Thermoelectric Refrigeration: Principles and Uses
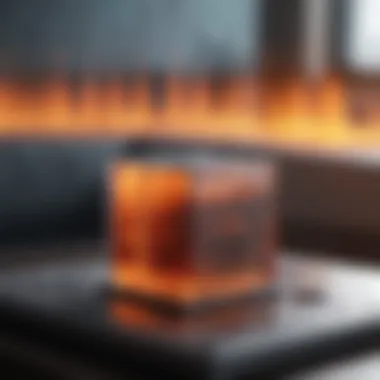
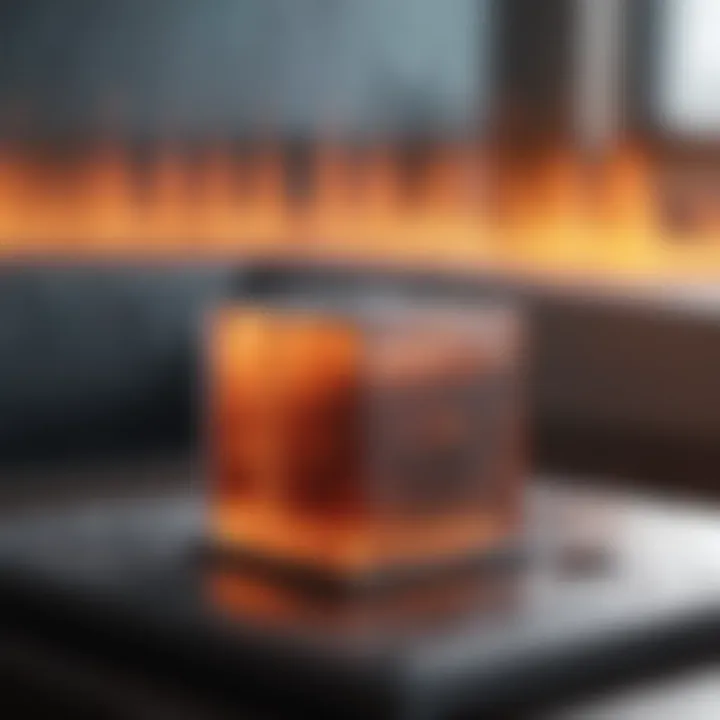
Intro
Thermoelectric refrigeration is a fascinating field of study that has been gaining traction as a practical alternative to conventional cooling methods. The underlying principle lies in the thermoelectric effect—a phenomenon that allows the conversion of temperature differences into electric voltage. This mechanism paves the way for a range of applications, from keeping electronic components cool to offering portable refrigeration solutions that suit modern lifestyles.
As climate concerns continue to grow and energy efficiency becomes paramount, thermoelectric refrigeration offers notable advantages. These systems are compact, environmentally friendly, and often possess a longer lifespan compared to traditional refrigeration systems. However, like any technology, various challenges exist, particularly in terms of material efficiency and cost-effectiveness. This article dives deep into the principles behind thermoelectric refrigeration, shedding light on the materials involved, operational mechanisms, and the vast array of applications.
Understanding the intricacies of thermoelectric devices is essential for researchers and industry professionals alike. By exploring both the advantages and limitations, we seek to illuminate this technology's potential and future trends.
Let's embark on this journey into the world of thermoelectric refrigeration and explore its significance in today's cooling technologies.
Prelims to Thermoelectric Refrigeration
Thermoelectric refrigeration stands at the intersection of science and practical application. This technology has gained traction in various fields where efficient cooling is paramount. Unlike traditional cooling technologies that rely on refrigerants and mechanical compressors, thermoelectric systems offer a unique method for heat management by utilizing solid-state devices. The simplicity of their design, alongside their potential for portability and miniaturization, highlights their relevance to a fast-evolving technological landscape.
In a world increasingly aware of environmental sustainability, thermoelectric refrigeration presents an enticing alternative. Devices built on thermoelectric principles eliminate the need for harmful refrigerants, thereby reducing greenhouse gas emissions. As we unpack the specifics in this article, understanding how these systems operate, their benefits, and limitations will lead us toward recognizing their role in future applications.
Definition and Overview
Thermoelectric refrigeration involves the use of thermoelectric devices to transfer heat from one side of the device to another, effectively cooling one side while warming the other. It operates on principles rooted in the thermoelectric effects, particularly the Peltier effect, which is the basis of its functionality. When an electric current passes through a junction of two different conductors, a temperature differential is created; one junction absorbs heat, while the other dissipates it.
