Exploring the Wave Effect Across Sciences
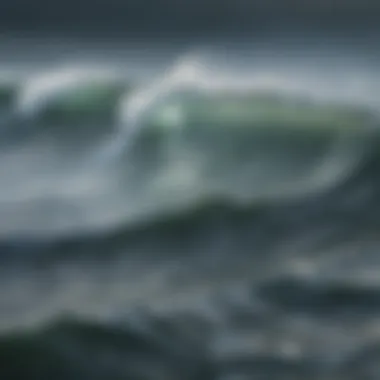
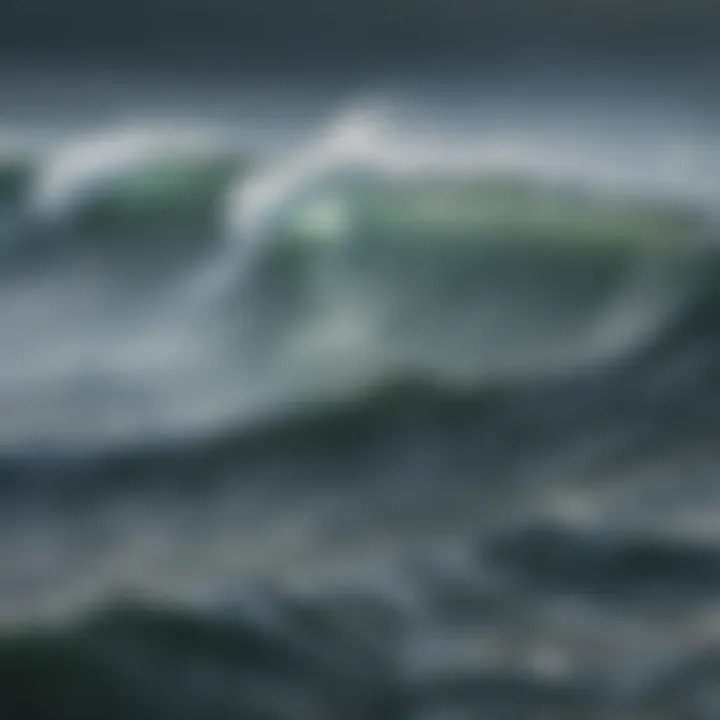
Intro
The concept of waves serves as a fundamental pillar across numerous scientific fields. Be it the rhythmic crashing of ocean waves, sound vibrations bouncing through the air, or the unseen patterns in molecular structures, waves behave in fascinating ways that warrant in-depth examination. This exploration delves into various disciplines—biological systems, physical phenomena, chemical interactions, and earth sciences—thus, accentuating the pervasive influence of wave dynamics.
Waves are more than mere ripples on the surface. They embody complex interactions that impact both the natural world and technological advancements. By understanding how wave patterns operate, we can glean insights that inform multiple areas of research.
Research Highlights
Overview of Key Findings
This piece will shed light on several critical areas:
- Biological Implications: The role of wave dynamics in biological processes and ecosystems, shedding light on phenomena like sound communication among animals or the ripples on a pond that affect its ecological balance.
- Physical Foundations: An outline of wave mechanics in physics, showing how principles apply in contexts like acoustics and optics, drawing connections to everyday technology.
- Chemical Applications: Investigating how wave behavior influences chemical reactions, particularly in photochemistry and reaction kinetics.
- Geophysical Insights: Understanding waves in relation to geological phenomena, including seismic waves and the study of natural disasters.
Significance of the Research
The significance of exploring wave effects lies in their ability to bridge gaps between disciplines. Waves not only represent physical phenomena but also unveil patterns and principles that can drive innovation. In a world dominated by rapid technological progress, grasping how waves influence both nature and technology will contribute to advancements in fields such as environmental science, renewable energy, and telecommunications.
To encapsulate, the wave effect serves as a connecting thread that ties together diverse scientific inquiries and applications. By engaging with this topic, researchers and professionals alike can cultivate a deeper appreciation for the natural world and its intricacies.
Original Research Articles
Summary of the Article
Each section will contain findings from various studies, transformed into a coherent narrative detailing the interconnectivity of wave effects across disciplines.
Author Contributions
Collaborators and experts from multiple fields will be cited, each contributing unique perspectives and findings, enriching the article and ensuring that it reflects a multi-faceted approach to the wave effect.
The Nature of Waves in Science
Waves are not merely a phenomenon confined to a specific branch of science; they permeate through multiple disciplines, acting as a connective thread in our understanding of the world. Grasping the essence of wave phenomena is crucial for students, researchers, and professionals alike, as it offers insights into a myriad of natural occurrences and human-made technologies. This section will navigate through the fundamental aspects of waves, deliberating their significance, characteristics, and roles across different fields of study.
