Understanding Carrier Gases in Gas Chromatography
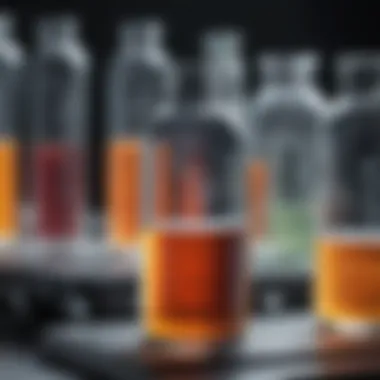
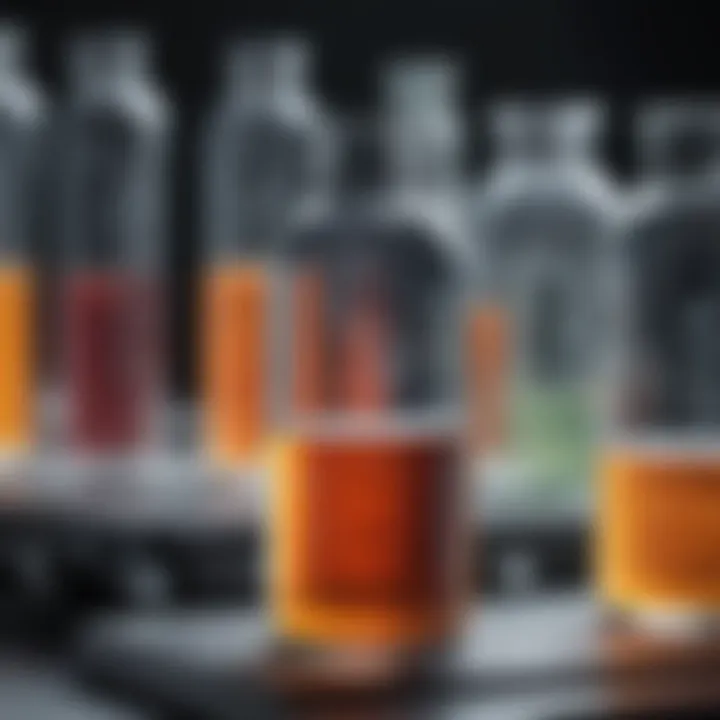
Intro
Gas chromatography is a fundamental technique in analytical chemistry, widely used for separating and analyzing compounds. Central to its functionality are carrier gases, which serve as the medium through which the sample mixture travels through the system. The importance of understanding these gases cannot be overstated, as they influence separation efficiency, resolution, and sensitivity of the analysis.
This article delves into the various aspects of carrier gases in gas chromatography. It will cover the types of carrier gases, their properties, effects on chromatographic performance, and best practices for their utilization in laboratory settings. By providing a thorough examination, this piece aims to equip both novices and experienced professionals with the knowledge necessary to optimize their chromatography procedures.
Research Highlights
Overview of Key Findings
Carrier gases play a pivotal role in the chromatography process. Parameters such as viscosity, density, and thermal conductivity of the carrier gas can significantly affect the separation efficiency. Among the commonly used carrier gases are helium, nitrogen, and hydrogen. Each gas has distinct properties that make it suitable for specific applications in the chromatographic analysis.
Significance of the Research
Understanding the choice of carrier gases not only enhances the resolution of individual components but also plays a crucial part in influencing the overall sensitivity of the detection methods used. Optimizing these gases can lead to improved analytical results and, consequently, better decision-making based on the obtained data.
The selection of an ideal carrier gas is essential for achieving optimal outcomes in gas chromatography, influencing both separation quality and analysis speed.
Types of Carrier Gases
- Helium
- Nitrogen
- Hydrogen
- Carbon Dioxide
- Historically preferred for its inert nature.
- Provides high efficiency in separating volatile compounds.
- Cost-effective and widely available.
- May cause lower resolution compared to helium.
- Offers high effciency and fast analyses.
- Safety concerns due to flammability and handling issues.
- Less common but useful in specific applications.
- Non-toxic and environmentally friendly.
Properties of Carrier Gases
Carrier gases must exhibit certain properties for effective performance in gas chromatography:
- Inertness: The gas should not react with sample components.
- Consistency: Delivering a uniform flow rate and pressure.
- Thermal Conducivity: Affects heat transfer in the system.
- Viscosity: Influences the efficiency of mass transfer.
