Unveiling the Most Powerful Microscopes Today
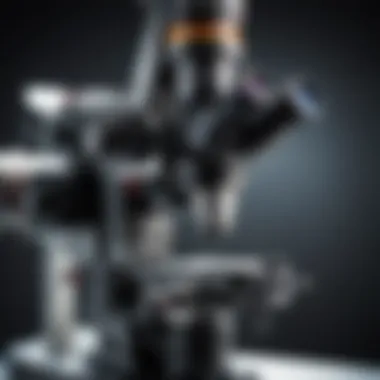
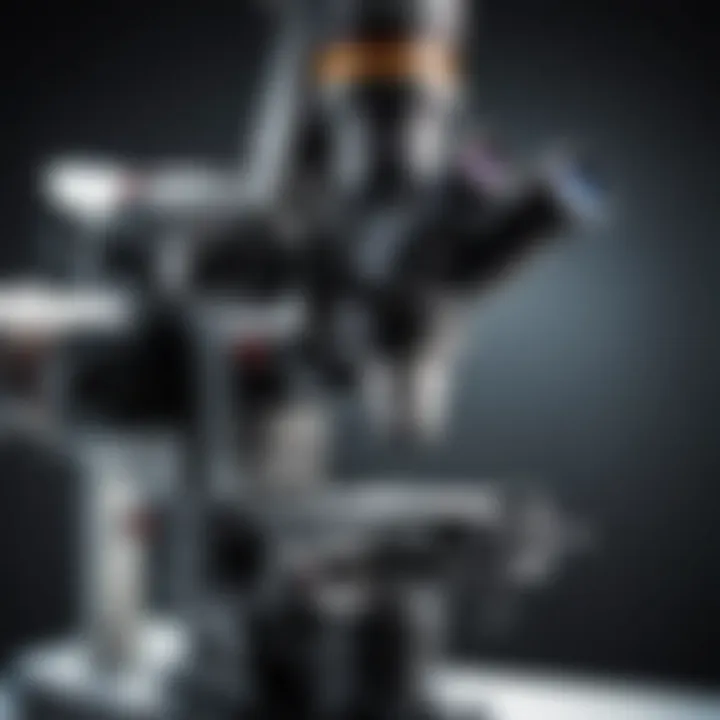
Intro
Microscopy has transformed the landscape of scientific research, allowing for exploration at a scale imperceptible to the naked eye. This article delves into the most powerful microscopes, examining their capabilities and the technology that propels them. Understanding how these tools apply in various scientific fields can enhance appreciation for their role in modern science.
Research Highlights
In this section, key findings and implications of advanced microscopy will be discussed, highlighting how these instruments shape current research methodologies.
Overview of Key Findings
Microscopy has evolved dramatically. Traditional optical microscopes have been significantly surpassed by techniques like electron and atomic force microscopy. These advancements allow researchers to observe samples at the atomic and molecular levels. The key findings of the research indicate that:
- Electron microscopes can achieve resolutions down to a few picometers. This opens new doors to materials science and biology.
- Super-resolution microscopy techniques have revolutionized cellular imaging, making it possible to see structures that were previously undetectable.
- The development of cryo-electron microscopy is enabling the visualization of biological samples at cryogenic temperatures, preserving their native state.
Significance of the Research
The implications of these findings are profound. Enhanced imaging capabilities lead to new discoveries in fields such as nanotechnology, biochemistry, and medical research. For instance, understanding virus structures better can inform vaccine development strategies. Moreover, the research highlights the interplay between various microscopy methods, suggesting that a multi-faceted approach often yields the best insights.
Original Research Articles
This section summarizes relevant original research articles that discuss significant developments in the field of microscopy.
Summary of the Article
Recent studies published in journals such as Nature and Science illustrate breakthroughs in imaging technology and their practical applications. For example, a study on the operation of scanning tunneling microscopy illustrates how it can be utilized to manipulate quantum states, promising advancements in quantum computing.
Author Contributions
Many experts in microscopy have contributed to advancing these technologies. Notable researchers like Eric Betzig and Stefan W. Hell have played key roles in developing super-resolution microscopy techniques. Their collaborative efforts and innovation have a profound impact on how scientists explore the micro-world, enabling finer resolutions and clearer imaging.
"The progress in microscopy reflects broader technological advances, impacting not just science, but also other fields, fostering interdisciplinary collaboration."
