Comprehensive Insights into Genetics: A Detailed Exploration
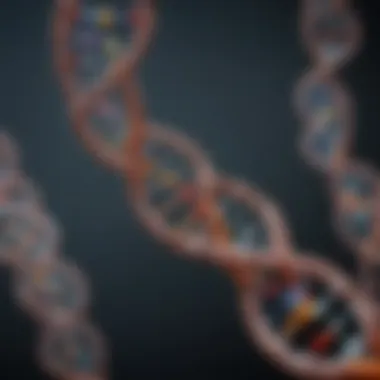
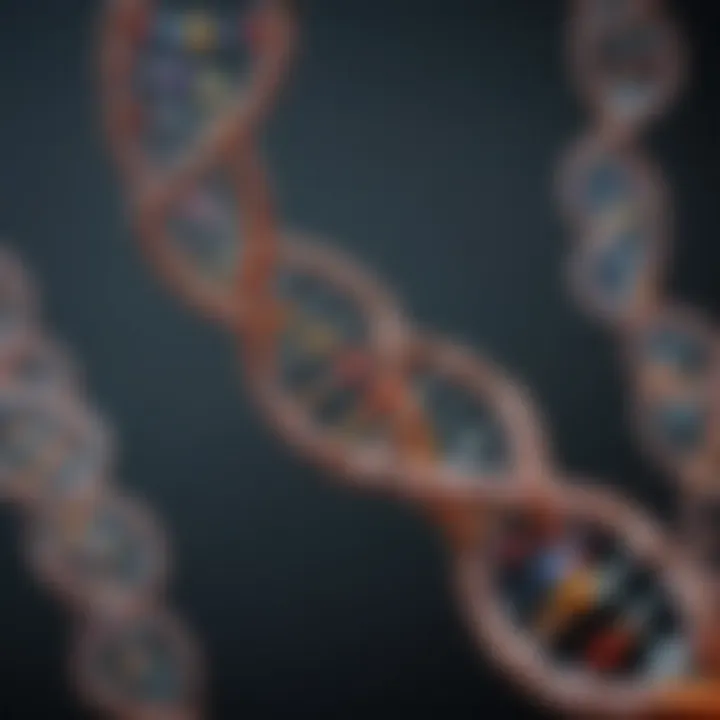
Intro
Genetics is a field that intertwines science and ethical considerations, shaping our understanding of life at the most fundamental level. This article navigates the complex landscape of genetics, highlighting critical principles and uncovering recent advancements. It seeks to present an integrated view that strikes a balance between technical detail and accessibility, making it valuable for a variety of audiences, from students just beginning their journey to seasoned researchers.
As we proceed, we will examine the historical evolution of genetic science, focusing first on foundational concepts before delving into modern breakthroughs and the ethical dilemmas they present. The discussion will highlight how genetics influences both individual lives and broader societal structures. Ultimately, our aim is to foster informed discussions that recognize the relevance of genetics in contemporary issues.
Research Highlights
Overview of Key Findings
Recent studies in genetics have provided remarkable insights into the mechanisms of hereditary traits and the role of specific genes in health and disease. Advances in molecular biology techniques, such as CRISPR-Cas9 gene editing, have unlocked new potential to modify genetic sequences in organisms. These findings open avenues for therapeutic interventions, particularly in genetic disorders like cystic fibrosis and muscular dystrophy.
Furthermore, research into epigenetics has shown how environmental factors can influence gene expression. This adds a layer of complexity to our understanding of genetics, emphasizing that heredity does not operate in isolation.
Significance of the Research
The implications of these findings are profound. First, they underline the potential of tailored medical treatments based on individual genetic profiles, known as personalized medicine. Second, societal discussions around genetic modifications and bioethics are becoming increasingly pertinent. As we understand more about our genetic makeup, the conversations about what is ethically acceptable will intensify.
Genetic research not only impacts medicine but also touches on agriculture and biodiversity, influencing how we approach food security and species conservation.
"Genetics stands at the intersection of technological advancement and ethical responsibility, prompting critical dialogues in modern society."
As we dive deeper into original research articles and their contributions, this article will provide a thorough synthesis of the information, guiding readers through the multifaceted world of genetics.
Prolusion to Genetics
Genetics serves as a critical cornerstone in the realm of biological sciences. Understanding genetics is essential not only for the study of organisms but also for the advancements in medicine, agriculture, and molecular biology. The discipline provides the tools to explore the hereditary traits, genetic variability, and the mechanisms that govern life itself. In this section, we will elucidate why genetics holds immense importance in both academic research and practical applications.
