Exploring the Maya Spectrometer: Principles and Applications

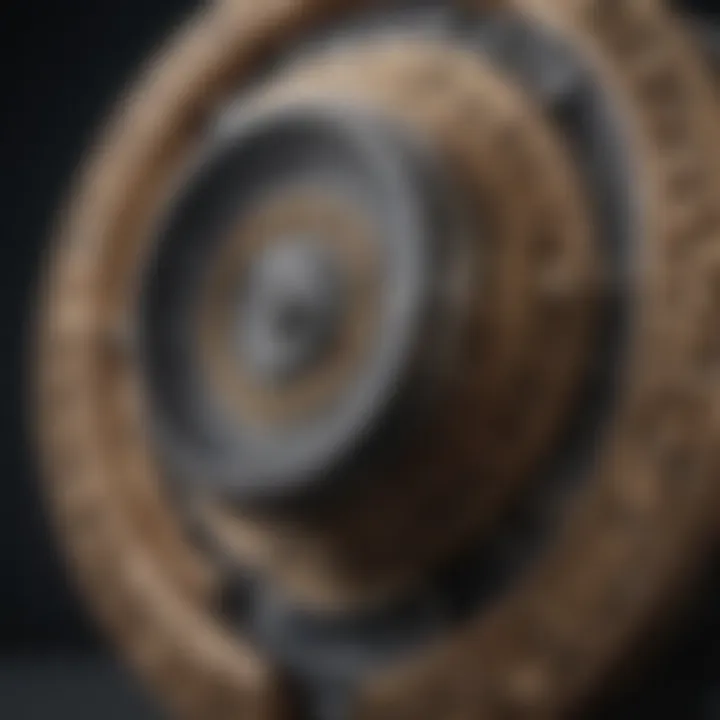
Intro
The Maya spectrometer stands as a pivotal instrument in various scientific arenas, revolutionizing methodologies for analyzing materials and understanding their properties. This article seeks to shed light on the principles, design, and multifaceted applications of the Maya spectrometer. From the intricacies of its engineering to the scope of its utility across diverse fields such as biology, chemistry, physics, and earth sciences, this comprehensive guide aims to equip students, educators, and researchers with an in-depth insight into this analytical marvel.
By unraveling the working mechanics behind the Maya spectrometer, we explore its capabilities and limitations. Moreover, considerations related to recent advancements and ongoing research in spectroscopic techniques will be discussed to place the instrument within the current scientific context. This endeavor serves not only as a resource but also as an invitation into the fascinating world of spectrometry, characterized by continuous innovation and inquiry.
Research Highlights
Overview of Key Findings
The Maya spectrometer employs innovative techniques to provide high-resolution spectral data across a range of wavelengths. Key findings of recent studies indicate that the device enhances the accuracy of spectral analysis significantly. Several research projects have demonstrated its application in detecting complex biochemical markers and analyzing material compositions with unprecedented precision. Neoteric features like integrated software for data analysis have also been noted, facilitating easier interpretation of results, thus adding value to inter-disciplinary research.
Significance of the Research
The relevance of research involving the Maya spectrometer extends far beyond academic interest. It lays the groundwork for advancements in critical areas, including environmental monitoring and medical diagnostics. The ability to analyze samples rapidly affects real-time decision-making in various industries. This significance is underscored by the instrument's alignment with current needs in data analysis and material science.
Advancements in Spectroscopic Techniques
Recent advancements in spectroscopic technology have prompted developments in the Maya spectrometer's design. Improvements in detector sensitivity and data processing speed allow for a more comprehensive array of spectral analysis. These enhancements enable scientists to conduct intricate experiments that were once deemed impractical.
Applications Across Disciplines
The versatility of the Maya spectrometer knows no bounds. In biology, it aids in the identification of biomolecules crucial for understanding diseases. In chemistry, it offers insights into reaction mechanisms. Physics utilizes this instrument for material characterization, while earth sciences benefit from its capacity to analyze environmental samples, improving soil and water testing methodologies. Each application underscores the instrument's role not merely as a tool of analysis but as a critical enabler of scientific progress.
"Understanding the Maya spectrometer provides a gateway to unlocking the potential present in various scientific disciplines."