End
In summary, carrier gases in gas chromatography are not mere technicalities but fundamental components that profoundly influence analytical outcomes. Understanding their characteristics and selection criteria is essential for any proficient practitioner in the field of analytical chemistry. This knowledge not only aids in improving methods but also in pushing the boundaries of what is achievable within gas chromatography.
Intro to Gas Chromatography
Gas chromatography is a crucial analytical technique widely used in various fields such as chemistry, biology, and environmental science. Its importance stems from its ability to separate and analyze chemical compounds in a mixture with high precision and efficiency. This section serves as an entry point into understanding the intricacies of the method and lays the groundwork for a deeper exploration of carrier gases, which play an essential role in the chromatographic process.
The significance of gas chromatography lies in its applications. It is employed in testing air quality, analyzing food and beverages, and conducting forensic investigations, among others. Such versatility comes from its capability to handle volatile compounds effectively, making it indispensable for both qualitative and quantitative analyses. The reliability of this technique relies heavily on the carrier gas, influencing performance metrics like resolution and sensitivity. As readers delve into the details of gas chromatography, they will appreciate not only its operational principles but also the nuances of applying the right carrier gas to achieve optimal results.
What is Gas Chromatography?
Gas chromatography is defined as a method used to separate and analyze compounds that can be vaporized without decomposition. In this technique, a sample is injected into a column where the separation occurs. The column is packed with a stationary phase, and a carrier gas serves as the mobile phase.
The separation of compounds occurs due to differences in their affinity towards the stationary phase and their volatility. When the sample mixes with the carrier gas and passes through the column, components of the sample travel at different rates based on their interactions with the stationary phase.
The resulting output, a chromatogram, visually represents the separation of compounds. Peaks on the chromatogram correspond to individual components, providing insight into their identity and quantity. The precision of gas chromatography makes it a favored method for analytes ranging from small molecules to larger volatile organic compounds.
Historical Development
The development of gas chromatography can be traced back to the mid-20th century. Although early forms of chromatography existed before then, it was in 1952 that Archer Martin and Richard L. M. Synge introduced a more refined version using gas as the mobile phase. This innovation set the foundation for what has become a standard method in analytical laboratories worldwide.
Initially, gas chromatography was limited in its application due to technological constraints. However, with advancements in detector technology, such as flame ionization detectors and mass spectrometers, the technique evolved significantly during the late 20th century. These enhancements allowed for increased sensitivity and the ability to analyze more complex mixtures.
As the methodology progressed, gas chromatography became a staple in various industries. Its integration into routine analytical procedures demonstrates its enduring relevance and adaptability in the face of evolving scientific challenges. Today, it remains at the forefront of analytical chemistry, continuously evolving through the incorporation of modern technology and techniques.
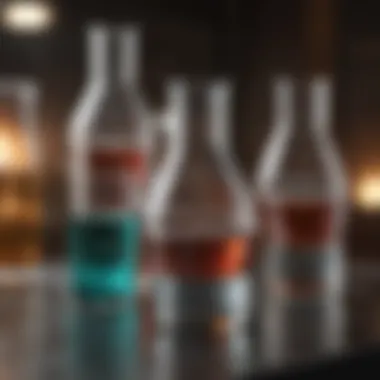
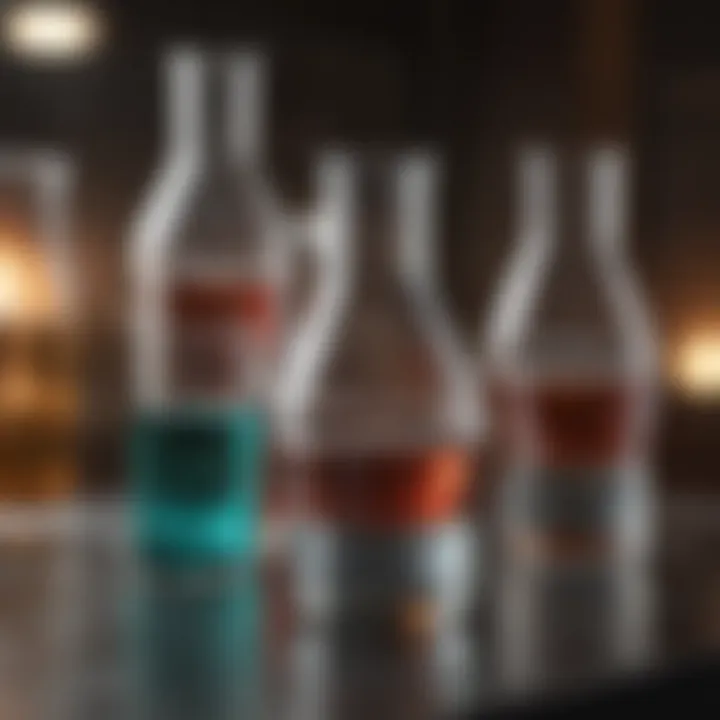
Fundamentals of Carrier Gases
Carrier gases are a fundamental component in gas chromatography. Their selection influences the overall effectiveness and efficiency of the separation process. Understanding carrier gases allows researchers and professionals to optimize their chromatographic techniques, leading to more accurate and reliable analytical results.