Defining Wave Phenomena
When we talk about waves, we dive into a complex world where energy is transferred through oscillations, rather than through matter itself. Wave phenomena can be defined as disturbances that propagate through a medium, whether that be air, water, or even in a vacuum as with light waves. A clearer understanding of these phenomena is not just a scientific exercise, it serves practical purposes too. For instance, in areas such as telecommunications, waves form the backbone of signal transmission, making everyday communication possible.
Types of Waves: An Overview
Waves are broadly classified based on their characteristics, including the medium through which they propagate. This classification opens doors to a deeper comprehension of how different types affect various scientific disciplines. Here, we will explore three primary categories:
Mechanical Waves
Mechanical waves require a medium for their propagation. Think of sound waves traveling through air. The key characteristic of mechanical waves is their dependence on the elasticity and density of the medium. This feature makes them particularly relatable for this article since they underpin countless natural and technological phenomena. A unique aspect here is that mechanical waves can be seen in both longitudinal and transverse forms—like the way ripples spread across the surface of water (transverse) versus how sound moves through air (longitudinal). While they are beneficial for understanding everyday experiences, their properties can vary dramatically based on the medium, presenting both advantages and limitations in various applications.
Electromagnetic Waves
Electromagnetic waves, on the other hand, do not necessitate a medium for their travel. They root themselves in electric and magnetic fields, which is a defining trait that makes them integral to this article. These waves span a vast spectrum—from radio waves to gamma rays—and significantly contribute to modern technology, especially in communications and imaging. A remarkable feature of electromagnetic waves is their incredible velocity, allowing them to traverse vast distances at the speed of light. This characteristic makes them exceptionally beneficial for initiatives requiring high-speed data transmission. However, understanding their interaction with different materials can be key in certain contexts, revealing layers of complexity in their applications.
Surface Waves
Surface waves represent another critical type of wave, primarily found at the interface of two media, like air and water. They carry energy along the surface of the medium, and are crucial for absorbtion—this can be likened to the waves you observe when tossing a stone into a pond. A key characteristic of surface waves is their ability to cause significant disturbances without completely displacing the medium, which is quite fascinating. Their behavior and effects can be observed in various ecosystems, such as coastal areas where these waves significantly affect sediment transport and marine life habitats. In engaging with surface waves for this article, it’s important to consider their environmental implications and the delicate balance they maintain in ecosystems.
Mathematical Foundations of Waves
Understanding the mathematics behind waves helps bridge the gap between theory and practice. Here the focus shifts to equations and transformations that explain the waveforms mathematically. Wave equations dictate how wave patterns behave and interact, while Fourier transformations offer tools for analyzing complex waveforms by breaking them down into simpler components.
Wave Equations
Wave equations are foundational to wave theory, governing how waves propagate through different media. Their mathematical forms can vary, but they typically involve second-order differential equations. One standout feature of wave equations is their ability to describe not only the position of a wave over time but also its velocity and acceleration. This characteristic makes wave equations invaluable for scholars and engineers focusing on applications in acoustics, optics, and other fields. However, deriving these equations often requires a robust understanding of calculus, sometimes presenting a barrier for newcomers to the subject.
Fourier Transformations
Fourier transformations revolutionized our way of looking at wave phenomena by allowing us to decompose complex waveforms into their constituent frequencies. In simpler terms, it’s like breaking down a symphony into individual notes, thereby providing clarity and insight across numerous applications. This mathematical tool is particularly beneficial for signal analysis, as it facilitates a breakdown of waveforms into manageable parts. However, the complexity may pose a challenge for those not familiar with advanced mathematics.
Understanding the nature of waves—be it through their types or their mathematical foundations—forms the bedrock of this analysis, revealing how interconnected various scientific disciplines are when examining the wave effect. This melding of insights ultimately enriches research across biology, physics, chemistry, and beyond, enabling a refined approach to understanding nature's rhythm and its implications for technological advancement.
Wave Dynamics in Biological Systems
The examination of wave dynamics within biological systems is crucial for understanding a variety of processes that underpin life itself. Waves influence not only structures but also interactions in biological organisms, making them significant to both ecology and physiology. Waves of energy, signals, and even chemical reactions are all part and parcel of how living systems adapt and communicate. By delving into the various aspects of wave dynamics, we can glean insights that may guide advancements in science, medicine, and environmental sciences. Ultimately, exploring this area reveals opportunities for bridging gaps between disciplines, fostering innovation and research.