Challenges and Future Directions
Despite its myriad applications, challenges persist in the efficient utilization of the Maya spectrometer. Calibration complexities and the need for user training may hinder its adoption among less experienced researchers. The future will likely see enhanced training resources and simplified calibration processes. Additionally, the focus on miniaturization of spectroscopic devices could open new avenues for in-field analyses and studies.
Epilogue
The Maya spectrometer represents a convergence of technology and scientific inquiry, offering extensive potential for analysis across various disciplines. As advancements continue, understanding this instrument's principles and applications will remain critical in addressing both current and future scientific challenges.
Prologue to Spectroscopy
Spectroscopy represents a pivotal domain within the scientific landscape, providing essential insights into the composition of matter. In this article, we explore this critical facet of analytical science, emphasizing its relevance to various applications. The ability to discern chemical and physical properties through spectral data confirms the significance of spectroscopy in evolving scientific methodologies. Furthermore, the advent of sophisticated instruments, like the Maya spectrometer, revolutionizes how researchers approach material analysis across disciplines.
Definition and Scope
Spectroscopy is essentially the study of the interaction between matter and electromagnetic radiation. Its scope has broadened considerably, encompassing a wide range of techniques adapted for diverse applications. Techniques such as infrared spectroscopy, ultraviolet-visible spectroscopy, and nuclear magnetic resonance spectroscopy serve various industries and research domains.
The definition of spectroscopy captures its thematic essence but also underscores a scope that is continually expanding. Each spectroscopic method varies significantly in how it probes the structural, electronic, and vibrational characteristics of materials. By analyzing spectra, chemists can identify materials, quantify concentrations, and elucidate structural information, making it indispensable in fields like chemistry, biology, environmental science, and even astrophysics.
Historical Background
The journey of spectroscopy traces back to the early scientific inquiries of the 17th century. Sir Isaac Newton’s experiments with prisms laid the groundwork by demonstrating that white light can be dispersed into a spectrum of colors. This was a along path for the later developments by pioneers like Joseph von Fraunhofer, who, in the early 19th century, provided insights into the absorption spectrum of sunlight.
The evolution of this field continued through the ages, with advancements in optical technology enabling more refined and accurate measurements. The advent of quantum mechanics in the 20th century further propelled the discipline, allowing scientists to link energy transitions of electrons with specific wavelengths of light. Today, spectroscopy embraces a plethora of techniques, enhanced by computer technology and advanced detectors, shaping modern-day applications and research.
Spectroscopy is not just a tool; it is a gateway to understanding our universe through the analysis of light and matter.
Since its inception, spectroscopy has evolved immensely, giving rise to specialized fields such as molecular spectroscopy, atomic spectroscopy, and mass spectrometry, each opening new vistas in scientific exploration. As we delve deeper into the specifics of the Maya spectrometer, understanding this foundational topic of spectroscopy becomes not just beneficial but essential for appreciating the instrument's capabilities and application potential.
Overview of the Maya Spectrometer
The Maya spectrometer stands as a pivotal instrument in modern analytical science. Understanding its significance provides insight into its applications across various fields. This section discusses what the Maya spectrometer is and its central components, enabling a comprehensive exploration of its role in scientific research.
What is the Maya Spectrometer?
The Maya spectrometer is an advanced tool designed for analyzing the spectral composition of light from various sources. Spectroscopy involves studying the interactions between light and matter. The spectrometer captures these interactions, translating light into readable data.
Employing a range of techniques, the Maya spectrometer detects and quantifies the presence of different compounds, making it invaluable in numerous disciplines. Fields such as biology, chemistry, and environmental science benefit from its capabilities. By employing precise wavelength measurements, researchers can identify substances, investigate material properties, and analyze chemical reactions. Ultimately, the Maya spectrometer offers a robust methodology for authors to gain detailed insights into the materials they study.