Defining Genetics
Genetics is the study of genes, genetic variation, and heredity in living organisms. At its core, this field seeks to understand how traits and characteristics are passed down from one generation to another. The term itself derives from the Greek word "genesis," meaning origin or creation. In contemporary science, genetics encompasses several subfields, including molecular genetics, population genetics, and quantitative genetics.
To define this complex discipline, one must consider key components:
- Genes: Fundamental units of heredity, responsible for encoding proteins and determining traits.
- DNA: The molecular blueprint that carries genetic information.
- Chromosomes: Structures within cells that house genes, organized into certain patterns.
An accurate grasp of these elements allows deeper engagement with how living systems develop and respond to their environment.
Historical Context
Understanding the historical context of genetics enhances its significance. The origins of genetics trace back to the 19th century, notably with the pioneering work of Gregor Mendel. Through meticulous experimentation with pea plants, Mendel established foundational principles of inheritance. His findings, such as the concepts of dominant and recessive traits, laid the groundwork for modern genetics but remained unrecognized for decades.
The rediscovery of Mendel's laws in the early 20th century coincided with advances in cytology and biochemistry, catalyzing the birth of genetics as a formal science. Key moments in this journey include:
- The mapping of chromosomes and the identification of genetic linkage.
- The elucidation of DNA structure by James Watson and Francis Crick in 1953, opening doors to molecular genetics.
Through these historical milestones, genetics evolved from a rudimentary understanding of heredity to a sophisticated field probing the intricacies of life. Studying its evolution provides essential insights into contemporary genetic research and its ongoing potential.
"Genetics is not just about traits. It's about the rules of life, how information is stored, shared, and transformed."
As we advance through this article, we will explore the fundamental concepts that underpin genetics and its myriad applications across various fields.
Fundamental Concepts in Genetics
Understanding the fundamental concepts in genetics forms the backbone of this article. These concepts provide clarity on the mechanisms that dictate heredity, variation, and the very function of life at a molecular level. Recognizing these basics lays a solid foundation for exploring advanced genetic theories and applications later in the article.
Genes and Genomes
Genes are the basic units of heredity. They are segments of DNA that encode instructions for building proteins, which carry out essential functions in living organisms. Each gene is composed of sequences of nucleotides, and variations in these sequences can lead to differences in the traits of individuals.
A genome refers to the complete set of DNA in an individual organism, including all of its genes. Understanding genomes is key in genetic research, as it involves not only studying genes individually but also their relationships and interactions within the larger system. Genomics, the study of genomes, has advanced rapidly, allowing researchers to explore the entire genetic blueprint of various organisms. This field is invaluable for applications such as personalized medicine, where treatments are tailored based on an individual’s genetic makeup.
Chromosomes and their Structures
Chromosomes are structures that organize and package DNA in cells. In humans, each somatic cell typically contains 46 chromosomes, arranged in 23 pairs. These structures are essential for ensuring proper segregation of genetic material during cell division. The understanding of chromosomes has evolved significantly, especially in the context of diseases that arise from chromosomal abnormalities, such as Down syndrome, which is caused by an extra copy of chromosome 21.
Chromosome structures can influence gene expression. For instance, regions within chromosomes have specific functions, such as the centromere, which plays a crucial role in chromosome segregation, and telomeres, which protect the ends of chromosomes from deterioration. The study of chromosome structure is essential for fields like epigenetics, which examines modifications that affect gene activity without altering the DNA sequence itself.
DNA Replication
DNA replication is the process through which a cell copies its DNA, ensuring that each daughter cell receives an accurate copy of the genetic material. This process is complex and involves multiple enzymes, including DNA polymerases, which are responsible for synthesizing new DNA strands.