The comprehensive exploration of powerful microscopes provided here underscores the necessity of continued advancements in this field as researchers push the boundaries of knowledge.
Understanding Microscopy
Microscopy is a fundamental aspect of scientific inquiry, allowing researchers to examine samples on a scale that is not visible to the naked eye. Understanding microscopy is essential for grasping the advancements in various fields, including biology, materials science, and nanotechnology. The precision and detail provided by microscopes enable scientists to explore structures and phenomena that would otherwise remain hidden. In this article, we will delve into the definition and historical development of microscopy, laying a foundation for understanding its significance in modern research and development.
Definition of Microscopy
Microscopy refers to the use of instruments called microscopes to observe objects that are too small to be seen with the unaided eye. These objects can range from cells to nanostructures. Microscopes function by magnifying the image of the sample, allowing for detailed analysis of its structure, composition, and behavior. There are several types of microscopy techniques, each designed to address specific scientific inquiries. The core goals of microscopy include enhanced clarity and resolution of images, which are vital for accurate interpretation of the biological or physical processes being studied.
Historical Development
The history of microscopy traces back to the late 16th century when the first glass lenses were developed, paving the way for the initial microscope designs. Early microscopes were rudimentary, but they laid the groundwork for future advancements. In the 17th century, Antonie van Leeuwenhoek made significant contributions by improving the design and function of microscopes, enabling the observation of microorganisms.
The development of optical microscopes continued through the centuries with contributions from notable scientists. The 19th century saw the introduction of achromatic lenses, reducing optical aberrations and enhancing image quality. With the 20th century came electron microscopy, introducing high-resolution techniques that allow visualization beyond the limits of light microscopy.
Today, microscopy has evolved to include a multitude of techniques, such as fluorescence microscopy and scanning probe microscopy, representing both technological advancements and the growing needs of scientific exploration. Understanding this historical context enriches one's appreciation of modern microscopy and its capabilities.
Types of Microscopes
Understanding the types of microscopes is essential in the field of microscopy. Each type serves a distinct purpose, offering unique advantages and limitations depending on the specific requirements of the study. Recognizing these differences helps researchers select the appropriate microscope for their work, ultimately influencing results and their significance in various scientific fields.
Optical Microscopes
Optical microscopes are one of the most common types used in laboratories, especially within biology and education. These instruments utilize visible light and optical lenses to magnify samples, allowing for direct observation of specimens. Typical applications include viewing cellular structures and histological samples.
The benefits of optical microscopes include their relatively low cost and ease of use. These microscopes do not require extensive sample preparation compared to other types, making them accessible for many educational institutions. However, they do have limitations, primarily in terms of resolution. The maximum resolution is limited by the wavelength of visible light, which restricts the ability to visualize smaller structures.
Some key considerations when using optical microscopes include:
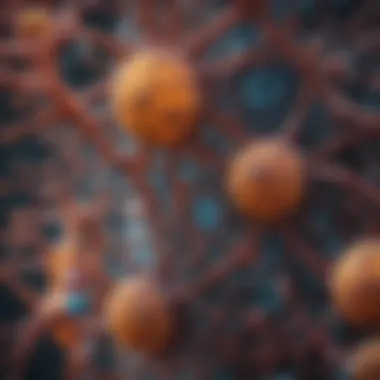
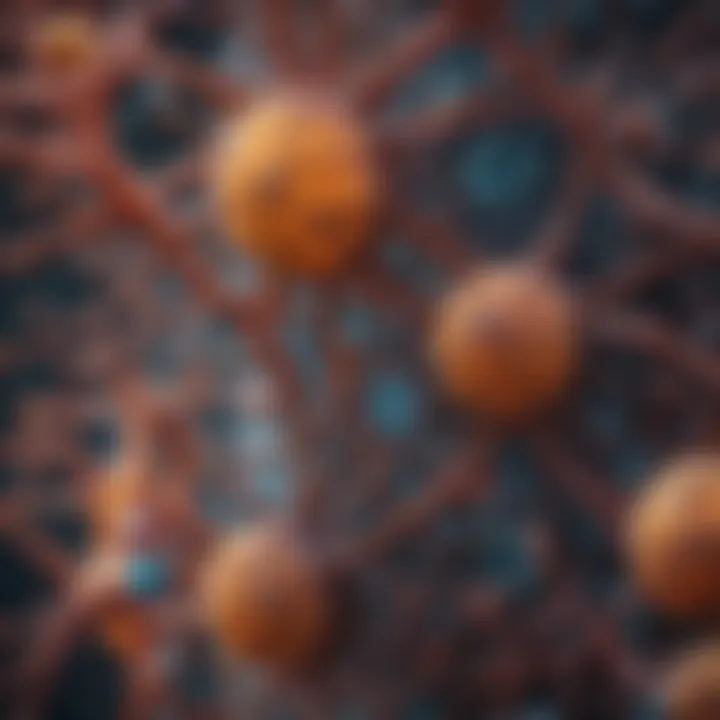
- Lighting conditions: Proper illumination is crucial for achieving the best image quality.