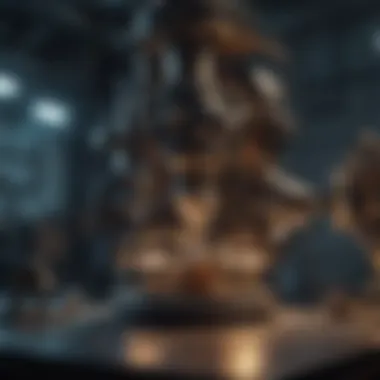
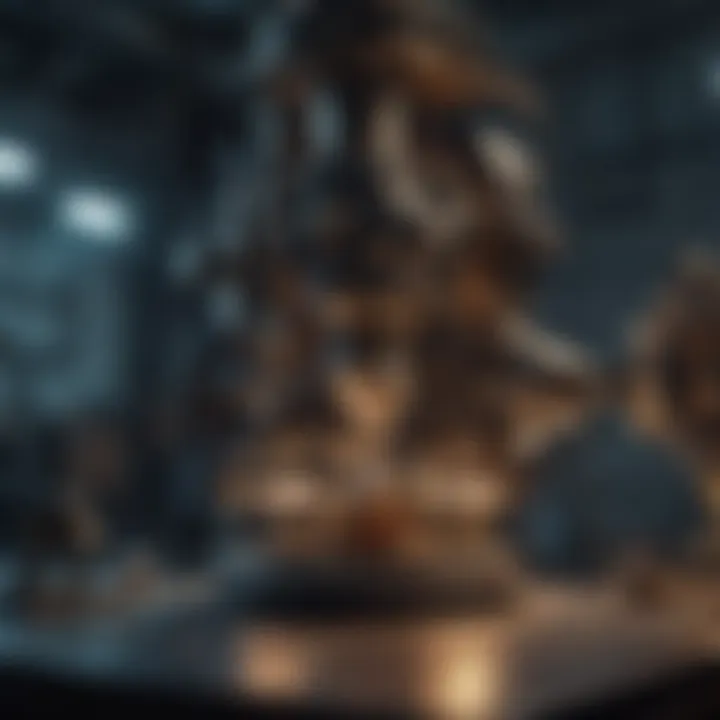
Core Features and Components
The Maya spectrometer comprises several key features that enhance its functionality. Understanding these features is essential for effective use and application:
- Wavelength Range: The spectrometer operates across an extensive range of wavelengths, making it versatile for various applications.
- Detector Type: Commonly, these devices employ CCD or photodiode detectors. These components capture light signals efficiently and convert them into digital data.
- Optical Elements: Lenses, prisms, and gratings within the spectrometer are crucial in dispersing light into its constituent wavelengths. Each optical element plays a specific role in refining the data.
- Data Acquisition System: This system ensures accurate data collection and storage, allowing users to analyze results further. The software often provided enhances the user experience by presenting data in a manageable format.
Understanding these core elements not only aids in operating the Maya spectrometer but also emphasizes its critical role in various research applications. The integration of advanced technology ensures that the spectrometer remains an essential instrument for scientists worldwide.
"The Maya spectrometer transforms light into knowledge, bridging the gap between observation and understanding in scientific research."
In summary, this overview outlines the significance of the Maya spectrometer in various disciplines. By examining its operational mechanisms and core components, we establish the groundwork for understanding its impressive scope of application across scientific fields.
Principles of Operation
Understanding the principles of operation is essential for grasping the functionality of the Maya spectrometer. This section outlines the operational frameworks that enable the spectrometer to analyze spectral data with precision. The effectiveness of any spectroscopic instrument relies significantly on its operational principles. Thus, elucidating these mechanisms will highlight the importance of accuracy in spectral measurements and the impact of calibration on results.
Spectral Analysis Mechanisms
Spectral analysis mechanisms in the Maya spectrometer are fundamental to its operation. At the core, the spectrometer employs diffraction gratings or prisms to separate incoming light into its constituent wavelengths. Each wavelength corresponds to a specific energy state of a molecule, allowing scientists to gain insights into chemical compositions and molecular structures. This means that by analyzing the light emitted or absorbed by a substance, critical information can be inferred concerning its molecular components.
The process begins when a light source illuminates a sample. As light interacts with the sample, certain wavelengths are absorbed while others are transmitted. The spectrometer captures this information. The separation of wavelengths allows for the creation of a spectrum, which visualizes the intensity of light at different wavelengths. This spectrum serves as a unique fingerprint for each substance, enabling identification and quantitative analysis of compounds.