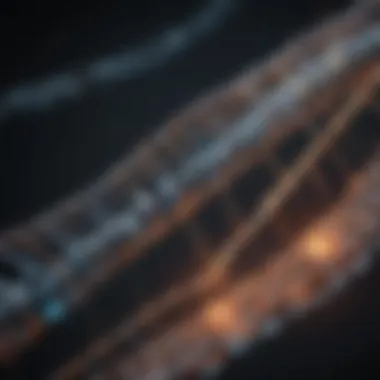
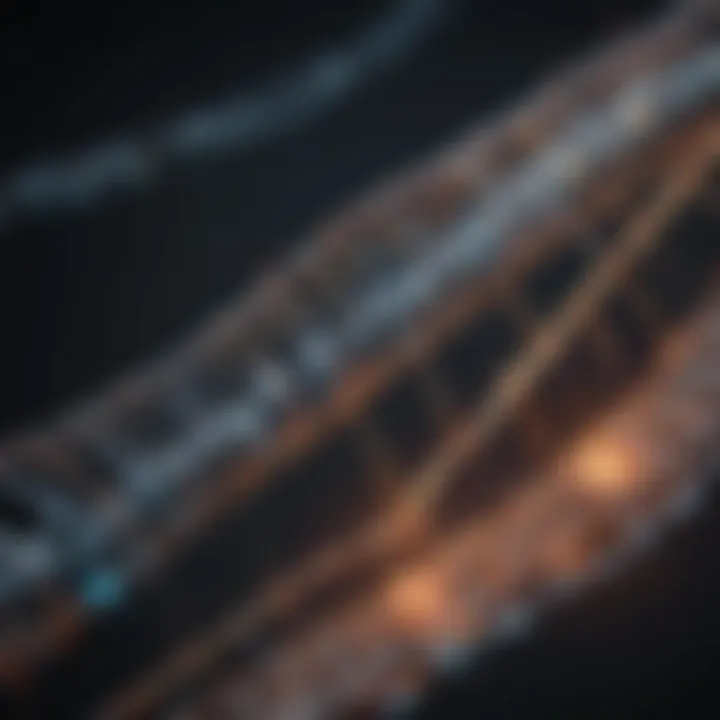
Replication begins at specific locations on the DNA molecule, known as origins of replication. The double helix unwinds, and each strand serves as a template for the creation of a new complementary strand. This mechanism is critical for cell division, as it preserves the continuity of genetic information across generations of cells. Errors during DNA replication can lead to mutations, which may have various consequences, from benign variations to significant health implications, including cancer.
"The integrity of DNA replication is crucial for genetic stability and organismal health."
In summary, mastering these fundamental concepts in genetics—the roles of genes, the structure of chromosomes, and the process of DNA replication—is vital for delving deeper into the more intricate aspects of genetic research and applications.
Mendelian Genetics
Mendelian genetics is a foundational aspect of genetic science, named after Gregor Mendel, who laid the groundwork for understanding inheritance patterns. The principles established by Mendel offer insights into how traits are passed from one generation to the next. This section discusses the importance of Mendelian genetics, its ramifications for research and practical applications in fields such as medicine and agriculture.
Mendel's work highlights a systematic approach to the study of heredity, using careful experimentation and observation.
The major benefits of understanding Mendelian genetics include:
- Predictive Power: It allows researchers to predict inheritance patterns and traits in organisms.
- Foundation for Further Research: Mendelian principles serve as a base for modern genetics, including molecular and population genetics.
- Agricultural Advances: Insights from Mendelian genetics have been pivotal in developing crops with desirable traits, enhancing food production.
The Work of Gregor Mendel
Gregor Mendel, often referred to as the father of modern genetics, conducted groundbreaking experiments in the 19th century. His choice of the pea plant as a model organism allowed him to control reproduction and observe distinct traits. Mendel meticulously documented his findings, leading to the formulation of key laws of inheritance.
His work went largely unrecognized during his lifetime but was rediscovered in the early 20th century. Mendel's observations led to the formulation of two fundamental laws:
- Law of Segregation: This law states that allele pairs separate during gamete formation, resulting in offspring receiving one allele from each parent.
- Law of Independent Assortment: This principle asserts that the segregation of alleles for one gene occurs independently of alleles for another gene.
Mendel's meticulous methodology and attention to detail laid a robust framework for future genetic research.
Principles of Inheritance
The principles of inheritance, derived from Mendel's experiments, articulate how traits are transmitted from parents to offspring. Understanding dominant and recessive alleles is core to these principles. Dominant alleles mask the effects of recessive alleles when both are present.
The key concepts include:
- Genotype vs. Phenotype:
- Homozygous vs. Heterozygous: An organism may have two identical alleles for a trait (homozygous) or two different alleles (heterozygous).
- Punnett Square: A tool used to predict the probability of genotypes in offspring based on parental alleles.
- Genotype refers to the genetic makeup of an individual, while phenotype describes the observable traits.
These principles are vital for various applications, including predicting inherited conditions and understanding evolutionary biology.
Phenotypic and Genotypic Ratios
Phenotypic and genotypic ratios are essential outcomes of Mendelian genetics that describe the expected distribution of traits. They are particularly useful in understanding inheritances such as Mendel's classic pea plant experiments.