- Objective lenses: Different lenses allow for varying degrees of magnification.
- Slide preparation: Ensuring slides are prepared accurately can enhance observation quality.
Electron Microscopes
Electron microscopes are powerful tools for imaging at the nanoscale. They utilize beams of electrons instead of light to achieve much higher resolutions. Transmission Electron Microscopes (TEM) and Scanning Electron Microscopes (SEM) are two main types of electron microscopes, each suited for specific applications.
TEM allows for the observation of ultra-thin samples, providing detailed internal structures of cells and materials. On the other hand, SEM is used primarily for surface imaging, giving detailed three-dimensional views. This capability is particularly important in materials science and nanotechnology.
The key advantages of electron microscopes include:
- High resolution: Capable of visualizing structures at atomic or molecular levels.
- Versatility: Applicable in various fields, including biomedical research and engineering.
However, challenges such as the need for vacuum environments and elaborate sample preparation can make electron microscopy more complex and costly.
Scanning Probe Microscopes
Scanning probe microscopes (SPMs) represent a unique approach to microscopy. They function by scanning a probe over a sample to gather data on its surface characteristics at atomic resolution. The most common form, the Atomic Force Microscope (AFM), offers precise height measurements and surface topography.
SPMs have become increasingly significant in nanotechnology and materials science because they can examine samples without special treatment. Significant benefits of SPMs include:
- Non-destructive testing: Useful for delicate samples that may be damaged by other techniques.
- Detailed surface information: Capability to analyze physical, chemical, and mechanical properties at very small scales.
On the downside, SPMs can be slower than optical and electron microscopes. Furthermore, the technique requires operator skill and training to achieve optimal results.
Fluorescence Microscopes
Fluorescence microscopes utilize fluorophores for imaging specimens, making them invaluable in cellular and molecular biology. By illuminating the sample with specific wavelengths of light, these microscopes can excite fluorescent molecules, producing bright images that highlight specific components within cells.
The key applications of fluorescence microscopy include:
- Cell tracking: Monitoring the movement of specific biomarkers within living cells.
- Protein localization: Understanding the distribution of proteins in cellular functions.
Some important considerations for using fluorescence microscopes include:
- Choice of fluorophores: Selecting the right dyes is essential for effective visualization.
- Photobleaching: Prolonged exposure to light can degrade the fluorescent signals, affecting results.
In summary, selecting the right type of microscope depends on the details of the research question at hand. Each type of microscope offers its strengths and weaknesses, inherently shaping the research outcomes.
Criteria for Power in Microscopy
Understanding the criteria that define the power of a microscope is crucial for evaluating its effectiveness in scientific inquiry. In this section, we will explore two primary aspects: resolution versus magnification and depth of field. Evaluating these criteria helps clarify how microscopes function and their suitability for specific applications.
Resolution vs. Magnification
Resolution refers to the smallest distance between two points that can be distinctly identified as separate entities. It is a critical characteristic that determines the detail that a microscope can resolve. Higher resolution provides finer details in the observed specimen, enabling better analysis and understanding of structure at micro levels.
Magnification, on the other hand, indicates how much larger the microscope makes the object compared to its actual size. Although higher magnification can make objects appear bigger, it does not necessarily lead to better visibility of fine details. A common misconception is that increased magnification alone signifies superior performance. In reality, it's the relationship between resolution and magnification that defines clarity and detail.
For instance, a microscope that magnifies an image by 2000 times but only resolves details down to 100 nanometers may not be as effective as another that magnifies the same image only 1000 times but can resolve details down to 50 nanometers. The effectiveness of a microscope is contingent upon both its optical design and the wavelength of the light or electrons used for imaging.
"The true power of a microscope lies in its ability to resolve details, not just enlarge images."