Biological Significance of Wave Patterns
Cellular Communication
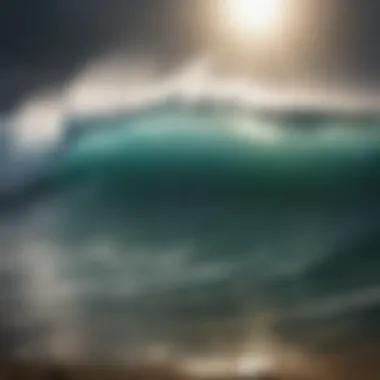
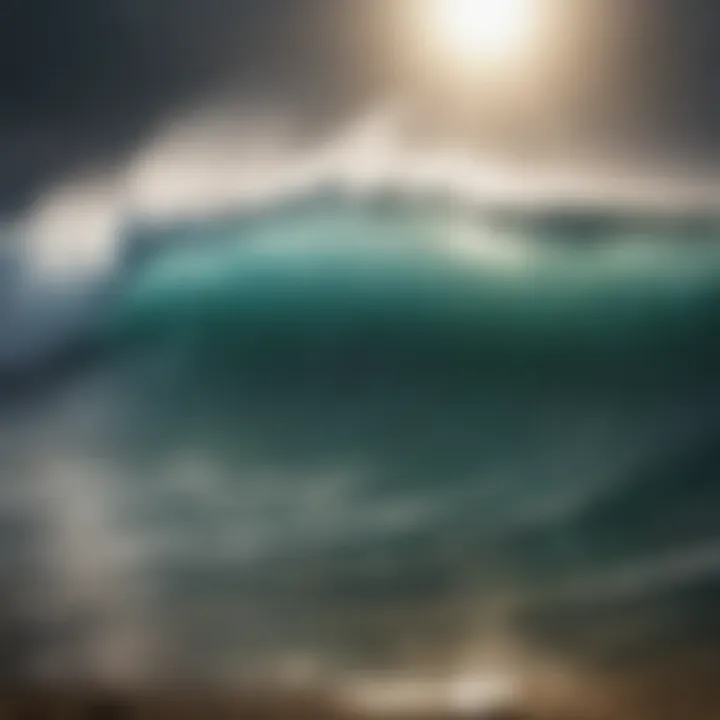
Cellular communication is fundamental for coordination among cells, essential for organismal development and function. At its core, cellular signaling often involves wave-like patterns of chemical or electrical signals that traverse cellular membranes to convey information. This dynamic is pivotal, as the waves facilitate critical functions such as growth, response to stimuli, and homeostasis.
The remarkable aspect of cellular communication is its efficiency; cells that can communicate rapidly via waves can optimize their functions. This is particularly beneficial in scenarios like nerve impulses where speed is of the essence. Despite its advantages, such a rapid process can sometimes lead to miscommunication or harmful signaling if pathways are disrupted, which could result in diseases. Thus, while cellular communication waves offer immense benefits, they also come with challenges that are essential for scientists to address.
Neural Oscillations
Neural oscillations refer to the rhythmic and repetitive patterns of neural activity in the brain, resembling wave patterns. They serve as the foundation for various cognitive processes, including attention, perception, and memory consolidation. The oscillations vary in frequency and amplitude, reflecting different states of consciousness or cognitive load.
One key characteristic of neural oscillations is their ability to integrate information across different brain regions, contributing to coordinated cognitive functions. This makes them a fascinating topic for researchers interested in understanding brain dynamics. However, the very nature of these oscillations can also pose risks; disruptions in their patterns have been linked to disorders like epilepsy. Thus, while neural oscillations provide critical insights into brain function, potential variability brings both light and shadow to their study.
Application of Waves in Ecology
Sound Waves in Animal Communication
Sound waves play a vital role in how animals interact with each other and their environment. Whether through mating calls, warning signals, or territorial claims, sound serves as a vital communication tool in the animal kingdom. These auditory waves are often tailored to specific environments, with animals evolving unique sounds that travel efficiently in their habitats.
The beauty of sound waves in communication lies in their versatility; they can convey complex information over long distances, making them a popular subject for study in biology. For instance, some species can modulate their calls based on background noise, showcasing adaptability. However, the reliance on sound for communication can also backfire, as changes in environments can disrupt these patterns, affecting behaviors and ultimately survival. This dimension in animal communication creates a challenging dynamic worth exploring.
Water Waves Affecting Coastal Ecosystems
Water waves profoundly impact coastal ecosystems, influencing nutrient distribution, sediment transport, and habitats for marine life. They play a role in shaping coastlines and determining the types of ecosystems that can develop in these areas. Beyond physical alterations, the dynamics of water waves can affect biological interactions, such as feeding behaviors in fish and the breeding cycles of marine organisms.