- Phenotypic Ratio: This ratio reflects the observable traits. For instance, in a monohybrid cross of pea plants, the typical phenotypic ratio of dominant to recessive traits is 3:1.
- Genotypic Ratio: This indicates the genetic constitution of the offspring. The genotypic ratio for the same monohybrid cross is often 1:2:1, representing the homozygous dominant, heterozygous, and homozygous recessive genotypes.
Understanding these ratios can help in fields such as breeding and conservation. They offer insight into the genetic diversity present in populations and help predict future trait distributions.
In summary, Mendelian genetics serves as a pillar for the broader field of genetics, influencing both theoretical and applied sciences. By comprehending these concepts, students, researchers, and professionals can appreciate the mechanisms underlying heredity. This, in turn, enriches discussions surrounding genetics in various aspects of life.
Molecular Genetics
Molecular genetics is a critical area in the field of genetics, bridging the gap between classical genetic theory and modern biotechnology. This subsection emphasizes an understanding of the molecular mechanisms that govern genetic function and inheritance. Its significance extends beyond academic curiosity; it has profound implications on agriculture, medicine, and evolutionary biology. By exploring the structure and functions of nucleic acids and their roles in cellular processes, we gain insight into how genetic disorders arise, how organisms adapt, and how we can manipulate genes for various applications.
Structure and Function of DNA
DNA, or deoxyribonucleic acid, is the hereditary material in almost all living organisms. Its structure is a double helix, composed of two strands that wind around each other. Each strand consists of a sugar-phosphate backbone and nitrogenous bases. These bases include adenine, thymine, cytosine, and guanine. The specific sequence of these bases encodes genetic information. DNA's stability and ability to replicate are crucial for maintaining genetic continuity.
This structure allows for precision when it comes to the replication of genetic information, which is essential during cell division. Errors in this process can lead to mutations, affecting an organism's phenotype. Understanding DNA also lays the groundwork for various biotechnological advances, such as genetic testing and therapeutic interventions.
Transcription and Translation
Transcription is the process by which the genetic information encoded in DNA is transferred to messenger RNA (mRNA). This step is essential for gene expression. During transcription, RNA polymerase binds to a specific region of the DNA and unwinds the helix, creating a complementary strand of RNA. This newly formed mRNA then carries the code from the DNA to the ribosomes, the sites of protein synthesis in the cell.
Translation occurs when the mRNA is read by ribosomes to synthesize proteins. Transfer RNA (tRNA) molecules transport specific amino acids to the ribosome, where they are added sequentially to a growing polypeptide chain based on the sequence of mRNA codons. This process is vital because proteins perform a myriad of functions critical to cellular operations, including structural roles, catalyzing biochemical reactions, and signaling functions.
Gene Regulation
Gene regulation refers to the mechanisms that control the expression of genes. Not all genes are active at all times; their expression can be up-regulated or down-regulated in response to various stimuli. This complexity allows for cellular diversity and environmental responsiveness. Regulatory elements include promoters, enhancers, and silencers, which interact with transcription factors to modulate gene activity.
Understanding gene regulation is critical, especially in areas like cancer research, where gene expression pathways may be disrupted, leading to uncontrolled cell growth. Furthermore, the insights gained from studying gene regulation can inform the design of gene therapies aimed at correcting genetic disorders.
"Molecular genetics serves as the foundation for understanding how genes function, interact, and contribute to the biological processes that underpin life."
From DNA structure to gene regulation, each facet of molecular genetics is interlinked, providing a comprehensive understanding of life's complexity. This knowledge also informs the ongoing research in genetic technologies, enhancing our ability to develop innovative solutions to genetic and health-related challenges.