Factors such as resolution, sensitivity, and optical design critically affect the performance and accuracy of spectral analysis. A higher resolution means finer details can be seen, critical for distinguishing closely lying spectral lines. Sensitivity indicates how faint a signal the instrument can detect and is vital for measuring trace substances.
Understanding these mechanisms is crucial because it informs users about the limitations and strengths of the spectrometer. For instance, inappropriate settings or faulty components can lead to misinterpretation of data, potentially skewing research outcomes.
Calibration Techniques
Calibration is crucial for maintaining the accuracy and reliability of the Maya spectrometer. This section explores the processes involved in ensuring that the spectral measurements reflect true values.
Traditionally, calibration involves the use of standard reference materials with known spectral properties. These materials are analyzed with the spectrometer so that any discrepancies can be noted and corrected. Regular calibration is recommended, as the instrument's performance can shift due to various factors such as temperature changes, mechanical wear, or environmental conditions.
There are several established calibration techniques:
- Wavelength Calibration: This ensures that the spectrometer correctly identifies the wavelengths of light. A known light source such as a mercury lamp can be utilized for this.
- Intensity Calibration: This is performed to ensure that the intensity of the measured spectra corresponds accurately to the actual concentration of substances within the sample. This often employs solutions of known concentrations.
- Baseline Correction: Instruments often require background noise to be accounted for, which can be done through baseline correction methods during calibration. This process improves data clarity and accuracy.
A consistent calibration process can greatly enhance the quality of results. It allows for comparability across different measurements and ensures that data interpretation remains valid. By adopting robust calibration techniques, researchers can make credible conclusions based on the data they gather using the Maya spectrometer.
Technical Specifications
The topic of technical specifications is crucial in understanding the Maya spectrometer. These specifications define how the instrument operates, its capabilities, and its limitations. This section will delve into the design specifications and examine the sensitivity and resolution that exemplify the performance of the Maya spectrometer. Understanding these elements not only enhances comprehension of the tool but also informs potential users about its applicability.
Design Specifications
The design specifications of the Maya spectrometer encompass several fundamental aspects, including the physical dimensions, optical layout, and material composition. The overall architecture is typically compact, allowing for portability without compromising functionality. A well-thought-out optical design is essential for maximizing light capture and minimizing interference, which can distort results.
Key components often outlined in design specifications include:
- Optical fibers: used for light collection and transmission.
- Optics: lenses and gratings positioned precisely to enhance spectral dispersion.
- Detector: often a CCD sensor, chosen for its sensitivity and rapid response time.
It is imperative that potential users pay attention to these specifications when choosing a spectrometer for specific applications. For instance, if a research project requires field measurements, lightweight and robust design features will be more beneficial than a high-end, lab-based setup. A good understanding of these technical aspects aids researchers in selecting the right instrument tailored to their needs.
Sensitivity and Resolution
Sensitivity and resolution are two of the most critical performance indicators of the Maya spectrometer. Sensitivity refers to the instrument's ability to detect low concentrations of substances or minor changes in signals. A highly sensitive spectrometer can significantly impact the quality of research findings, especially in fields such as biochemical analysis.
Resolution, on the other hand, relates to the spectrometer's capability to distinguish between closely spaced spectral lines. High resolution ensures detailed spectral information, which is vital in applications like material identification or environmental monitoring. Factors influencing sensitivity and resolution include:
- Detector Quality: Higher quality detectors yield better sensitivity.
- Optical Design: An optimized optical layout can greatly enhance resolution.
- Calibration Methods: Regular calibration ensures consistent performance and accurate results.
Users must evaluate these specifications when planning experiments. A balance between sensitivity and resolution is often necessary, as priorities may shift depending on the application.
A detailed understanding of technical specifications will empower researchers to leverage the full capabilities of the Maya spectrometer in their scientific inquiries.
