pressure mounts for companies to reduce their carbon footprints, understanding the financial aspects of carbon capture technologies becomes crucial. This section dives into the costs associated with these technologies and sheds light on the policies and incentives that can facilitate their adoption.
Cost Analysis of Carbon Capture Technologies
Analyzing the costs related to carbon capture technologies is essential for determining their feasibility.
- Initial Investment: Setting up carbon capture systems typically requires significant capital. Expenses can range widely, influenced by factors such as technology type, project scale, and plant location. For example, direct air capture systems require advanced infrastructure, which can lead to higher upfront costs.
- Operational Costs: Beyond initial investment, operational costs play a vital role. These costs encompass energy, maintenance, and staff necessary to run the systems efficiently. For instance, chemical capture methods might involve high energy usage, significantly affecting profitability.
- Long-Term Savings: While the initial and ongoing expenses can be daunting, investing in carbon capture may lead to long-term savings and benefits, particularly when factoring in potential future carbon taxes and regulatory fines. In places where emissions trading systems are in place, companies could see financial returns from captured carbon being sold as credits.
Capturing and reusing carbon is not merely an environmental initiative. It holds the potential to transform how industries operate economically.
Ultimately, a thorough cost-benefit analysis that accounts for both direct and indirect financial implications plays a significant role in deciding whether to implement carbon capture technologies.
Financial Incentives and Policies
To stimulate the growth of carbon capture and reuse technologies, several financial incentives and policies have emerged:
- Government Grants and Tax Credits: Various governments offer grants or tax credits for companies that invest in carbon capture initiatives. These incentives can reduce initial capital costs significantly, making implementation more attractive.
- Subsidies for Research and Development: Funding is directed towards groundbreaking research in technologies aimed at improving the efficiency and reducing the costs of carbon capture. Such subsidies can help advance methods that are not only economically viable but also environmentally beneficial.
- Emission Trading Systems: Policies that allow companies to trade emission credits can create a financial benefit for capturing carbon. By lowering their emissions, companies can either sell excess credits or avoid costly penalties associated with exceeding allowable emissions.
- International Agreements: Participation in global agreements focused on climate change can motivate nations to establish national policies that incentivize businesses to adopt carbon capture technologies. International collaboration can enhance funding opportunities through pools of resources and shared innovations.
Challenges in Carbon Capture Implementation
The implementation of carbon capture technologies is not without its hurdles. While the potential for reducing carbon emissions is immense, various challenges can impede progress. These barriers range from technical issues related to the capture processes to the regulatory landscapes that govern them. Understanding these challenges is crucial for stakeholders looking to advance carbon capture and reuse initiatives effectively.
Technical Challenges
Technical challenges present a significant roadblock in the adoption of carbon capture technologies. The primary difficulty lies in the efficiency of capturing carbon dioxide from various sources. Current technologies vary in their effectiveness based on the emissions source. For instance, carbon capture from a cement plant differs starkly from capturing emissions from a power generation facility.
The cost of amine-based chemical absorption, a common capture method, can be high. This expense arises from both the energy requirement to regenerate the amines and the necessity for constant maintenance of the equipment. Furthermore, scalability remains an issue. Many capture technologies are still in their infancy or are only tested at pilot scales. Transitioning them to larger, industrial applications poses numerous challenges, including the need for larger infrastructure and more extensive operational oversight.
Moreover, the behavior of captured carbon during storage is still a matter that scientists are exploring. There are potential risks of leakage or unintended consequences when injecting carbon into geological formations. Ongoing research is necessary to ensure these methods can be not only effective but also safe over the long term.
Regulatory and Policy Barriers
Navigating the regulatory landscape poses another challenge for carbon capture technologies. The policies governing greenhouse gas emissions vary greatly by region, and in many cases, they lack clarity on carbon capture and storage initiatives. Without specific guidelines, businesses may hesitate to invest in these technologies due to uncertainty about regulatory compliance and potential liabilities.
In addition, the lack of economic incentives hampers progress. While some governments offer financial aid and tax breaks, others provide little to no encouragement. This inconsistency can deter companies from pursuing carbon capture projects, as the associated costs may outweigh expected benefits without adequate support.
Regulatory frameworks must evolve alongside technological advancements. This means that policymakers need to work closely with researchers and industry players to craft regulations that support innovation while ensuring safety and environmental standards. Efforts to streamline the permitting process for carbon capture projects could greatly enhance implementation efficiency.