Depth of Field
Depth of field pertains to the thickness of the specimen that can be in focus at one time. Microscopes with a greater depth of field can maintain clarity over a thicker slice of the specimen. This is an essential factor when examining three-dimensional structures or uneven surfaces, as it allows researchers to maintain focus on multiple layers or components without constantly readjusting the focus.
A larger depth of field can lead to clearer images for certain applications, like biological specimens, where thickness can vary significantly. That being said, achieving high depth of field might compromise the resolution, as it can lead to a loss of detail in the observed area.
In summary, the criteria for power in microscopy—resolution versus magnification and depth of field—are fundamental to evaluating microscopic systems. Both parameters must be considered by scientists and researchers to choose the appropriate microscope that aligns with their investigation needs. Understanding their nuances can significantly enhance the effectiveness of research in various scientific fields.
The Most Powerful Microscopes in Use
The significance of powerful microscopes lies in their ability to reveal structures that are otherwise invisible to the naked eye. In modern science, these instruments have evolved to push the boundaries of resolution and magnification. The discussion around the most advanced types of microscopes focuses on their unique capabilities, applications, and potential impact on various fields.
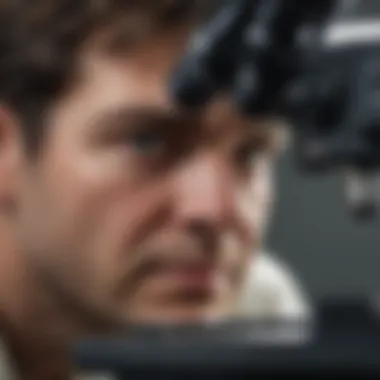
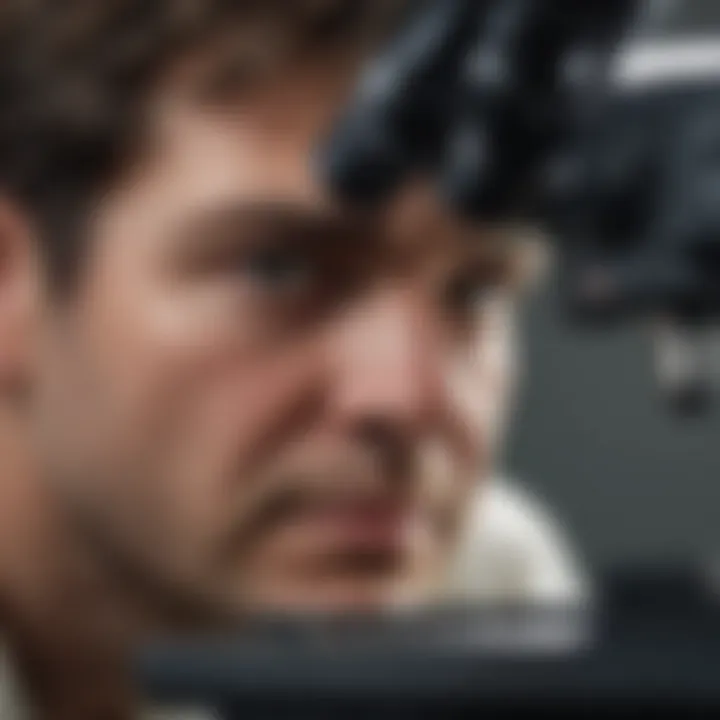
Each microscope mentioned below serves distinct purposes, contributing meaningfully to research in areas like materials science, biology, and nanotechnology. Understanding these differences is essential for researchers when selecting the appropriate instruments for their work.
Transmission Electron Microscope (TEM)
The Transmission Electron Microscope (TEM) is a transformative tool in the world of microscopy. It employs a focused beam of electrons to pass through thin specimens, offering images with high resolution—often at the atomic scale. This technology enables scientists to observe the detailed internal structures of material samples.
The significance of TEM extends into several scientific domains. For example, in materials science, researchers can examine crystalline structures, defects, and the distribution of phase boundaries. Biologists also utilize TEM to uncover cellular structures and organelles, providing insights into cellular function and pathology.
Researchers should note that working with a TEM often requires substantial sample preparation. The specimens must be exceedingly thin, which can complicate the analysis process. Despite this challenge, the unparalleled resolution provided by TEM remains a substantial advantage.