Applications of the Maya Spectrometer
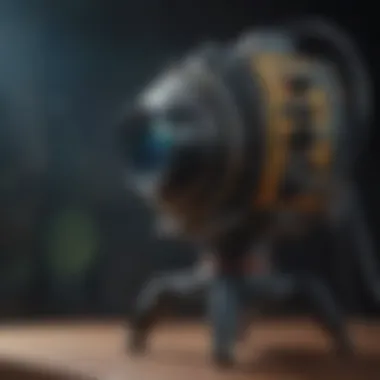
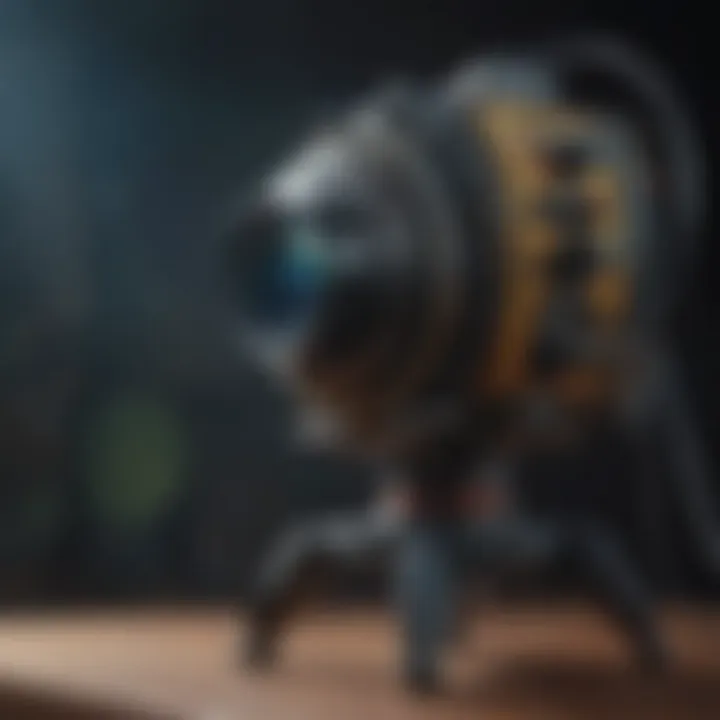
The Maya spectrometer plays a crucial role in numerous scientific disciplines, making its applications noteworthy for students, researchers, and educators. Its versatility allows it to be used in numerous fields, each yielding significant advancements and insights. The proper utilization of this instrument not only enhances research quality but also pushes the boundaries of knowledge in both basic and applied sciences.
In biological analysis, the Maya spectrometer assists in understanding the molecular composition of biological samples. This capability allows researchers to detect specific compounds, identify metabolic changes, and study complex biochemical pathways. Such analysis proves valuable in medical research, diagnostics, and environmental studies.
Chemical investigations benefit from the Maya spectrometer's keen ability to delineate chemical structures and reactions. It enables chemists to quantify the concentration of substances in mixtures, facilitating more informed decisions in both academia and industry. This precision in chemical analysis supports both fundamental research and practical applications in fields like pharmaceuticals and materials science.
In planetary science, this spectrometer aids in exploring celestial bodies and their composition. By analyzing the light spectra from planetary surfaces or atmospheres, researchers can deduce the presence of elements and compounds, which can inform our understanding of planetary formation and evolution.
Materials characterization is another essential application of the Maya spectrometer. It helps in determining the properties of materials such as purity, phase composition, and structural information. Industries that rely on standards of quality can use this information to enhance product reliability and performance.
Each of these applications underlines the significance of the Maya spectrometer in contemporary scientific inquiry, marking its place as an indispensable tool across various research fields.
Biological Analysis
Biological analysis using the Maya spectrometer is pivotal for advancing our understanding of complex living systems. The spectrometer can analyze proteins, nucleic acids, and metabolites with high sensitivity. This enables researchers to uncover key biochemical pathways and mechanisms underlying various biological phenomena.
Some benefits of utilizing the Maya spectrometer in biology include:
- Non-destructive testing: The spectrometer can objectively analyze samples without damaging them.
- Multi-dimensional analysis: It can analyze multiple components simultaneously, saving time and enhancing throughput.
- Refined technique: Techniques like fluorescence spectroscopy can be applied, allowing for detailed cellular studies.
Overall, this technology is an essential ally for bioscientists working on diagnostics, drug development, and environmental research.
Chemical Investigations
In chemical investigations, the Maya spectrometer holds significant value, especially for its analytical capabilities. It provides insight into the composition and structure of chemical compounds, offering precise quantification of materials.