This mechanism allows for precise temperature control, making thermoelectric refrigeration suitable for various applications like electronics cooling, refrigeration units, and portable coolers. Moreover, the complexity of the devices can range from simple modules used in small-scale applications to advanced systems designed for high-performance cooling under specific conditions. Importantly, these systems can be integrated into a wider range of technology, from household appliances to medical equipment.
Historical Context
The journey of thermoelectric refrigeration can be traced back to the early 19th century with the discovery of the thermoelectric effects. In 1821, Thomas Johann Seebeck discovered the Seebeck effect, which would later lay the groundwork for thermoelectric technology. Further advancements came with Jean Charles Athanase Peltier’s discovery in 1834, when he revealed the Peltier effect, showcasing how electric currents could be utilized for cooling.
Over the decades, advances in material science and semiconductor technology have propelled the field forward. Initially, metals were used in thermoelectric devices, but the focus gradually shifted to semiconductors, which offered higher efficiencies. By the late 20th century, with the advent of advanced materials like bismuth telluride, practical applications gained momentum.
Today, thermoelectric refrigeration is not just an academic curiosity; it represents a burgeoning field that continues to evolve, spurred by a growing demand for efficient cooling technologies that are upwards of mechanical systems. This ongoing evolution signifies an important shift in how we approach thermal management in a technology-driven world.
Fundamentals of Thermoelectricity
Understanding the fundamentals of thermoelectricity is essential for grasping how thermoelectric refrigeration works. At the core, the fundamentals explore how materials can convert temperature differences into electric voltage, and vice versa. This principle opens up a range of practical applications that not only benefit technology but also have implications for energy efficiency and environmental impact.
The Seebeck Effect
The Seebeck effect is a fascinating phenomenon where a temperature difference across a conductor leads to the generation of an electric voltage. When one end of a conductive material is heated while the other remains cool, electrons move from the hot side to the cooler side, creating an electric current. This can be observed in thermoelectric devices, making them capable of harnessing waste heat effectively.
To put it simply, if you have a metal rod with one end placed in hot water and the other end in ice, the temperature gradient triggers electron movement, producing electricity. This unique capability opens doors to innovative solutions in energy recovery systems.
"The Seebeck effect demonstrates a straightforward yet impactful way to transform thermal energy into electrical energy, paving the road for numerous applications in computational devices."
The Peltier Effect
Contrasting the Seebeck effect is the Peltier effect, where applying an electric current through two different conducting materials causes heat to move. Depending on the direction of the current, one junction cools down while the other heats up. This effect is pivotal in thermoelectric refrigeration, as it allows for the creation of cooling systems without mechanical components.
For instance, if you connect a thermoelectric module to a power source, one side of the module will absorb heat from its environment, providing a cooling effect, while the opposite side dissipates this heat. This principle is utilized in portable coolers and compact refrigeration units, showcasing the versatility of thermoelectric devices.
The Thomson Effect
Completing this triad is the Thomson effect, which describes how a current passing through a conductor with a temperature gradient can either absorb or emit heat. Essentially, this effect allows for the modification of thermal conditions within materials during electrical conduction. It might not find as direct an application as the Seebeck or Peltier effects, but it still plays a vital role in understanding and refining thermoelectric materials and devices.
As you delve deeper into thermoelectricity, it becomes evident that these foundational effects are key in harnessing thermal energy and transforming it into a useful form. The interplay between these concepts not only informs engineering but also drives innovation in various industries, emphasizing their significance in modern applications and future developments.
Composition and Structure of Thermoelectric Devices
The composition and structure of thermoelectric devices are integral to their functionality and effectiveness in refrigeration applications. Understanding how these devices are put together allows us to appreciate not just their operational capabilities but also their limitations and potential advances in technology. Thermoelectric devices harness the thermoelectric effect to transfer heat from one side to the other, enabling cooling. This process is highly dependent on the materials used and the configuration of the device, both of which play a vital role in performance characteristics such as efficiency and longevity.