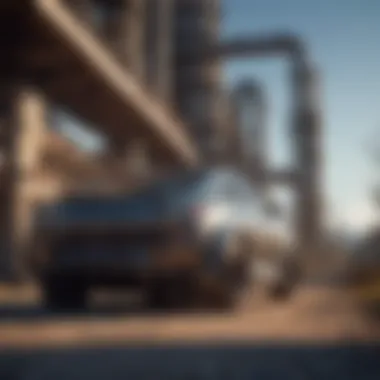
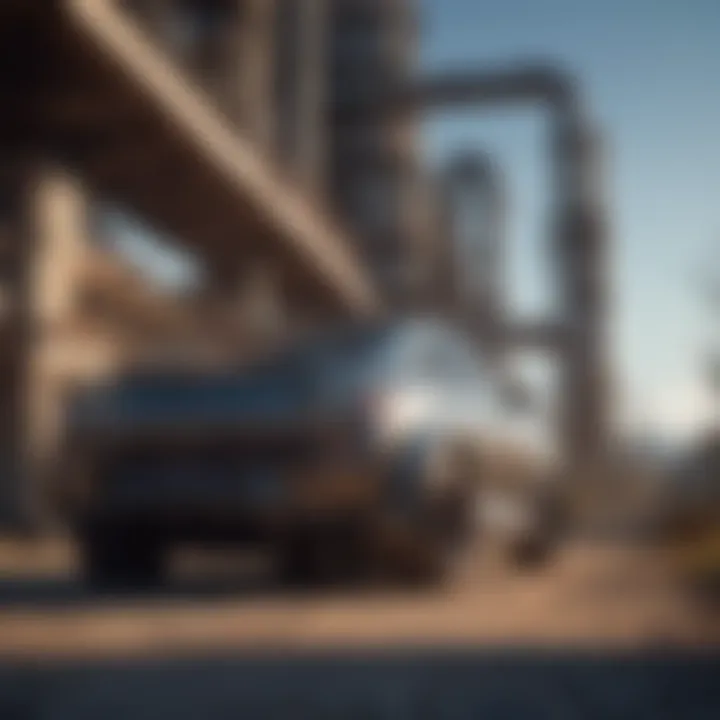
"Carbon capture can't just be a siloed solution; it requires an integrated approach to technology, policy, and public perception to succeed."
In summary, the successful implementation of carbon capture technologies hinges on overcoming both technical and regulatory challenges. Addressing these barriers is not merely an exercise in fixing problems but a critical step toward sustainability. Confronting these challenges head-on entails collaboration among industry, government, and research communities to pave the way for effective carbon capture and reuse practices.
Case Studies of Successful Carbon Capture Projects
Examining real-world applications of carbon capture provides an essential lens through which to appreciate the potential and effectiveness of these technologies. Through case studies, we get granular insights into how specific projects have not only faced various challenges but also achieved notable success. Analyzing these instances helps identify best practices, innovative strategies, and potential areas for improvements that could benefit future initiatives in carbon capture and reuse.
Industrial Scale Projects
One prominent example of industrial-scale carbon capture is found at the Boundary Dam Carbon Capture Project in Saskatchewan, Canada. This facility, which started operations in 2014, employs a post-combustion carbon capture system aimed at reducing emissions from a coal-fired power plant. The technology has proven to be effective, capturing approximately 1 million tons of carbon dioxide annually. This captured CO2 is then utilized for enhanced oil recovery, showcasing a dual-layer benefit of cutting emissions while simultaneously contributing to oil production.
In contrast, the Sleipner Project off the coast of Norway illustrates another robust example. Since 1996, this offshore storage project has been successfully injecting CO2 into deep geological formations under the North Sea. The project not only demonstrates long-term carbon storage but also serves as a vital research platform, enhancing our understanding of CO2 behavior in geological settings. Such projects illustrate how effective integration of carbon capture can align with existing energy systems while reducing the carbon footprint significantly.
Research and Development Initiatives
In addition to large-scale projects, numerous research and development initiatives are pushing the boundaries of what carbon capture technologies can achieve. The Carbon Capture Utilization and Storage (CCUS) program spearheaded by the U.S. Department of Energy is a critical research endeavor aimed at innovating ways to capture and store carbon. Through various pilot projects, they are exploring new materials and methods that could increase capture efficiency and reduce costs.
Another noteworthy initiative is the NET Power Project in Texas. This groundbreaking endeavor is aiming to demonstrate a new type of natural gas power plant that not only captures carbon but generates energy at a lower carbon intensity. By utilizing a novel thermodynamic cycle, this project seeks to revolutionize the way carbon capture can fit seamlessly into energy generation without compromising efficiency or economic viability.
"Real-world applications serve as living laboratories, offering invaluable data and lessons that can steer future advancements in carbon capture technologies."
In essence, successful case studies like Boundary Dam, Sleipner, and ongoing research initiatives embody the complexities and potential pathways forward in carbon capture and reuse. They remind us that capturing carbon is not merely a theoretical exercise but a feasible and necessary avenue toward a more sustainable future.