Key aspects of using the Maya spectrometer in chemical research include:
- Quantitative analysis: This method allows for accurate measurement of concentration for various substances in mixtures.
- Reaction dynamics: Observing real-time chemical changes is possible, aiding in understanding reaction mechanisms.
- Complex mixtures disentangling: The ability to analyze complex mixtures lets chemists navigate challenging systems, yielding useful chemical data.
These features lend themselves well to applications in industries such as pharmaceuticals, petrochemicals, and food sciences.
Planetary Science
The application of the Maya spectrometer in planetary science is groundbreaking. Spectrometry helps in analyzing the surface and atmospheric compositions of celestial bodies. This data is fundamental for comparing their features to Earth and assessing their potential for habitability.
Using the Maya spectrometer, scientists can achieve:
- Composition analysis: Identifying minerals and gases on planetary surfaces.
- Remote sensing: Observing distant celestial bodies without direct contact, crucial for space exploration missions.
- Astrobiological implications: The instrument can spot organic compounds that hint at past or present life.
Such applications are significantly advancing our knowledge of the solar system and beyond.
Materials Characterization
Materials characterization is another vital use of the Maya spectrometer. This instrument provides detailed information about the physical and chemical properties of various materials.
Benefits of using the spectrometer in materials science consist of:
- Quality assurance: Ensuring materials meet defined standards, crucial for manufacturers.
- Improved research: Gathering insights on molecular structures can inform the development of new materials.
- Surface analysis: Examining surface properties can lead to improved coatings and treatments in manufacturing.
This application is key for industries such as aerospace, automotive, and construction, enhancing product development and innovation.
The wide-ranging applications of the Maya spectrometer illustrate its importance and adaptability in modern scientific research. From biology to materials science, it is an essential tool that enriches our scientific capabilities and understanding.
Recent Developments in Spectrometry
Recent advances in spectrometry have crucial implications for enhancing the functionality and applications of instruments like the Maya spectrometer. These developments are pivotal in fostering more accurate, efficient, and versatile measurements across various scientific fields. As technology evolves, the integration of new methods and improvements becomes essential. This section sheds light on the most significant technological trends and software upgrades that have influenced contemporary spectrometry.
Technological Innovations
Technological innovations in the realm of spectrometry are advancing at a rapid pace. These innovations encompass a range of breakthroughs that expand the capabilities of instruments. One notable example is the introduction of miniaturized spectrometers, which retain high performance while being compact and cost-effective. This has increased accessibility for educational institutions and research facilities. Moreover, the integration of nanotechnology has led to significant enhancements in sensitivity and detection limits, allowing researchers to analyze samples with unprecedented precision.
The adoption of multi-channel and multiplexing techniques has also revolutionized spectral data acquisition. This enables simultaneous measurement of multiple wavelengths, reducing analysis time while improving data quality. Additionally, advancements in laser technology, such as the development of laser-induced breakdown spectroscopy (LIBS), provide more accurate elemental analysis, which is particularly beneficial in materials characterization.
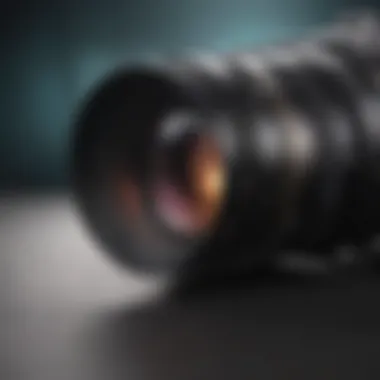
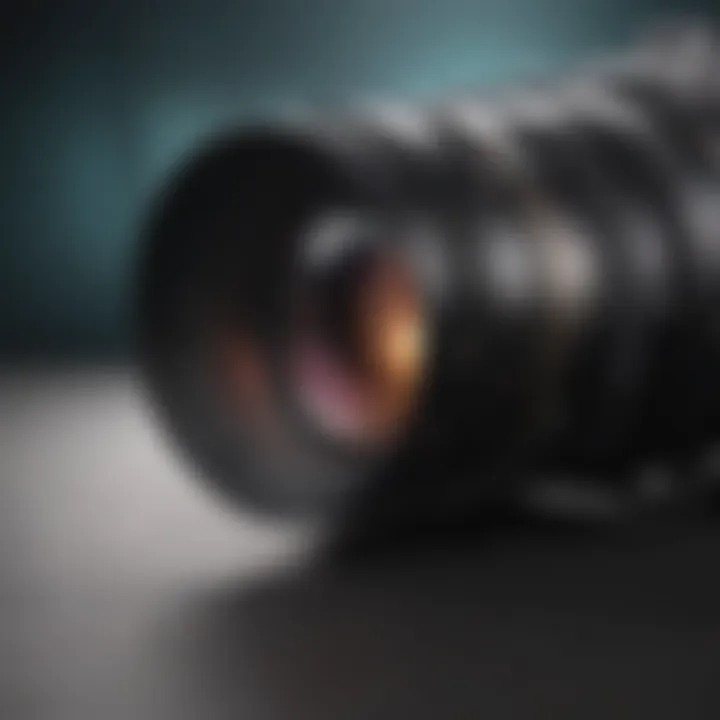
Software Improvements
On the software front, recent improvements have made a considerable impact on spectrometric analysis. Cutting-edge data processing algorithms have emerged, allowing for better noise reduction and peak identification. These enhancements aid researchers in interpreting complex spectra more accurately and efficiently.