Definition of Carrier Gas
A carrier gas in gas chromatography is an inert gas that transports the sample through the chromatographic system. This gas does not react with the analytes. Common examples include helium, nitrogen, hydrogen, and carbon dioxide. The choice of carrier gas can affect resolution, sensitivity, and the time it takes for the analytes to pass through the column.
In practice, carrier gases must meet specific standards of purity to avoid contamination or interference with the analysis. In analytical chemistry, where precision is crucial, understanding the definition and function of carrier gases cannot be underestimated.
Role in Gas Chromatography
The role of carrier gases in gas chromatography is multi-faceted.
- Transport Function: The primary role is to carry the vaporized sample through the column. An effective carrier gas must provide a uniform flow and minimize pressure fluctuations.
- Influencing Retention: Different carrier gases interact differently with the stationary phase of the column, affecting the retention time of various compounds. This influences the resolution and separation of analytes.
- Temperature Management: Carrier gases can help manage the temperature within the chromatography system. For instance, certain gases can maintain stable thermal conditions during the analysis, which is critical for consistent results.
- Impact on Sensitivity: The choice of carrier gas can also impact the overall sensitivity of the detection method. Certain gases can improve the detector response, leading to better identification and quantification of analytes.
The role of carrier gases is crucial in determining both the qualitative and quantitative aspects of gas chromatography. Their characteristics may dictate success or failure in analytical outcomes.
Ultimately, a deep understanding of these fundamental aspects of carrier gases is essential for anyone involved in gas chromatography. By selecting the correct gas, professionals can significantly enhance their analytical capabilities.
Types of Carrier Gases
Understanding the types of carrier gases is crucial in gas chromatography as they directly influence the separation efficiency of analytes. Each type of carrier gas offers unique properties, costs, and advantages that can affect the analytical result. Knowing these differences helps researchers and technicians make educated decisions regarding the optimal carrier gas for specific applications.
Helium
Helium is often regarded as the gold standard in gas chromatography. Its properties make it an ideal choice for many applications. Helium has a low viscosity, allowing for a faster mass transfer and improved separation efficiency. Additionally, it is chemically inert, meaning it will not react with the sample or the stationary phase, ensuring that the integrity of the analysis is maintained. This inertness is vital especially when working with sensitive analytes that could degrade or alter due to reactions with the carrier gas.
However, helium is also more expensive than other options. This can be a significant factor, especially for laboratories that conduct high volumes of analysis. Its supply can also be sporadic, which may contribute to workflow disruptions.
Nitrogen
Nitrogen presents an economical alternative to helium. It is not as efficient as helium in terms of separation speed but offers good performance for many types of analyses. Its high availability and lower cost make it appealing for routine work.
One must consider the separation characteristics of nitrogen. It has a higher viscosity compared to helium, which can lead to slower mass transfer. Thus, larger sample volumes might be necessary to achieve similar sensitivities as with helium. Additionally, nitrogen can play a role in the chromatographic parameters, like retention time and resolution. Careful optimization of method conditions is essential when using nitrogen as a carrier gas.
Hydrogen
Hydrogen is another alternative that has been gaining traction within the chromatography community. It provides high efficiency and can lower analysis times. Like helium, hydrogen is also very low in viscosity. This trait allows for faster separations and shorter column lengths, which can contribute to minimized analysis costs.
Nonetheless, working with hydrogen poses specific safety risks due to its flammability. Laboratories utilizing hydrogen must adhere to strict safety protocols and ensure proper ventilation. Therefore, the choice of hydrogen as a carrier gas is often contingent upon the laboratory's safety infrastructure.