Future Directions in Carbon Capture and Reuse
The field of carbon capture and reuse is a rapidly evolving arena. It holds promise not just for combating climate change, but also for driving economic opportunities and innovation. As we turn our attention to the future, several critical elements beckon exploration. Not only can advancements in technology fundamentally alter how we approach carbon emissions, but they can also enhance the viability of captured carbon in various applications.
The importance of focusing on future directions in this domain is paramount. It opens pathways for newer techniques that could untangle the challenges of existing methods. Moreover, it aligns with global sustainability goals, pushing the envelope towards more effective carbon management strategies. This section aims to elaborate on two main threads: Technological Innovations on the Horizon and Potential Influence on Global Climate Policies.
Technological Innovations on the Horizon
Innovations in technology are central to the evolution of carbon capture strategies. As more entities invest in R&D, we see a surge of ideas that could redefine current paradigms.
- Advancements in Direct Air Capture: New pathways are emerging that enhance efficiency and reduce costs. Systems are becoming more compact and capable of capturing CO2 with minimal energy input. For example, methods utilizing sorbents made from abundant materials are being developed, promising better performance without heavy reliance on rare resources.
- Integration with Renewable Energy: The coupling of carbon capture systems with renewable energy sources is gaining traction. Utilizing excess energy from solar or wind can power carbon capture operations, making the process not only greener but also more sustainable in the long run.
- Artificial Photosynthesis: A fascinating direction is the mimicry of natural processes. Researchers are working on technologies that imitate photosynthesis, potentially producing useful fuels while absorbing CO2.
- Revolutionary Utilization Approaches: Concepts are being tested where captured carbon could be transformed into a wider range of products, from plastics to construction materials. This offers a dual advantage: reducing emissions while providing valuable commodities.
The amalgamation of these advancements heralds a new era where carbon capture becomes not just a means for reducing atmospheric CO2 but also a way to create valuable resources.
Potential Influence on Global Climate Policies
The trajectory of carbon capture and reuse has far-reaching implications for global climate governance. Governments and international bodies are increasingly recognizing the necessity of incorporating such technologies into their climate action frameworks.
- Policy Incentives: Countries can stimulate growth in the carbon capture industry through tax breaks and subsidies. Policies that reward innovation will be crucial, especially for fostering grassroots solutions while encouraging large-scale implementations.
- International Collaboration: Climate change knows no borders. Collaborative initiatives among nations can lead to shared technology, investment resources, and knowledge. Such partnerships might be the key for developing nations that struggle to footing the bill for R&D.
- Setting Clear Targets: As carbon capture technologies gain momentum, establishing clear international targets for carbon reduction can boost investments. Targets can guide technology development and ensure accountability among nations.
"The future of carbon capture technologies isn’t just a tale of emissions control, but an intertwined story of economic revitalization and international cooperation."
Ultimately, the potential influence of these innovations on policy structures can lead to a brighter and more sustainable future for our planet. As we forge ahead, keeping an eye on these pathways will be essential in crafting effective strategies for global climate action.
Closure
The Importance of Carbon Capture and Reuse in Climate Action
In the overarching narrative of combating climate change, carbon capture and reuse stands as not just a technological innovation but a pivotal strategy. This method addresses the pressing challenge of CO2 emissions head-on, recognizing that merely reducing emissions is not enough. Rather, it proposes a more dynamic approach — capturing carbon dioxide from the atmosphere and transforming it into valuable resources. The potential of this dual-purpose solution cannot be overstated.
First and foremost, carbon capture provides a pathway to mitigate the escalating levels of carbon in our atmosphere. As emissions from industrial processes and transportation rise, the urgency for effective solutions intensifies. Harnessing technologies that can trap this carbon represents a significant step towards reducing the greenhouse gases that contribute to global warming.
The benefits extend beyond merely blocking CO2 from entering the atmosphere. By reusing captured carbon in various industries, we can foster an economy that values sustainability. For example, when carbon dioxide is converted into fuels or used in chemical production, it effectively closes the carbon loop, rendering previously wasted emissions into materials that can contribute positively to our economy.
Moreover, this approach aligns with global climate policies aiming for net-zero emissions by mid-century. Carbon capture and reuse technologies not only comply with these commitments but also enhance the adaptability of existing infrastructures. They offer industries a chance to innovate within their current operations rather than overhauling entire systems, which can often be costly and time-consuming.
Here are several essential aspects regarding the importance of this technology:
- Resource Efficiency: Utilizing captured carbon can help industries save on material costs, reducing overall dependence on fossil fuels.
- Job Creation: These technologies can pave the way for new green jobs, fostering economic growth while addressing climate issues.
- Policy Alignment: By integrating with existing frameworks for emissions reductions, carbon capture technologies have the potential to ease the transition for sectors that are typically harder to decarbonize.
"The transition to a sustainable future cannot happen overnight; however, carbon capture and reuse provides a feasible method for gradual improvements while keeping industries operational."