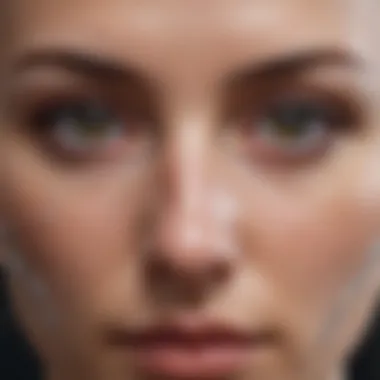
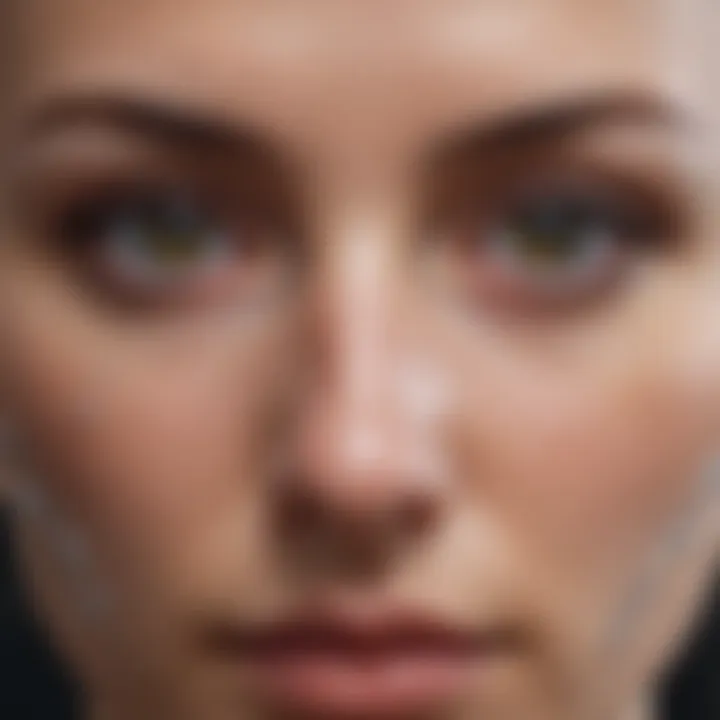
Population Genetics
Population genetics serves as a crucial branch of the field, exploring the genetic composition and evolution of populations. This section emphasizes the significance of understanding genetic diversity, allele frequency, and the evolutionary dynamics that shape populations over time. By examining populations, scientists can gather valuable insights into how genetic variation occurs and its implications for species survival and adaptability.
Genetic Variation and Adaptation
Genetic variation is fundamental to the concept of adaptation. It refers to the differences in DNA among individuals within a population. These variations can arise from mutations, gene flow, and sexual reproduction, seemingly minor processes that collectively play a significant role in shaping the genetic landscape of a species.
The importance of genetic variation lies in its direct connection to adaptation. When environmental conditions change, populations containing a wider range of genetic traits are more likely to survive and thrive. For instance, in a scenario where a disease spreads through a population, individuals with a genetic resistance will have a higher survival rate. Over time, the alleles associated with this resistance increase in frequency, demonstrating natural selection at work.
Moreover, adaptation is not limited to resistance against pathogens. It includes traits related to climate tolerance, mating strategies, and resource utilization. The interplay between genetic variation and adaptation highlights the ongoing evolutionary processes that define a species' capability to respond to environmental challenges.
"In every adaptation lies potential for not just survival, but evolution itself."
Natural Selection and Evolution
Natural selection acts as a primary mechanism driving evolution, with its roots firmly planted in population genetics. This process involves the differential survival and reproduction of individuals based on their genetic traits. In essence, those best suited to their environment tend to survive longer and produce more offspring.
A classic example illustrating this concept is the peppered moth in England. Initially, light-colored moths thrived in a landscape filled with lichen-covered trees. However, as pollution darkened the tree bark, darker moths became more common. Here, natural selection favored the darker phenotype, enhancing its chances of survival against predators. This case exemplifies how environmental changes can alter the selective pressures on a population, reinforcing the importance of understanding these dynamics in genetics.
Natural selection is closely tied to the concepts of fitness and adaptation. The term fitness refers to an organism's capacity to survive and reproduce in a specific environment. This idea establishes a connection to evolutionary success; it underscores how population genetics can predict potential future developments of species based on current genetic variability and environmental pressures.
Through studying these dynamics, researchers can better understand the evolutionary mechanisms and predict how populations might adapt or evolve in response to environmental stressors. This understanding is essential for fields such as conservation biology, where strategies can be implemented to preserve genetic diversity and enhance species resilience.
Genetic Technologies
Genetic technologies have transformed the landscape of modern genetics, enabling groundbreaking discoveries and applications. These technologies are crucial for understanding genetic principles, advancing medical treatments, and enhancing agricultural practices. Understanding how these technologies work, along with their benefits and ethical implications, is essential for anyone involved or interested in the field.
CRISPR and Gene Editing
CRISPR, or Clustered Regularly Interspaced Short Palindromic Repeats, has revolutionized the way scientists approach gene editing. This technology allows precise modifications of DNA sequences. With CRISPR, researchers can target specific genes, add or delete genes, and correct mutations.
The application of CRISPR spans various fields, including medicine and agriculture. For example, it holds promise for treating genetic disorders like cystic fibrosis or sickle-cell anemia. The efficiency and affordability of CRISPR make it accessible for many labs, leading to rapid advancements in genetic research.