One noteworthy feature of water waves is their capacity to transfer energy across vast distances, making them a powerful force in oceanic environments. This wave action can also result in beneficial nutrient upwellings that support diverse marine food webs. However, the impact of waves is a double-edged sword; severe wave action from storms or human activities can lead to habitat destruction or biodiversity loss. Understanding these dynamics is essential for conservation efforts and addresses the pressing issues of climate change.
Physics of Waves: The Theoretical Framework
The study of waves is fundamental to our understanding of various natural phenomena, making the theoretical framework of wave physics not just essential, but also intriguing. Waves manifest in countless forms across different disciplines, and their principles govern many processes we encounter in everyday life, from the sound of music to the radiance of outer space. Understanding waves introduces a coherent way to bridge concepts across biology, chemistry, and geology, offering insights into the interconnectivity of these fields.
Wave Properties and Behavior
Wavelength and Frequency
Wavelength refers to the distance between successive crests of a wave, while frequency indicates how many crests pass a point in a given period. Their relationship is straightforward: more significant wavelengths typically mean lower frequencies and vice versa. This inverse relationship is pivotal when studying waves as different media transfer energy differently.
It's important to note that the wavelength is a key characteristic because it directly influences the wave's utility in applications such as telescopes or sonar systems. For instance, longer waves penetrate deeper water, making them ideal for sonar use in oceanographic studies. However, longer wavelengths can also mean reduced resolution in visual technologies, presenting a unique feature that must be considered in design.
One significant advantage of grasping the concepts of wavelength and frequency lies in their applicability to numerous technologies, from telecommunications to medical imaging systems like ultrasound. Conversely, the disadvantage often lies in the complexity of measuring and adjusting these variables in practical situations, which can require elaborate setups or calculations.
Amplitude and Energy Relationships
Amplitude defines the height of the wave from its rest position and serves as a direct indicator of its energy. Waves with greater amplitude carry more energy and can produce more significant effects when interacting with their surroundings. The connection between amplitude and energy is crucial for understanding wave behavior in various contexts.
The key characteristic of amplitude is that it directly correlates with the wave's impact on matter; for example, louder sound equals a higher amplitude. This principle makes it beneficial in industries where controlling sound levels is critical, such as in concert halls or recording studios. However, higher amplitude waves can lead to destructive results, like when sound barriers are broken and create noise pollution, highlighting a unique feature of amplitude's dual nature.
While leveraging amplitude can enhance applications in acoustics or signal transmission, a disadvantage emerges when waves of excessively high amplitude result in unintended consequences, such as structural damage or healthcare issues related to noise exposure.
Wave Interference and Diffraction
Constructive and Destructive Interference
Interference is a fascinating aspect of waves where two or more waves overlap, resulting in a new wave pattern. Constructive interference occurs when waves combine to create a wave with greater amplitude, enhancing the signal. This effect is significant in applications like amplification in audio systems, where maximizing sound output is a goal. Conversely, destructive interference happens when waves cancel each other, leading to a decrease in amplitude, which can be problematic in scenarios demanding clear transmissions.
The balance between these two phenomena is crucial for devising strategies in signal processing, ensuring a stable output in communication technologies. However, the chance of unintentional interplay between sound waves in crowded spaces demonstrates the real-world implications of interference that may not always be beneficial.
Applications in Optics
Wave theory applies significantly in optics, wherein light behaves as a wave. Various optical phenomena, such as diffraction and interference patterns, shed light on how light interacts with different materials. This interaction is crucial in designing lenses and optical devices, where precision is key.
One key characteristic in optics is color, which results from waves of different wavelengths interacting with our perception systems. Understanding this can lead to advancements in display technology or even paint formulations. The unique feature of optics is its ability to manipulate light through various mediums, enhancing numerous applications in photography and microscopy.
However, working with optical applications can be advantageous because of the extensive tools and methods available for wave manipulation. Still, challenges in calibration and alignment can lead to inaccuracies if not meticulously accounted for during experimentation or product design.
"Understanding the theoretical aspects of waves enriches our grasp of how interconnected various scientific realms are."
This section highlights the essential principles governing wave behavior, showcasing how they serve as the building blocks for scientific exploration and technological advancement in diverse fields.
Chemical Waves: Reactions and Effects
Chemical waves play a significant role in various chemical processes, reflecting patterns that not just advance our basic understanding but also shape a range of applications in material science and beyond. The phenomena of chemical waves come to light through the study of reaction dynamics, where reactions can exhibit spatial and temporal patterns. This section aims to unpack these complexities, considering both the theoretical and practical implications of such systems.
Wave Patterns in Chemical Reactions
Reaction-Diffusion Systems
At the heart of reaction-diffusion systems lies an intricate balance between chemical reactions and the diffusion of reactants. These systems are crucial as they provide a framework for understanding how localized reactions can lead to large-scale pattern formation. A key characteristic of reaction-diffusion systems is oscillation, which allows for dynamic behavior over time. This makes them particularly popular for modeling biological processes such as pigment patterns in animal skins or even the formation of spatial structures in ecological systems.