Scanning Electron Microscope (SEM)
The Scanning Electron Microscope (SEM) offers another dimension in the exploration of microscopic structures. SEM generates three-dimensional images by scanning a focused electron beam across the surface of a specimen. The interaction of electrons with the specimen produces signals that provide detailed information about surface morphology and composition.
One of the main advantages of SEM is its ability to analyze a broader range of sample types compared to TEM, including bulk materials. SEM is widely used in fields ranging from biological sciences to engineering materials. For example, while examining biological specimens, SEM helps visualize the fine structures of cells, tissues, and microorganisms in their natural positions.
However, it is important for users to understand that SEM operates in a vacuum, which can alter the characteristics of certain materials, especially biological samples. Thus, sample preparation remains a key consideration while using the SEM technology.
Atomic Force Microscope (AFM)
The Atomic Force Microscope (AFM) is designed for nanoscale imaging and measurement. AFM utilizes a cantilever with a sharp tip that scans over the specimen's surface. This contact can generate images with high resolution, which provides insight into surface profiles and mechanical properties at the atomic level.
AFM's versatility is one of its most notable aspects. It can analyze a variety of sample types, including conductive and insulating materials, without needing extensive preparation. This feature makes it invaluable for researchers in nanotechnology and materials science.
Despite its advantages, AFM does present challenges. The scanning speed may be slower than the techniques used in SEM or TEM. Additionally, the data interpretation requires a solid understanding of both the imaging mode and the material characteristics. This complexity should be carefully considered before employing AFM in research.
"Understanding the respective capabilities of these powerful microscopes is crucial for maximizing their utility in scientific research."
Applications of High-Power Microscopy
High-power microscopy serves as an invaluable asset across numerous scientific disciplines. Its ability to unveil intricate details of structures at the micro and nanoscale cannot be understated. Understanding the applications of these powerful microscopes offers insight into how they influence research and practical advancements in various fields.
Biological Research
In biological research, high-power microscopy is instrumental for studying cells, tissues, and microorganisms. Techniques like fluorescence microscopy and electron microscopy allow researchers to visualize cellular processes in real-time. This capability aids in understanding disease mechanisms, drug interactions, and cellular anatomy.
Benefits include:
- Enhanced visualization of cellular components such as organelles and membranes.
- Ability to track biological processes over time, such as protein expression.
- Support for high-resolution imaging to reveal structures often obscured under lower magnifications.
Researchers utilize the Transmission Electron Microscope (TEM) to observe viruses and small cellular structures that would be invisible to traditional optical methods. Such insights are vital for developing vaccines and therapeutic treatments.
Materials Science
Materials science benefits considerably from high-power microscopy tools. The ability to analyze the microstructure of materials leads to advancements in developing new substances or improving existing ones. Scanning Electron Microscopes (SEM) provide detailed views of surface topographies and compositions, essential for understanding material properties.
Considerations in this field include:
- Evaluating crystallography to enhance material performance.
- Investigating failures in materials, which can lead to critical safety improvements.
- Analyzing defects in nanomaterials, which is essential for nanotechnology applications.
Thus, high-power microscopy not only facilitates basic research but also promotes technological advancements in industries such as aerospace, automotive, and electronics.
Nanotechnology
In the field of nanotechnology, high-power microscopy enables researchers to manipulate and understand materials at an atomic or molecular level. Techniques such as Atomic Force Microscopy (AFM) are crucial for measuring surface forces and mapping surface features with exceptional resolution.
Key implications for nanotechnology include:
- Precision in fabricating nanoscale devices, which has applications in electronics and medicine.
- Exploration of phenomena that occur at the nanoscale, shedding light on unique properties of nanomaterials.
- Development of nanoscale imaging techniques that improve detection and analysis of biological markers in medical diagnostics.
With these applications, high-power microscopy not only expands our understanding of material properties but also drives innovations that improve everyday technology and treatment options in healthcare.
In summary, the applications of high-power microscopy are diverse and crucial. They significantly enhance our understanding of complex systems in biology, materials science, and nanotechnology, paving the way for future scientific innovations.