Modern spectrometers now often come equipped with sophisticated software that supports Machine Learning techniques. This allows for pattern recognition and predictive analytics, substantially improving the analysis process. Users can benefit from real-time data analysis, facilitating instant feedback during experiments. Furthermore, enhanced graphical user interfaces (GUIs) make it easier to operate the spectrometer and interpret data, which is especially important for users who may not possess extensive expertise in spectroscopy.
Challenges in Spectroscopic Techniques
Understanding the challenges in spectroscopic techniques is crucial for advancing the field. Despite the remarkable capabilities of instruments like the Maya spectrometer, several barriers remain—especially in terms of instrumentation limitations and data interpretation.
Instrumentation Limitations
The Maya spectrometer, like any analytical device, is subject to various limitations. These limitations often relate to the technology’s inherent characteristics and operational constraints. For instance, a common issue is the sensitivity of the instrument. High sensitivity is necessary for detecting subtle spectral features, but achieving that in practice can be difficult. Environmental factors such as temperature and humidity can affect the performance of the spectrometer. Additionally, the quality of the optical components plays a significant role in determining resolution and overall performance.
The cost of high-end components can limit accessibility for some researchers. This is increasingly important in educational settings, where budget constraints might prevent the purchase of optimal equipment. Limited funding can restrict students and educators from fully exploiting advanced spectroscopic techniques.
Furthermore, there can be issues with the signal-to-noise ratio. Background noise can obscure real signal, making it difficult to obtain clear results. This challenge is compounded in complex sample matrices, where multiple substances may interfere with spectral readings.
Data Interpretation Issues
Even when instrumentation functions beautifully, the interpretation of spectral data remains a complex task. Spectral data can produce a significant volume of information, leading to potential for misinterpretation. Certain peaks in the spectra might represent noise rather than actual data about the sample being analyzed. When inexperienced users handle data analysis, the risk of misidentification increases.
Software used for data analysis can also present challenges. While there have been strides in improvements, some programs do not fully account for all variables during data interpretation. This oversight can yield inaccurate conclusions about sample composition or properties. Additionally, the complexity of the software may be daunting for those new to spectroscopy, requiring significant training or experience to master.
Many researchers encounter difficulties because they lack a strong foundational knowledge of spectroscopic principles. This ignorance can lead to reliance on faulty assumptions, skewing results further. The sharing of best practices and collaborative learning is essential to mitigate these issues.
"Technological advancements do not guarantee accurate results. It is critical to enhance understanding among users for effective application."
In summary, while the technology behind the Maya spectrometer is sophisticated, challenges remain. A focus on overcoming instrumentation limitations and enhancing data interpretation skills can greatly improve outcomes in scientific research.
Future Perspectives in Spectroscopy
The future of spectroscopy holds vast potential, promising to enhance our understanding and manipulation of various scientific phenomena. Spectroscopy plays a vital role in multiple domains such as environmental science, materials engineering, and health diagnostics. The continuous evolution of technology necessitates that scientists and researchers continuously adapt and refine their methodologies to harness the full capabilities of advanced spectrometric techniques. As we explore these future perspectives, it becomes clear that an insight into emerging trends and new applications will be essential.