The unique feature here is that reaction-diffusion systems don’t rely solely on the concentration of substances. They involve feedback loops wherein the products influence the reactants, thus encouraging complex pattern generation. Advantages of this approach include the ability to predict emergent behavior in complex systems. However, one cannot overlook the disadvantages, as these systems can sometimes lead to chaotic patterns that are difficult to model accurately.
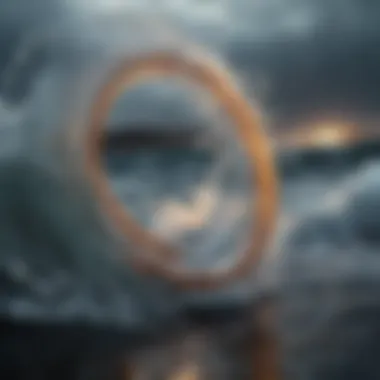
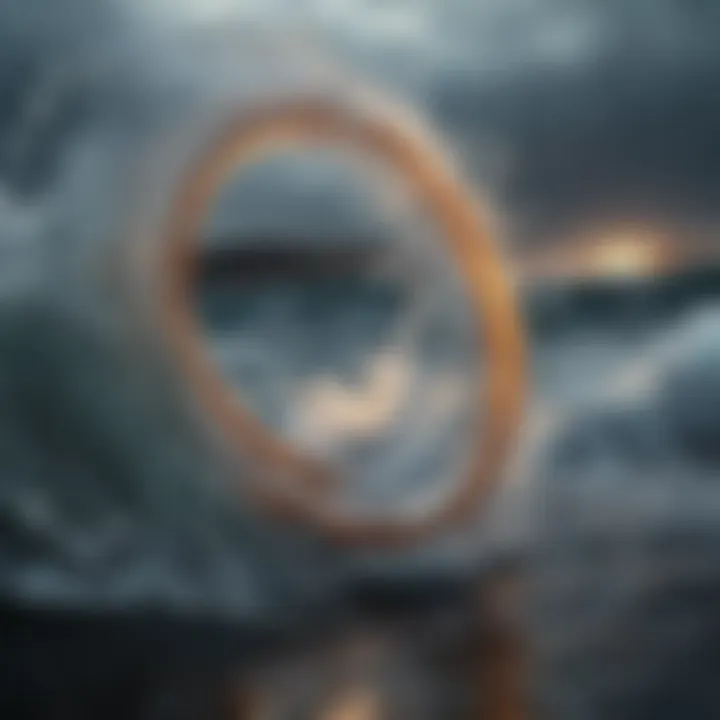
Oscillating Chemical Reactions
Oscillating chemical reactions are another fascinating aspect within the domain of chemical wave phenomena. These reactions are characterized by periodic changes in concentration of reactants and products, resulting in distinctive rhythmic patterns. A notable example is the Belousov-Zhabotinsky reaction, which showcases vibrant color changes over time, echoing a natural wave-like rhythm.
The periodic nature of these reactions makes them a compelling subject of study as they allow insights into non-equilibrium thermodynamics. Their advantage lies in their ability to provide real-time observation of chemical processes, which can be a useful tool for both research and education. Nevertheless, a unique challenge with oscillating reactions is that they may require well-controlled conditions to maintain stability, which can limit their practical applications.
Implications for Material Science
Through the lens of material science, the exploration of chemical waves broadens the horizon on how materials can be developed and utilized. Investigating these chemical phenomena allows scientists to harness wave patterns to improve the properties and performance of various materials.
Wave Phenomena in Nanotechnology
In the realm of nanotechnology, wave phenomena can be pivotal, especially in controlling reactions at the nanoscale. The key characteristic of wave phenomena here lies in their ability to influence the ordering and self-assembly processes of nanomaterials. This can lead to the development of advanced applications in electronics, sensors, and drug delivery systems.
The distinct feature of wave phenomena in nanotechnology is their capacity for precise control, leading to the fabrication of materials with tailored properties. The advantages are substantial; it allows for efficiency and innovation in material design. However, challenges arise in replicating these phenomena consistently across larger scales, which limits immediate applications in some cases.
Role of Waves in Polymer Reactions
Waves also play an intriguing role in polymer reactions. The dynamics involved can impact chain growth rates and branching, markedly affecting material properties like elasticity and strength. A key characteristic of these waves is that they can initiate self-organization in polymer systems, leading to enhanced material performance.
The unique aspect of waves in polymer reaction is their potential to optimize formulations for specific industrial applications, which can be highly beneficial. However, one must also consider the disadvantages, such as the complexity of achieving uniform reaction conditions across batches, which can impede scalability.