Carbon Dioxide
Carbon dioxide is less common but serves specific applications effectively. It is a moderately polar gas, which means it can enhance the interaction between the carrier gas and polar compounds. This trait can improve the separation of certain compounds that more inert gases may not adequately resolve.
CO2 can also aid in the analysis of volatile and thermally labile compounds due to its low boiling point. However, its utility in conventional gas chromatography is limited compared to helium, nitrogen, and hydrogen. Researchers must evaluate the specific requirements of their analyses to determine if CO2 provides a clear advantage.
In summary, each carrier gas has distinct advantages and disadvantages, and understanding these can help optimize gas chromatography results. The appropriate choice of carrier gas can substantially impact the performance of chromatographic methods.
Properties of Ideal Carrier Gases
In the realm of gas chromatography, the properties of the carrier gas are fundamental to the success of the analytical method. Each characteristic plays a critical role in determining how well the system performs. Ideal carrier gases need to meet certain requirements to ensure efficient separation and enhanced detection.
Inertness
Inertness is perhaps the most crucial property of an ideal carrier gas. The gas should not react chemically with the sample or with the stationary phase in the chromatographic column. If the carrier gas were to interact with the analytes, it could lead to degradation or alteration of the samples, which would skew results and produce inaccurate data. Helium and nitrogen, for example, are commonly used due to their low reactivity. This property allows for the integrity of samples to be maintained throughout the separation process, thus ensuring reliable analyses.
Purity
Purity is another vital property when selecting a carrier gas. Any contaminants present in the gas can interfere with the separation of analytes. It can also lead to baseline noise, reducing sensitivity and making it more difficult to detect low-concentration compounds. In practice, the purity level of carrier gases must be very high; typically, 99.999% is desired. Special gas handling and purification systems may be employed to achieve and maintain this level of purity. Using pure carrier gases ultimately enhances the quality of results obtained.
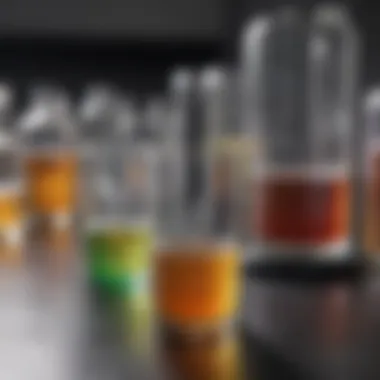
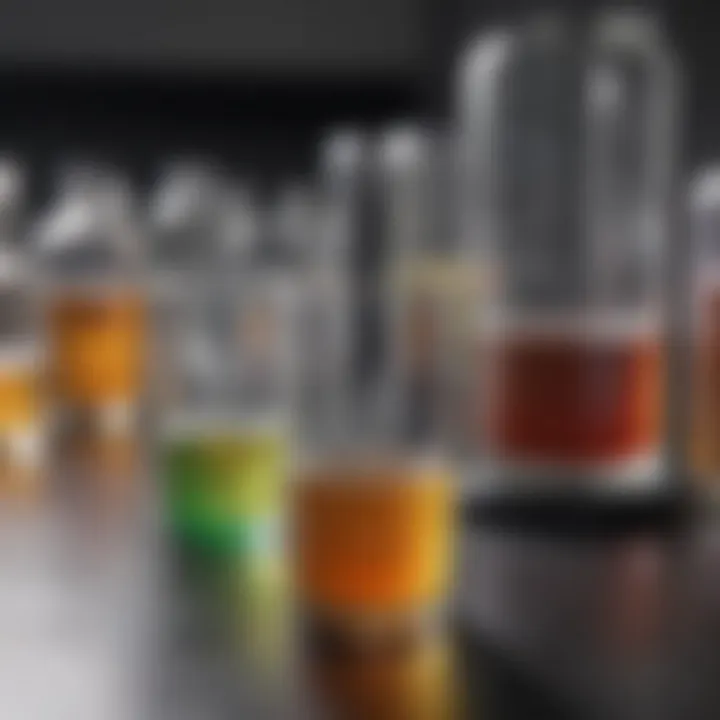
Viscosity and Temperature Stability
The viscosity of the carrier gas can affect flow rates within the chromatographic system, influencing the efficiency of the separation. A gas with lower viscosity allows for quicker flow rates, whereas higher viscosity can lead to slower flow rates, potentially causing increased retention times for analytes. Additionally, temperature stability is essential because changes in temperature can cause variations in gas behavior, leading to inconsistent results. Ideal carrier gases should maintain their properties under varying temperature conditions to ensure reliable data throughout the analysis.