Emerging Trends in Research
In the realm of spectroscopy, several emerging trends are garnering attention.
- Integration of Artificial Intelligence: Machine learning algorithms can analyze complex datasets faster and more accurately than traditional methods. The implementation of AI aims to automate spectral analysis, improve noise reduction, and enhance data interpretation.
- Miniaturization of Instruments: Advances in nanotechnology enable the development of portable spectrometers. Smaller devices can offer researchers the ability to conduct analyses in situ, reducing the need for sample transportation and minimizing contamination risks.
- Multimodal Spectroscopy: Combining different spectroscopic techniques, such as Raman and infrared spectroscopy, allows for a more comprehensive analysis of materials. This trend facilitates identifying components in complex samples, offering enhanced specificity and sensitivity in detection.
These trends reflect a shift towards more efficient, precise, and accessible spectroscopic methods that can meet the diverse needs of researchers today.
Potential New Applications
The expansion of spectroscopy's applications appears promising. Here are several noteworthy considerations:
- Environmental Monitoring: Innovative spectroscopic methods could enhance the detection of pollutants and toxins in air and water. For example, imaging spectroscopy allows for tracking changes in ecosystems efficiently.
- Medical Diagnostics: Non-invasive diagnostic techniques using spectroscopy can revolutionize how diseases are detected early. Techniques like fluorescence spectroscopy can identify biomarkers for various conditions, improving outcomes for patients.
- Food Safety and Quality Control: Spectroscopic methods can ensure food safety by quickly analyzing nutritional content or detecting harmful substances. With the increased focus on food safety, these applications hold substantial relevance.
The advancements in spectroscopy, driven by technology and innovation, hold the promise of extensive applications that can significantly transform scientific research.
While this field evolves, it is crucial to remain aware of ethical considerations and challenges that may arise. As spectroscopy advances, collaboration among scientists, industry experts, and regulatory bodies becomes increasingly important to ensure responsible development and application.
Finale
The conclusion serves as a crucial segment in this article, encapsulating the essence of the exploration of the Maya spectrometer. It's the final opportunity to reflect on its significance and the insights gleaned through this extensive discussion. This section emphasizes the critical role of the Maya spectrometer across various scientific domains, from biological analysis to planetary science.
With advancements in spectrometry, the Maya spectrometer stands out for its precise measurements and versatility. These characteristics are not just beneficial, they highlight the growing demand for sophisticated analytical tools in modern research. By synthesizing key points from previous sections, this conclusion aims to reinforce the instruments' applications and encourages ongoing inquiry into its capabilities.
Summary of Key Points
- Core Principles: The article elucidates the fundamental principles of how the Maya spectrometer functions, including its mechanisms for spectral analysis and calibration techniques.
- Design and Components: An overview of the technical specifications and design elements that contribute to the instrument's effectiveness was examined.
- Broad Applications: The diverse applications across biological, chemical, and planetary science fields was outlined, underscoring its relevance in various research settings.
- Challenges and Developments: Challenges in instrumentation and data interpretation were discussed alongside recent technological advancements, providing a balanced view of its utility.
- Future Directions: Emerging trends and potential new applications were identified, suggesting a vibrant future for research using this instrument.
Implications for Future Research
The implications for future research are substantial. With the increasing complexity of scientific inquiries, the need for robust analytical tools like the Maya spectrometer is essential. Upcoming research could explore enhancements in sensitivity and instrumentation, which are pivotal to advancing scientific knowledge.
Moreover, interdisciplinary applications could reveal insights previously unobtainable. As researchers fine-tune analytical methods, there are opportunities to innovate in fields such as environmental monitoring, materials science, and even our understanding of extraterrestrial environments.
In the long term, developing better calibration procedures and software improvements can maximize the potential of the Maya spectrometer. Attention should also focus on enhancing user accessibility, ensuring that even those less experienced with spectrometry can utilize its full capabilities.
In summary, the Maya spectrometer is more than just an analytical tool; it is a gateway to enhancing scientific understanding across disciplines. Its future in research promises not only continued relevance but also transformative potential.