Materials Used in Thermoelectric Refrigerators
When it comes to the materials utilized in thermoelectric refrigerators, the selection is paramount. The efficiency of thermoelectric devices hinges on the thermoelectric properties of the materials, specifically their Seebeck coefficient, electrical conductivity, and thermal conductivity. Here are some key materials traditionally used:
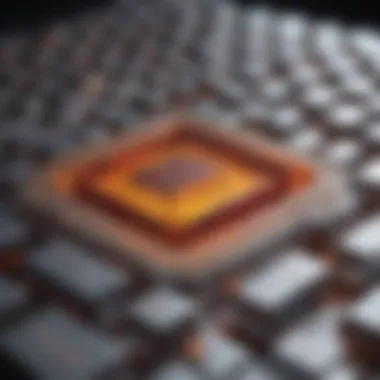
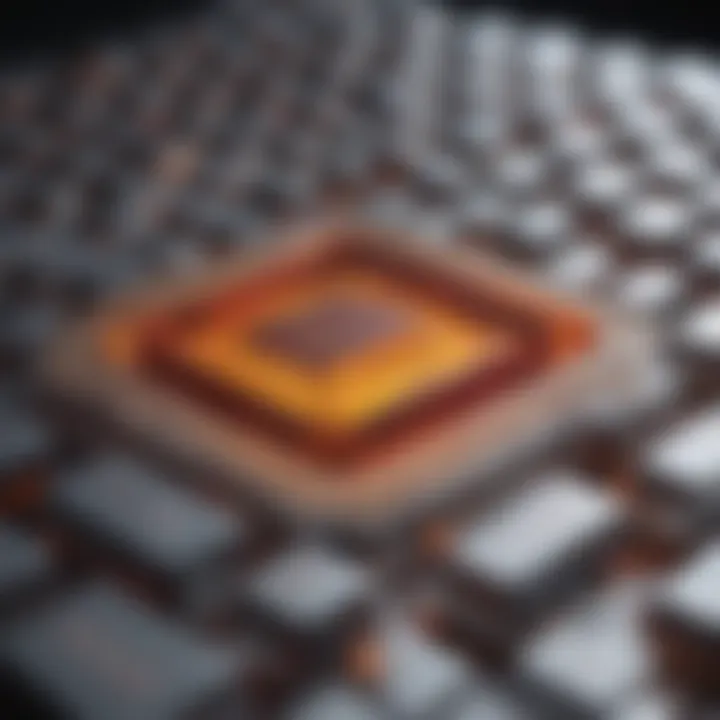
- Bismuth Telluride (Bi2Te3): Frequently employed in near-room temperature applications, this material showcases an excellent balance between performance and cost.
- Lead Telluride (PbTe): Better suited for higher temperature ranges, lead telluride maintains favorable thermoelectric performance at elevated temperatures.
- Silicon-Germanium Alloys: These are generally used in high-temperature applications, such as space missions, due to their robustness and high performance in extreme conditions.
- Skutterudites: This category has been gaining attention for their impressive thermoelectric efficiency due to their unique crystal structure that facilitates low thermal conductivity while maintaining good electrical properties.
Choosing the right material is crucial not just for fitting the desired application but also for ensuring longevity and effectiveness in various operational conditions. A thorough understanding of the properties of these materials can lead to significant innovations in thermoelectric refrigeration technology.
Thermoelectric Module Configuration
The configuration of a thermoelectric module is another critical factor influencing its performance. Generally, a thermoelectric module consists of several thermoelectric elements that are arranged in a specific manner to achieve optimal heat transfer and cooling efficiency. The two most common arrangements are:
- Series Configuration: In this setup, thermoelectric elements are connected end to end. This facilitates a consistent flow of charge carriers, but it can lead to a bottleneck effect if one element underperforms.
- Parallel Configuration: Here, elements are connected side by side, which allows for a more even distribution of current. This configuration can be advantageous in applications requiring uniform cooling across a surface.
The choice between these configurations depends largely on the specific application and the desired cooling capacity. Moreover, the physical arrangement can have a notable impact on thermal management within the device. Alignments that minimize thermal bridges and enhance heat flow will ultimately improve overall performance.
"The advancement in materials science and configuration design stands as the cornerstone of evolving thermoelectric refrigeration systems, making them increasingly viable for broad applications."
By comprehending the composition and structure of thermoelectric devices, stakeholders can not only enhance existing technologies but also pioneer new applications and solutions across various domains. This understanding lays a foundational stone upon which future innovation can be built.
Operational Mechanism of Thermoelectric Refrigeration
Understanding the operational mechanism of thermoelectric refrigeration is crucial for grasping how these systems harness electricity to achieve cooling effects. At the heart of this technology lies intricate interactions between heat flow and the unique properties of thermoelectric materials. The knowledge of these mechanics not only illuminates the fundamental principles involved but also reveals their applicability in various fields. Through examining the dynamics of heat transfer and energy conversion efficiency, one gains insights into the potential benefits and limitations of these refrigeration solutions.