Despite its advantages, CRISPR raises ethical questions. The potential for off-target effects, where unintended parts of the genome are altered, is a concern. Furthermore, gene editing in human embryos opens debates about designer babies and genetic inequalities. Thus, while CRISPR offers immense potential, it requires careful consideration of its applications and consequences.
Genomic Sequencing
Genomic sequencing involves determining the complete DNA sequence of an organism's genome. This process has become increasingly faster and cheaper, opening new avenues for scientific inquiry. By sequencing genomes, researchers can identify genetic variants linked to diseases, allowing for personalized medicine approaches tailored to individual genetic profiles.
The role of genomic sequencing extends to evolutionary biology; it helps track genetic changes over time within populations. Understanding these variations can provide insights into evolution and species adaptation. Moreover, with techniques like whole exome sequencing and targeted sequencing, researchers can focus on specific genes of interest.
Genomic sequencing technology has also found its place in forensic science. DNA profiling using genomic data can play a pivotal role in solving crimes and identifying individuals. This adaptability underscores the value of sequencing technology in both medical and forensic contexts.
Synthetic Biology
Synthetic biology is another exciting facet of genetic technologies. It involves designing and constructing new biological parts, devices, and systems. Synthetic biologists manipulate genetic components to create organisms that can perform specific tasks, such as producing biofuels or pharmaceuticals.
One significant area in synthetic biology is the engineering of microorganisms for bioremediation. These engineered microorganisms can break down environmental pollutants, offering sustainable solutions for cleaning contaminated sites.
Furthermore, synthetic biology holds potential for agricultural advancements. By creating crops with improved traits, such as increased resistance to pests or enhanced nutritional content, food security can be addressed. This technology, though promising, also prompts concerns about biodiversity and the long-term impacts of such modifications.
"Synthetic biology represents a radical shift in how we understand and manipulate life on Earth."
Ethical Considerations in Genetics
Ethical considerations in genetics are increasingly vital as we navigate the complexities of modern genetic technologies. These considerations encompass rights, responsibilities, and the implications of genetic knowledge. As our understanding of genetics expands, so do the debates surrounding its uses and effects on society. It is essential to address these issues thoroughly, as they impact not only individuals but also communities and future generations. Understanding the ethical dimensions helps to foster responsible practices and informed discussions around genetics.
Genetic Discrimination
Genetic discrimination refers to the unfair treatment of individuals based on their genetic information. This can occur in various contexts, such as employment, insurance, and healthcare. Employers might look at genetic predispositions to certain illnesses and make hiring decisions based on this information. Similarly, insurance companies might deny coverage or charge higher premiums to individuals identified as at-risk.
In the face of such risks, laws like the Genetic Information Nondiscrimination Act in the United States have been established to protect individuals. However, enforcement remains complex, and many argue that more comprehensive regulations are necessary to prevent genetic discrimination from occurring.
Informed Consent in Genetic Testing
Informed consent is a cornerstone of ethical medical practice. In the field of genetics, this means that individuals should have a complete understanding of what genetic testing entails and the potential consequences of the results. This includes, but is not limited to, their rights, data privacy, possible outcomes, and implications for family members.
• Transparency: Genetic testing companies must be transparent about what data is being collected and how it will be used. • Awareness: Patients must be made aware of their options and the various pathways following test results. • Voluntariness: Consent must be given freely and not coerced.
Clear guidelines and transparent communication must be prioritized to respect the autonomy of individuals undergoing genetic testing.