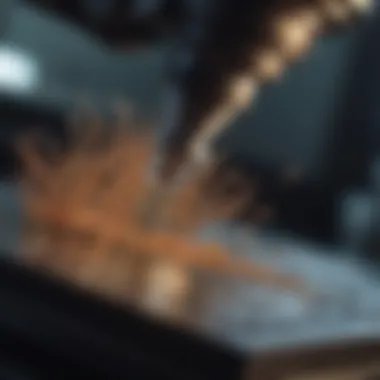
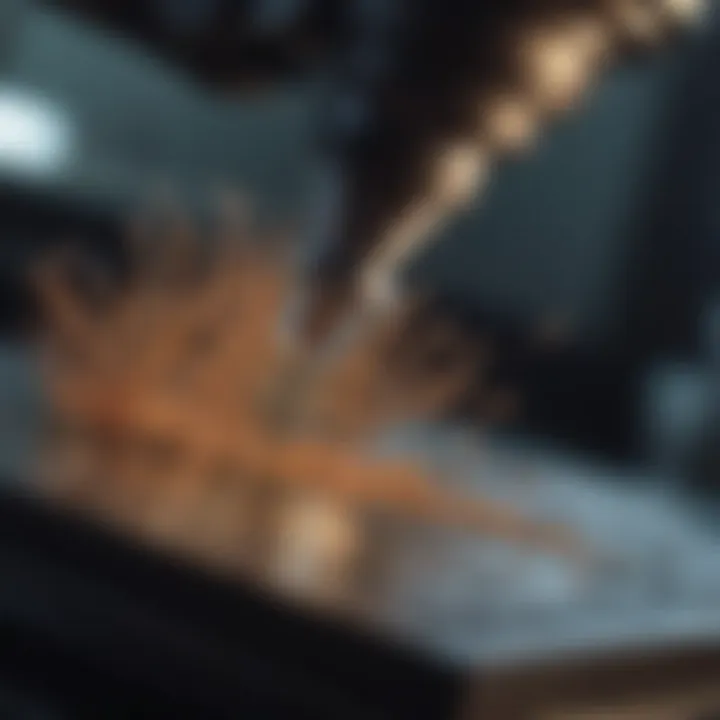
High-power microscopy serves as a bridge between theoretical science and practical application, making it an essential tool in the modern research landscape.
Challenges in Advanced Microscopy
The field of advanced microscopy is marked by significant technological progress, yet it encounters various challenges that can impact its overall effectiveness in research and practical applications. Understanding these challenges is essential for anyone looking to delve deeper into microscopy, as they highlight potential limitations and considerations that may influence study outcomes or commercialization of results. This section elaborates on two prominent issues: sample preparation hurdles and the cost and accessibility of advanced microscopes.
Sample Preparation Issues
Sample preparation plays a crucial role in the successful acquisition of microscopic images. The requirement for high-quality samples is imperative for achieving accurate results. With different microscopy techniques, various preparation methods are needed. For instance, electron microscopy often necessitates that samples be dehydrated and embedded in resin to withstand the high vacuum. This can distort biological specimens and obscure their natural state, leading to misleading interpretations.
In addition, the need for thin sectioning in techniques like Transmission Electron Microscopy (TEM) can be difficult, especially with biological materials that are often soft and fragile. If not done properly, the sample can break or become damaged, affecting the overall quality of the imaging output.
Moreover, certain specimens may require staining or labeling, which introduces another layer of complexity. Stains can impart additional information but may also alter the sample's inherent properties. Therefore, careful balance is needed during preparation. Despite these hurdles, significant advancements in automation and micro-sampling techniques continue to emerge, aiming to address preparation issues more efficiently.
Cost and Accessibility
The cost associated with advanced microscopy is another major challenge in this field. Instruments like the Scanning Electron Microscope (SEM) and Atomic Force Microscope (AFM) represent substantial financial investments. Acquisition costs can range into hundreds of thousands of dollars, not accounting for ongoing maintenance, training, and operational costs. For many institutions, particularly smaller labs or those in developing regions, these expenses can limit access to cutting-edge technology.
In addition, the expertise required to operate these machines can be a barrier. Training personnel to effectively use advanced microscopes demands time and resources, which may not be readily available, particularly in lower-budget environments. Some institutions may also face difficulties in competing for grant funding to support these endeavors.
Moreover, the exclusivity of access can lead to disparities in research opportunities. Institutions with better funding can offer their researchers more access to advanced microscopy technologies, creating an uneven landscape in scientific inquiry.
"Addressing the cost and preparation challenges in microscopy is vital for democratizing access to knowledge and fostering innovation across diverse scientific communities."