"Understanding chemical waves opens up a dialogue between chemistry and material science, illuminating pathways for innovation."
Overall, the study of chemical waves—their patterns, implications, and interactions—provides a deeper understanding of the processes that govern material behavior. As intricate as they may be, these waves embody a potent interplay of reaction dynamics and structural integrity, which is vital to harnessing their full potential in diverse fields.
Geological Waves and Their Impact
Geological waves play a pivotal role in understanding our planet’s dynamics, particularly regarding the shifts in the Earth's crust and the enormous energy release during geological events. Grasping the nuances of geological waves is essential not just for geologists but for various sectors, including civil engineering, urban planning, and disaster management. This section will explore the intricacies of both seismic and ocean waves, their impacts, and how they interlace with our daily lives.
Seismic Waves and Earthquakes
Types of Seismic Waves
Seismic waves are energy waves that propagate through the Earth, generated primarily by tectonic movements during earthquakes. They come in various forms, primarily categorized into two types: Body waves and Surface waves.
- Body Waves: These travel through the Earth’s interior and are subdivided into Primary (P) waves and Secondary (S) waves. P waves travel faster and can move through liquids, while S waves cannot. Their speed and ability to reveal subsurface structures make them vital for geological surveys and earthquake research, contributing to our understanding of the Earth’s makeup.
- Surface Waves: These waves travel along the ground and are generally responsible for the majority of the destruction during an earthquake. Their slower speed contrasts with body waves, yet they carry the most energy. The prominent oscillating motion characteristic of surface waves can cause considerable ground shaking and thus severe infrastructural damage.
Seismic waves are beneficial in deciphering Earth's layers and understanding the mechanics behind earthquakes. The unique features of these waves, like their varied speeds and types, allow geologists to estimate the epicenter and magnitude of seismic events, proving pivotal in impact assessments and mitigation strategies.
"Understanding the types of seismic waves allows us to prepare and react more effectively to earthquakes, potentially saving lives and property."
Impact Assessment and Mitigation
Impact assessment related to seismic activity is crucial for minimizing risks associated with earthquakes. This process involves evaluating the potential effects of seismic events on structures, the environment, and communities. The aim here is to devise strategies to reduce these impacts.
One key characteristic of impact assessment is the integration of advanced technologies like Geographic Information Systems (GIS) and seismic hazard mapping. This approach provides detailed analyses of potential earthquake impacts, considering geographical and structural vulnerabilities.
Moreover, mitigation strategies encompass retrofitting buildings, improving urban design, and establishing early warning systems. These unique features make the assessment and mitigation process a cornerstone of urban resilience against seismic hazards. The advantages are manifold: community safety, economic savings, and enhanced emergency preparedness. However, challenges such as funding, technological access, and community awareness remain significant hurdles.
Ocean Waves and Coastal Dynamics
Ocean waves, much like their geological counterparts, significantly influence coastal environments and ecosystems. Thes waves are vital for understanding coastal dynamics, erosion patterns, and the health of marine habitats.
Tsunamis and Their Effects
Tsunamis are among the most destructive ocean waves; they result from undersea earthquakes, volcanic eruptions, or landslides. The specific aspect that defines tsunamis is their ability to travel long distances at incredible speeds, often unnoticed until they reach shallow waters, where they rise dramatically, flooding coastal areas.
The primary characteristic of tsunamis is their immense energy and volume, capable of causing catastrophic damage to infrastructure and loss of life. Their effects extend beyond the immediate impact, leading to long-term ecological changes in coastal ecosystems and providing a barrier against human encroachment in sensitive areas.
While the understanding of tsunamis is beneficial for disaster response and recovery planning, the unique features that enable their study—such as tsunami warning systems—allow for timely evacuations. The disadvantages hinge on their unpredictability and the need for comprehensive monitoring systems, which can be costly to maintain.
Waves in Marine Navigation
Waves play a critical role in marine navigation, influencing the routes taken by ships and the safety of maritime operations. This aspect is crucial, as over 80% of global trade by volume transits by sea. Understanding wave patterns and predicitions allows navigators to ensure safety and efficiency.
A key characteristic of this topic is the use of wave modeling systems that predict wave behavior based on various factors like wind speed and ocean currents. These models assist in avoiding hazardous conditions, allowing for smoother and safer voyages.
The unique feature here is the interdependence of marine navigation and environmental conditions, which influence not only navigation routes but also maritime safety protocols. However, challenges often arise from the volatility of ocean conditions and the need for real-time data, emphasizing the importance of ongoing research and technology in improving navigation.