"Understanding the properties of ideal carrier gases is imperative for anyone involved in gas chromatography. The choice of gas can significantly affect both the outcome and the efficiency of the analysis."
In summary, the inertness, purity, and viscosity along with temperature stability of carrier gases are key factors to optimizing performance in gas chromatography. The importance of these properties cannot be overstated, as they ensure the accuracy and reliability of results in analytical chemistry.
Selecting the Appropriate Carrier Gas
Choosing the right carrier gas is essential in gas chromatography. It influences the efficiency of separation, peak resolution, and sensitivity of the detected analytes. Each application has unique needs, making this selection a critical step. Finding a balance among factors such as cost, performance, and safety is vital for optimal chromatographic outcomes.
Analyte Characteristics
Analyte properties significantly dictate carrier gas selection. The polar or non-polar nature of the analyte impacts how well it interacts with the column and the carrier gas. For example, hydrophobic compounds may benefit from a non-polar carrier gas like helium. Conversely, if the analyte is polar, a more polar carrier may be necessary for improved resolution.
Factors to consider:
- Molecular Weight: Heavier analytes may require different conditions than gas-phase analytes.
- Chemical Behavior: Reactivity of the analyte should align with the chosen carrier gas to prevent unwanted reactions.
- Volatility: Highly volatile substances may prefer lighter gases for faster transfer and lower retention times.
Detection Methodology
The type of detection system used can greatly affect carrier gas choice. Certain detectors, like flame ionization detectors, work best with specific carrier gases. For example, hydrogen is favored for detectors requiring high sensitivity, while nitrogen offers more stability and safety in other scenarios. Understanding the compatibility between the carrier gas and detection technology enhances overall performance.
Considerations may include:
- Sensitivity Requirements: Some detectors require gases that help optimize sensitivity based on the analytes.
- Temperature Limitations: Carrier gases should operate efficiently within the temperature ranges of the chosen detection method.
- Interference Potential: The ideal carrier gas should not contribute to noise or interference with analyte detection.
Column Type
The selection of the Chromatographic column type is intertwined with carrier gas choice. Columns differ in coating and length, which directly influences how analytes are separated. A narrow bore column might require lighter gases for effective transport, while wider columns may manage heavier gases better without losing efficiency.
Important aspects include:
- Column Dimensions: Wide versus narrow columns can perform differently with various gases.
- Phase Coating: Compatibility of the gas with the stationary phase is crucial to avoid degradation of column performance.
- Temperature Profile: Increased temperatures can affect gas viscosity and, therefore, how effectively they carry analytes through the column.
"The choice of carrier gas can define the success of an analytical procedure.”
It's imperative to weigh these considerations to optimize laboratory practices.
Impact of Carrier Gas on Chromatographic Performance
The choice of carrier gas is integral to the efficacy of gas chromatography. A proper understanding of this aspect can significantly enhance analytical performance. Essentially, the impact of the carrier gas can influence the quality of the results obtained. There are several specific elements to consider when evaluating how the carrier gas affects chromatographic performance.
Resolution
Resolution is a measure of how well a gas chromatographic system can separate individual components within a mixture. The type of carrier gas affects both the width of the peaks on the chromatogram and the time taken for each analyte to pass through the column. Generally, helium provides a high resolution because it flows rapidly. This rapid flow reduces peak broadening, leading to sharper peaks that are easier to differentiate. In contrast, nitrogen is less efficient in providing the same level of separation. When using nitrogen, careful optimization of flow rates is necessary to achieve comparable resolution, which can sometimes be challenging.
Sensitivity
Sensitivity in gas chromatography refers to the ability to detect low concentrations of analytes. The carrier gas plays a significant role in determining the sensitivity of the method. Hydrogen, for instance, is known to enhance sensitivity more than helium or nitrogen. This is because hydrogen has a higher diffusion rate, which accelerates the transfer of analytes toward the detector. Opting for hydrogen as the carrier gas can lower the limit of detection, making it a preferred choice for applications that require the detection of trace components.