In line with these challenges, ongoing efforts need to focus on making advanced microscopy more affordable and accessible while also improving sample preparation methodologies. Such initiatives can help level the playing field, allowing researchers from varied backgrounds to explore the vast potential that modern microscopy offers.
Future Trends in Microscopy
In the evolving landscape of scientific research, understanding future trends in microscopy has become essential. As technology advances, microscopes are undergoing significant transformations. These advancements not only enhance the capabilities of existing microscopes but also pave the way for novel applications in various fields. This section will delve into two primary areas driving innovation in microscopy: advancements in imaging techniques and the integration of artificial intelligence.
Advancements in Imaging Techniques
Recent developments in imaging techniques are redefining microscopy. These advancements are important as they address the limitations of traditional microscopy methods, allowing for higher resolution and faster imaging. One notable technique is super-resolution microscopy, which surpasses the diffraction limit of light. This method permits scientists to observe cellular processes in real-time at unprecedented detail.
Another intriguing trend is the use of 3D imaging techniques. These allow for the visualisation of biological samples in three dimensions, enhancing our understanding of complex structures. Technologies such as light-sheet microscopy and computed tomography have emerged, enabling researchers to study samples non-invasively and with reduced photodamage.
Furthermore, developments in correlative microscopy combine multiple imaging modalities, providing comprehensive insights into sample morphology and function. This holistic approach strengthens data interpretation across different observational contexts. All these advancements contribute significantly to understanding the microscopic world, enhancing research capabilities in numerous scientific disciplines.
Integration with Artificial Intelligence
The integration of artificial intelligence in microscopy is a compelling trend that holds the potential to revolutionize research. AI algorithms can analyze vast amounts of data generated by advanced microscopes more efficiently than any human. This capability is particularly beneficial in fields such as biology and materials science, where identifying patterns can be crucial.
Machine learning techniques can be employed to enhance image reconstruction processes, improve segmentation accuracy, and automate quantification tasks. By reducing the time and effort researchers spend on data analysis, AI allows them to focus on interpretation and discovery.
Moreover, AI can assist in the development of predictive models, enabling researchers to hypothesize outcomes based on microscopic observations. This fusion of technology not only streamlines workflows but also promotes innovative discoveries that would not be possible through traditional methodologies.
"The integration of AI in microscopy transforms how researchers interact with data, painting a more vivid picture of the microscopic realm."
Closure
The exploration of microscopy offers significant insights into the microscopic world and its broader implications in science and industry. As microscopes evolve, their power and versatility shape research practices across various disciplines. Understanding the strengths and limitations of different microscopes is vital for researchers and professionals alike. The conclusion drawn from this article emphasizes the need to appreciate both the fundamental principles of microscopy and the technological advancements that propel the field forward.
Microscopy is not merely an academic subject; it unlocks phenomena that are not visible to the naked eye. This capability enables breakthroughs in biological research, nanotechnology, and materials science. The significance of this knowledge can therefore not be overstated, as it informs practical applications that have the potential to enhance our quality of life.
Summary of Key Points
In summation, there are several pivotal elements to consider:
- The definition and historical context of microscopy is essential for grasping its evolution.
- Various types of microscopes exist, each with unique features and applications.
- Criteria such as resolution and depth of field are fundamental to assessing a microscope's power.
- Advanced microscopes like the Transmission Electron Microscope and Atomic Force Microscope represent the pinnacle of scientific tools.
- Microscopes have transformative applications in critical fields including biology and nanotechnology.
- Challenges such as high costs and sample preparation issues still remain for practitioners.
- Future directions include advancements in imaging and the integration with artificial intelligence.
In considering these points, one can understand the intricate relationship between microscopy and modern scientific inquiry.
Implications for Future Research
The implications for future research are profound. As microscopy technology continues to advance, new methodologies may emerge.
- Increased Accessibility: Efforts to reduce costs could democratize access to high-power microscopes. This will enable smaller institutions and even individual researchers to utilize advanced tools.
- Enhanced Scientific Discovery: With improved imaging capabilities, researchers will uncover details of structures at the atomic level, paving the way for innovations in various fields.
- Interdisciplinary Collaborations: New applications for microscopy can inspire partnerships between disciplines, such as biochemistry and materials science, leading to combined efforts in solving complex challenges.
Overall, a forward-looking perspective on microscopy reveals vast potential. The integration of artificial intelligence and enhanced imaging techniques will likely redefine how researchers investigate materials and biological specimens, unleashing new waves of discovery.