This exploration of geological waves illustrates their profound influence on both natural systems and human infrastructure, highlighting the necessity for ongoing study across multiple disciplines to foster resilience and adaptability in the face of geological phenomena.
Technological Applications of Wave Theory
The breadth of wave theory's influence extends far beyond mere academic pursuits, tangling itself with our day-to-day technological environments. By dissecting wave phenomena, we not only gain insights into fundamental science but also hone practical applications that shape communication, energy systems, and even environmental considerations. Wave theory is undeniably the backbone of many modern technologies, enabling advancements that connect people and harness natural power in innovative ways.
Wave Technology in Communication Systems
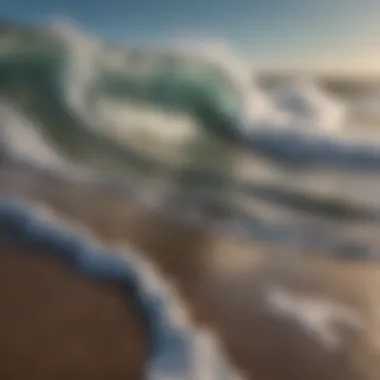
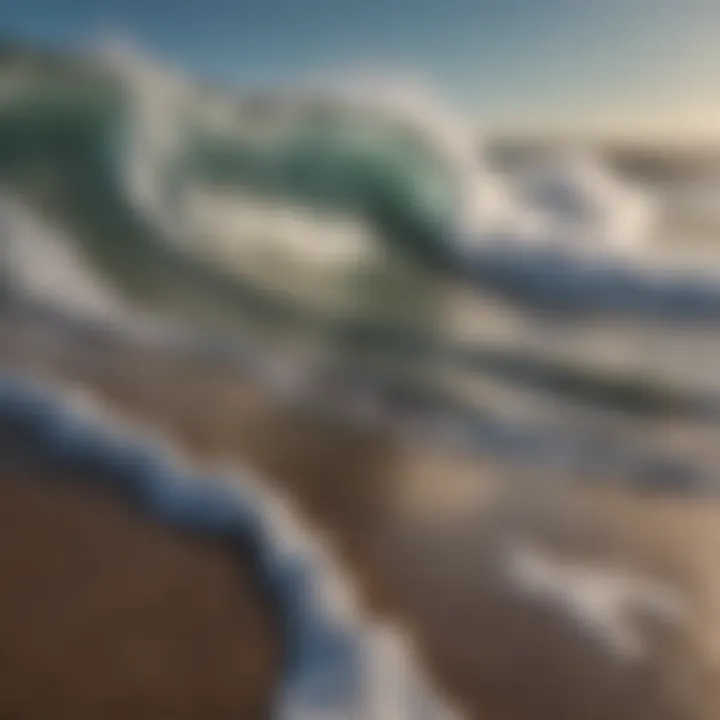
Radio Waves and Telecommunications
Radio waves stand as a pillar of telecommunication, facilitating the rapid exchange of information globally. Rooted in electromagnetic wave theory, these waves are characterized by their long wavelength and ability to travel vast distances with minimal loss. This quality makes radio waves particularly potent for applications ranging from AM/FM broadcasts to mobile connectivity. Interestingly, the unique feature of radio waves is their capacity to penetrate various materials, making them an optimal choice for transmitting signals through buildings and atmospheric conditions.
Advantages:
- Wide coverage area, enabling communication over large distances.
- Ability to transmit audio without the need for wires, which is both convenient and cost-effective.
While radio waves offer significant benefits, they also come with drawbacks. For instance, overcrowding of frequencies can lead to interference, impacting communication clarity.
Optical Fibers and Light Waves
Shifting gears from radio waves, we enter the realm of optical fibers and light waves—another major player in the communication sector. These fibrous pathways leverage the properties of light waves to transmit data at astonishing speeds, far surpassing traditional metal wire systems. The key characteristic of optical fibers is their capacity to reflect light internally, allowing for minimal loss of data across long distances. This unique feature not only enhances transmission speeds but also significantly lowers the risk of electromagnetic interference.
Advantages:
- High bandwidth, capable of handling massive amounts of data.
- Greater energy efficiency than traditional cabling methods, leading to reduced operational costs.
However, the installation and maintenance of optical fiber networks can be more complex and costly, a consideration that can’t be ignored, especially when planning extensive networks.
Innovations in Wave Energy
Wave Energy Conversion Techniques
Tapping into the rhythmic pulse of ocean waves, wave energy conversion techniques represent a promising avenue for sustainable energy generation. These technologies convert the kinetic energy of ocean waves into electricity. The undeniable appeal of wave energy lies in its vast potential; it's a clean, renewable source that can contribute significantly to global energy needs. A common approach is point absorber buoys, which float on the water's surface and move in response to wave movements, generating power as they bob.