Implications for Genetic Engineering
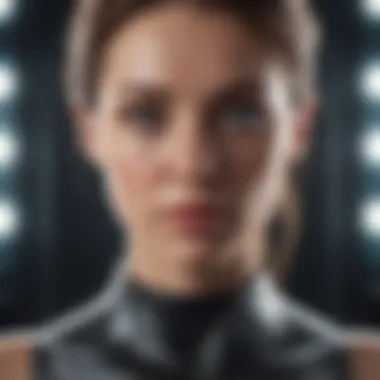
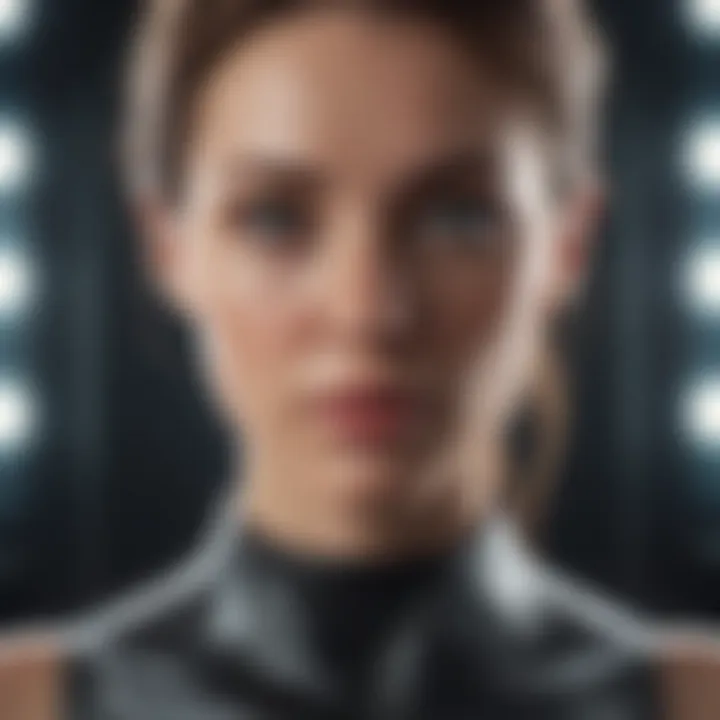
Genetic engineering presents remarkable potential for innovation in medicine, agriculture, and beyond. However, it also raises significant ethical concerns. As we manipulate genes, questions emerge about the limits of such interventions. Are we ready to modify the human genome? What are the possible risks of creating genetically modified organisms?
The implications extend beyond individual choices to societal norms. Society must grapple with questions about how to balance the benefits of genetic engineering with inherent risks. Potential regulatory frameworks must be developed to ensure that genetic engineering advances human well-being without compromising ethical values.
"The future of genetics will not just be about what we can do with our technology, but also about what we should do with it."
In summary, the ethical considerations surrounding genetics are profound and multifaceted. They prompt essential dialogues about discrimination, consent, and engineering. Addressing these issues will lead to better practices and a more equitable society.
Applications of Genetics
The field of genetics extends far beyond simple inheritance patterns. Its applications have profound implications across various sectors, including medicine, agriculture, and forensic science. This section examines the practical uses of genetics, exploring its benefits and considerations while emphasizing its significance in modern society.
Genetics in Medicine
Genetics plays a crucial role in medicine, helping to understand disease mechanisms, personalize treatment plans, and enhance preventive care. One of the notable applications is in genetic testing, which allows for the identification of mutations associated with specific hereditary diseases. This early detection can guide healthcare providers in making informed decisions about patient care.
Gene therapy is another transformative aspect. By targeting genetic defects, this approach aims to correct abnormalities at the molecular level, offering potential cures for previously untreatable conditions. Additionally, pharmacogenomics examines how an individual's genetic makeup influences their response to medications. This field promises more effective and safer drug prescriptions tailored specifically to patients, reducing the trial-and-error approach in traditional medicine.
Agricultural Genetics
In agriculture, genetic applications significantly contribute to food security and sustainability. The use of genetic modification enables scientists to enhance crop resilience against pests and diseases, ensuring higher yields. For example, genetically engineered varieties such as Bt corn produce a toxin that is harmful to specific insects, reducing the need for chemical pesticides.
Moreover, plant breeding techniques have evolved, leading to hybrid varieties that exhibit desirable traits. These innovations enable farmers to cultivate crops that thrive in varying environmental conditions, which is crucial given the challenges posed by climate change. Agricultural genetics also involves animal husbandry, where genetic selection can improve livestock quality, leading to better growth rates and disease resistance.
Forensic Genetics
Forensic genetics applies genetic analysis techniques in the legal realm, aiding criminal investigations. DNA profiling has revolutionized how law enforcement agencies identify and convict offenders. This method involves analyzing specific regions of an individual's DNA to create a unique profile, which can be matched to samples collected from crime scenes.
Such applications extend beyond criminal cases. Paternity testing frequently employs similar techniques to establish biological relationships. Another area of forensic genetics is wildlife forensics, where genetic tools are used to combat poaching and illegal wildlife trade by identifying species from biological samples. The ability to analyze DNA provides critical evidence that can influence legal decisions and promote conservation efforts.
Overall, the applications of genetics are vast and multifaceted, influencing various aspects of human life and the environment. As research continues to advance, the potential for genetics to contribute solutions to pressing global challenges remains promising.