Analyte Retention Time
Retention time is the period an analyte spends in the chromatographic column before reaching the detector. This duration can vary significantly based on the carrier gas used. Generally, higher molecular weight carrier gases create longer retention times, thus affecting the efficiency and duration of the analysis. In a practical setting, choosing a lighter carrier gas like helium often leads to shorter retention times, facilitating faster analyses. Conversely, if longer analyses are needed to ensure complete resolution, researchers might select a heavier gas.
The choice of carrier gas is not merely a technical specification; it fundamentally shapes the analytical outcome and merits considerate attention during method development.
Practical Considerations in Using Carrier Gases
In the realm of gas chromatography, the choice and handling of carrier gases is not merely technical but also a blend of science and best practices that impact the efficiency of analytical outcomes. Carrier gases are foundational to the separation process, affecting resolution, sensitivity, and retention times of analytes. Thus, understanding practical considerations in using these gases ensures reliable and reproducible results in analytical chemistry.
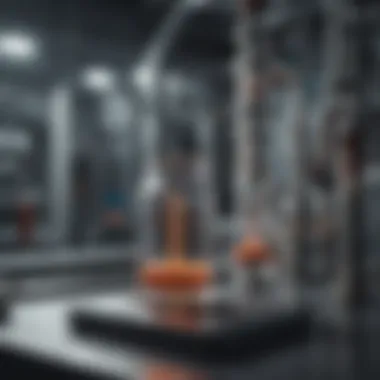
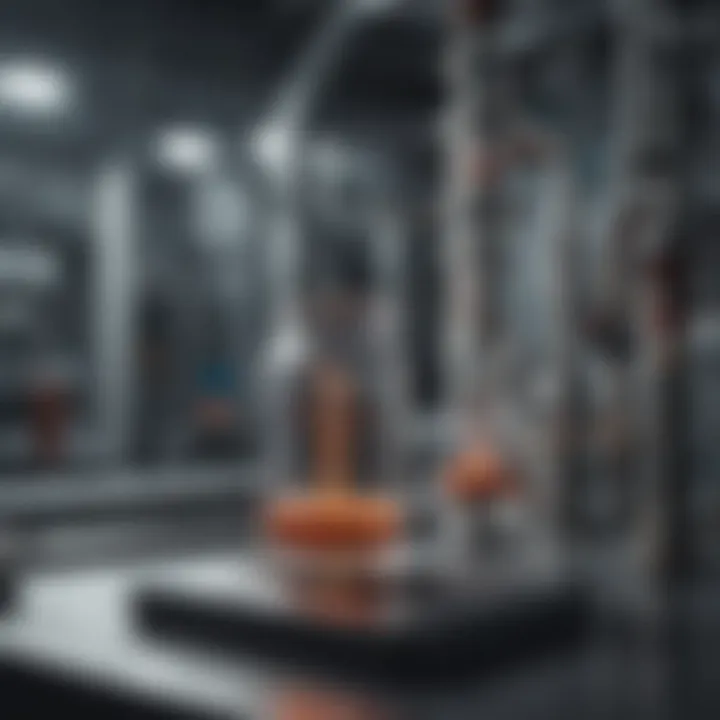
Gas Purity Standards
Gas purity is paramount when selecting carrier gases for chromatography. Any impurity can significantly alter the sensitivity and resolution of the chromatographic method, introducing background noise that can mask analyte signals. To maintain accuracy, laboratories should adhere to stringent gas purity standards.
- Common purity levels include:
- 99.999% for high-performance applications
- 99.99% for routine analyses
By employing gases of high purity, the risks of contamination are minimized. Regular testing and validation against purity standards should be implemented. Employing certified gas suppliers is a recommended practice to ensure that the gases used fit the specified purity requirements.
Regulatory Compliance
Adhering to regulatory compliance is crucial in laboratory settings, particularly when dealing with hazardous or flammable gases. Various guidelines govern the use of carrier gases in analytical chemistry. Familiarity with regulations from bodies such as the Occupational Safety and Health Administration (OSHA) or Environmental Protection Agency (EPA) helps institutions avoid legal ramifications and ensure safe operational procedures.
Understanding and implementing proper records for gas usage can aid in compliance. This includes detailing the types of gases used, their quantities, and any incidents that may occur.
"Proper adherence to regulatory compliance not only protects the laboratory personnel but also enhances the credibility of the research outcomes."
Safety Protocols
Implementing stringent safety protocols is essential when working with carrier gases. Each gas type presents unique hazards which require specific measures to address. The following guidelines should be observed:
- Training: Ensure all personnel are trained on the properties of the gases they are handling and the associated risks.