Advantages:
- Sustainability: Wave energy is inexhaustible as long as the seas exist.
- Consistency: Ocean waves provide a predictable energy source, complementing solar and wind power variability.
Nonetheless, challenges exist such as environmental impacts on marine ecosystems, and high costs of initial installations.
Environmental Impact of Wave Energy
As we advocate for renewable resources, assessing the environmental impact of wave energy becomes crucial. While harnessing wave energy presents a low-carbon advantage, it's essential to evaluate how installations might interact with marine life and coastal environments. The key characteristic of focusing on environmental implications is that these considerations can either bolster or hinder future projects. Sustainable practices ensure that the benefits of this energy don't come at the expense of ocean biodiversity.
Advantages:
- Potential to significantly reduce fossil fuel dependency.
- Can foster new habitats if designed thoughtfully.
However, ecological concerns persist. Disruption to natural habitats and changes in local water currents are aspects that require diligent study. Moreover, public perception influences the development of wave energy technologies, as communities look to balance innovation with conservation.
"The intersection of wave theory and technology is not merely a field of study; it’s a gateway to sustainable living and connectedness that modern society deeply needs."
Interdisciplinary Perspectives on Wave Phenomena
The phenomenon of waves serves as a common thread that weaves through various scientific fields. Taking an interdisciplinary perspective not only enriches our understanding of wave dynamics but also enhances collaboration among disciplines. This is crucial, especially as scientific challenges grow more complex in today's world. Recognizing that waves impact biological systems, technological innovations, and geological events underscores their relevance across domains.
Collaborative Research Across Disciplines
Synergy Between Physics and Biology
One fascinating aspect of the synergy between physics and biology is how wave mechanics can explain processes within living organisms. For instance, sound waves play a crucial role in cellular signaling and neural communication. This intersection is particularly useful in studying how organisms adapt to their environment, as sound waves can influence behavior and perception.
A key characteristic here is the application of principles from physics, such as resonance and frequency analysis, to decode biological communication. This cross-pollination paves the way for innovative solutions in fields such as bioacoustics and medical diagnostics.
Unique features include the ability to draw on mathematical models from physics to describe biological phenomena in new ways. This approach can yield significant advantages, like enhanced understanding of diseases linked to auditory functions or improved techniques in imaging. However, challenges arise due to the inherent complexity of biological systems, making it tough to develop universally applicable models.
Chemical and Geological Interactions
Moving onto chemical and geological interactions, we find another rich area for exploration. The interplay of waves in both chemical reactions and geological events like earthquakes can yield insights into material behavior and safety protocols. Understanding this interaction can also help in resource management and disaster preparedness.
One striking feature here is the way chemical waves, like the Belousov-Zhabotinsky reaction, demonstrate how symmetry and chaos can occur within chemical processes. Examining these interactions offers a compelling look at natural systems. The advantage of studying wave-induced phenomena in this context is the potential for advancing material science, especially in creating new composites. Still, the downside is the unpredictability that comes with complex systems, posing risks in real-world applications.
Future Directions in Wave Research
With all this in mind, we look toward the future paths we can tread in wave research.
Emerging Technologies
Emerging technologies in wave research continue to make headlines. Tools like advanced imaging techniques and computational models are changing how we observe and predict wave behaviors across all fields. These technologies enhance our capacity to visualize phenomena that were once unseen. They also allow for real-time analysis, which can be a game changer in fields like environmental monitoring and healthcare.
The unique aspect of these technologies lies in their ability to handle vast data sets, which leads to better modeling of complex systems. The challenge, however, is ensuring the accuracy and integrity of the data being processed, especially as the technology becomes more accessible.
Challenges and Opportunities
Finally, challenges and opportunities mingle as we examine the trajectory of wave effects in research. The constant evolution of scientific inquiry means that the methodologies and frameworks we employ are under continuous scrutiny. While this offers the opportunity to refine approaches and enhance knowledge, it also requires adapting to new paradigms.
A key characteristic here is the focus on sustainability; researchers are grappling with the environmental implications of their work. Unique features include interdisciplinary collaborations, which open doors to fresh insights and potential breakthroughs. However, balancing the intricate demands of varied scientific fields remains a significant challenge.
"The beauty of understanding waves lies in their omnipresence, teaching us that every discipline can contribute to a more cohesive picture of reality."
In summary, interdisciplinary perspectives on wave phenomena highlight not just scientific curiosity but a necessity for collaborative efforts. By effectively merging insights from physics, biology, chemistry, and geology, we bolster our capacity to address pressing global issues. The road ahead will undoubtedly be paved with opportunities, but it requires navigational skill, open-mindedness, and a willingness to explore uncharted territories.