Future Directions in Genetics Research
The field of genetics is continuously evolving. Future directions in genetics research are crucial to understanding how these advancements can impact healthcare, agriculture, and our understanding of human biology. By investigating new technologies and methodologies, researchers aim to unlock the potential of genetics. This section outlines the significance of emerging technologies, potential discoveries, and interdisciplinary approaches in shaping the future of genetic research.
Emerging Technologies
Emerging technologies in genetics are fundamentally changing how researchers approach genetic studies. Techniques like CRISPR-Cas9 have revolutionized gene editing. Researchers can now alter specific genes with precision. This capability promises significant advancements in treating genetic disorders, enhancing agricultural productivity, and even combating climate change. Other technologies like next-generation sequencing allow scientists to decode entire genomes quickly and affordably.
Benefits of these technologies:
- Increased accuracy in gene editing.
- Rapid data generation for genomic studies.
- Enhanced ability to identify genetic variants linked to diseases.
These tools do not just simplify research; they provide a platform for major breakthroughs. As technologies improve, the ability to manipulate genetic material will likely lead to further advancements in applications ranging from medicine to environmental science.
Potential Discoveries
The future of genetic research holds the promise of transformative discoveries. Investigations into the human microbiome are one area ripe for exploration. The microbiome's influence on health and disease suggests a significant link, which may lead to personalized treatment plans.
Additionally, areas such as epigenetics reveal how genes are expressed differently based on environmental factors. Understanding these mechanisms better could result in novel therapeutic approaches. As scientists delve deeper, potential discoveries could not only advance science but also offer solutions to pressing health and environmental issues.
"Future discoveries in genetics may hold the key to curing currently untreatable diseases."
Interdisciplinary Approaches
Interdisciplinary approaches combining genetics with other fields, such as bioinformatics, developmental biology, and ethics, will amplify the impact of genetic research. Collaborations across disciplines allow for a more comprehensive understanding of complex systems. Bioinformatics, for instance, enhances data analysis, interpreting genetic data quickly and accurately.
Such collaboration is necessary for ethical considerations as research progresses. Employing an interdisciplinary framework ensures that as new technologies emerge, ethical guidelines evolve similarly. This integrated approach paves the way for responsible advancements in genetics.
Closure
The conclusion of this article serves as a crucial element in wrapping up the extensive discussion on genetics. It summarizes key concepts while emphasizing their relevance in both scientific and societal contexts. Such summarization not only reinforces the knowledge gained but also highlights the potential future directions in genetics research.
Recapitulation of Key Points
In reviewing the significant aspects of genetics covered in this article, several key points emerge:
- Foundational Principles: Genetics is centered on the study of genes and heredity. Understanding the basic concepts of DNA, genes, chromosomes, and how they function is essential in grasping complex phenomena related to genetics.
- Mendelian Genetics: The principles established by Gregor Mendel laid the groundwork for the modern understanding of inheritance. Mendel's work underscores how traits are passed from one generation to the next, forming a basis for both classical and molecular genetics.
- Technological Advances: Emerging technologies like CRISPR and genomic sequencing have transformed genetic research, allowing for precise manipulations of DNA and better understanding of genetic sequences. These innovations push the boundaries of what is possible in areas like medicine and agriculture.
- Ethical Considerations: The rapid advancements in genetic science bring significant ethical considerations. Topics such as genetic discrimination and informed consent in genetic testing are paramount, urging society to engage critically with the implications of such technologies.
- Future Directions: The potential for future discoveries in genetics is vast. Emerging interdisciplinary approaches promise to unlock even more insights, which could lead to breakthroughs in treating genetic disorders, enhancing food security, and addressing various challenges posed by climate change.
The Importance of Genetics in Society
Genetics plays a pivotal role in contemporary society. Its relevance spans various sectors, impacting health, agriculture, and even forensic science. By understanding genetics, individuals and communities can make informed decisions related to health care, agricultural practices, and personal choices regarding genetic traits.
Moreover, genetics challenges our ethical frameworks, compelling us to consider questions about identity, privacy, and inequality.
"As we navigate this landscape, the necessity of robust ethical guidelines becomes clear. Genetics has the power to create significant advancements, but it also requires responsible stewardship."
In summary, genetics is not just confined to scientific discourse; it is embedded within the tapestry of societal evolution. The discussions surrounding genetics will only become more pronounced as technology advances, making it imperative for individuals at all levels to engage with and understand its complexities. Continuing the dialogue on genetics ensures that we harness its potential while mitigating risks associated with misuse and misunderstanding.