- Storage: Gases should be stored in appropriate cylinders, clearly labeled, and kept in well-ventilated areas to prevent accumulation of potentially hazardous vapors.
- Use of personal protective equipment (PPE): Maintain a requirement for safety glasses, gloves, and other PPE necessary for handling and working with gases.
By prioritizing safety protocols, laboratories can not only safeguard their staff but also adhere to compliance requirements, thus ensuring operational efficiency and reliable results.
Future Trends in Carrier Gas Applications
The study of carrier gases in gas chromatography is evolving rapidly. As analytical techniques advance, this section will explore significant future trends shaping the industry. Understanding these trends is essential for researchers and professionals aiming to stay ahead in the ever-changing landscape of analytical chemistry.
Emerging Technologies
Recent developments in analytical chemistry are highlighting new technologies that involve carrier gases. One notable trend is the advancement of micro gas chromatography. This technology employs smaller columns and samples, which require highly efficient carrier gases. Helium and hydrogen are gaining attention due to their unique properties, such as higher diffusivity, enhancing separation efficiency.
Another emerging technology is the integration of machine learning algorithms into gas chromatography. These tools optimize the selection of carrier gases based on specific analyte characteristics. By analyzing vast datasets, machine learning can predict the ideal gas for various conditions, resulting in better reproducibility and accuracy in chromatography results.
The shift to more compact instruments also necessitates innovative approaches in carrier gas management. New methods generate on-site gases or recycle used gases to minimize waste and reduce costs. This pushes the boundaries of what is achievable in gas chromatography.
Sustainability Considerations
Sustainability is becoming increasingly relevant in scientific practices. With heightened awareness about environmental impacts, laboratories are looking for ways to reduce their carbon footprint. The choice of carrier gas plays a vital role in this transition. Natural gas, for example, is being explored as an alternative to traditional gases like helium.
Implementing more sustainable practices can have several benefits for laboratories:
- Cost Reduction: Using locally sourced natural gases can lower expenses associated with purchasing and transporting gases.
- Energy Efficiency: New systems to generate and manage gases on-site can significantly reduce energy consumption.
- Regulatory Compliance: As environmental laws become stricter, laboratories must adapt to meet new regulations.
Some researchers are investing in studies to assess the lifecycle analysis of different carrier gases. This information aids in understanding the most environmentally-friendly options and practices in gas chromatography.
Innovations in Gas Purification
For gas chromatography to achieve optimal performance, the purity of the carrier gas is paramount. Innovations in gas purification systems are emerging as they are essential to eliminate impurities that may affect analyte response.
Advanced filtration technologies, such as membrane separation or pressure swing adsorption, are gaining traction to enhance gas quality. These systems can remove moisture and other contaminants efficiently.
"The cleanliness of carrier gases can significantly influence the results obtained in chromatographic analyses."
Such innovations promise not only improved accuracy but also cost savings through reduced downtime and reanalysis. As the industry progresses, researchers and laboratories must keep pace with these developments in gas purification to maintain the reliability of their analytical procedures.
In summary, the future trends in carrier gas applications reflect a convergence of technological progress and environmental responsibilities. Keeping informed about these advances will empower professionals to utilize carrier gases more effectively while supporting sustainability initiatives within the field.
Culmination
One must consider several factors when selecting a carrier gas. These include the physical properties of the analyte, the type of detector used, and the specifics of the chromatographic column employed. For example, helium is favored for its efficiency, while hydrogen offers increased sensitivity at a lower cost. Understanding these nuances allows for better optimization of the chromatographic process.
Moreover, the impact of carrier gas characteristics—such as purity and inertness—is significant. The presence of contaminants can distort results, leading to erroneous conclusions in research and laboratory settings. Therefore, practitioners need stringent protocols to ensure gas purity, accompanied by compliance with regulatory standards.
As we look to the future of gas chromatography, it is clear that advancements in carrier gas technology, sustainability efforts, and innovations in purification processes will play pivotal roles. Keeping abreast of these trends will not only enhance analytical capabilities but also align with broader environmental responsibilities.
Ultimately, the chapters of this article emphasize the intricate relationship between gas chromatography and carrier gases. Each section builds a strong case for understanding their essential nature and applications. From beginners to seasoned researchers, all stand to gain from a thorough grasp of these principles, elevating their capabilities in analytical chemistry.