Heat Flow Dynamics
In thermoelectric refrigeration, heat flow dynamics are governed by the Peltier and Seebeck effects. When a thermoelectric device is powered, it creates a temperature differential between its two sides. This thermal gradient causes heat to move from the hot side to the cold side. As a result, heat absorption occurs on one side, providing the desired cooling effect.
Key Factors Influencing Heat Flow Dynamics:
- Material Properties: The effectiveness of heat transfer is heavily reliant on the characteristics of the materials used in the thermoelectric devices. High thermal conductivity is essential, as it facilitates optimal heat transfer while maintaining low electrical conductivity to minimize energy losses.
- Temperature Difference: A substantial temperature difference between the two sides amplifies the cooling effect, creating a more efficient cooling cycle. Small temperature gradients may hinder the performance.
- Geometry and Design: The configuration of thermoelectric modules also plays a critical role. A well-designed system encourages better heat flow and enhances overall efficiency.
In more practical terms, when a voltage is applied to a thermoelectric module, electrons migrate from the hot side to the cold side, which pulls heat away from the cold side and dissipates it on the hot side. This process not only exemplifies the interplay of physics and materials science but also serves several applications across industries, facilitating everything from smaller consumer electronics to temperature control in automotive environments.
"Understanding heat flow dynamics is like navigating a river; knowing where the currents go makes all the difference in getting from point A to B efficiently."
Energy Conversion Efficiency
Energy conversion efficiency is another cornerstone of thermoelectric refrigeration systems. Simply put, this refers to how effectively the device converts electrical energy into a cooling effect. High efficiency is paramount for the viability of any cooling technology, especially in times where energy consumption considerations are more pressing than ever.
Several factors impact energy conversion efficiency:
- Quality of Material: Advanced materials like bismuth telluride and lead telluride exhibit superior thermoelectric properties, thereby enhancing efficiency levels.
- Operating Temperature: Efficiency is also influenced by operating conditions. Devices performance varies widely depending on ambient temperatures, and optimizing this can yield better results.
- Cooling Load: The requirements of the system, such as the amount of heat it needs to manage, play a crucial role in determining how efficiently it operates.
The energy conversion efficiency can be quantified by the dimensionless figure of merit (ZT), which serves as a critical benchmark in comparing different materials and configurations. In practical scenarios, achieving high efficiency isn't just about understanding materials but also about integrating system designs that allow for optimal energy use.
In summary, grasping the operational mechanisms of thermoelectric refrigeration—including heat flow dynamics and energy conversion efficiency—provides a roadmap for advancing technologies that champion energy conservation and efficient cooling capabilities. As innovations continue to emerge in this area, the benefits are set to evolve even further.
Advantages of Thermoelectric Refrigeration
When discussing thermoelectric refrigeration, it’s impossible to overlook the advantages this technology brings to the table. By highlighting these benefits, we can truly appreciate why thermoelectric systems are making waves in various industries. Understanding their strengths can lead to more informed decisions regarding their implementation and encourage further exploration in the field. Here are the main advantages:
Compactness and Portability
One standout feature of thermoelectric refrigeration is compactness. Traditional refrigeration setups can often be bulky and heavy, posing challenges in space-constrained environments. In contrast, thermoelectric modules are lightweight and may easily fit into tight spots that larger units can’t. A good example can be seen in portable coolers used for camping or outdoor activities. These coolers can incorporate thermoelectric elements that maintain temperatures without taking up as much space as conventional cooling methods.
The portability aspect extends beyond consumer goods. For example, in medical applications, having a small yet effective refrigeration solution is crucial for transporting temperature-sensitive medications. This is where thermoelectric refrigeration shines, providing a reliable option without the cumbersome weight of traditional cooling systems.
Eco-Friendliness
Today's world demands a shift towards more sustainable technologies, and thermoelectric refrigeration falls neatly within that framework. Unlike conventional systems that typically rely on harmful refrigerants, thermoelectric systems use solid-state technology, which is more environmentally friendly. The absence of moving parts leads to reduced waste and less energy consumption, thus lowering the overall carbon footprint associated with cooling solutions.
The operation of thermoelectric devices does not emit greenhouse gases, making it a preferable choice for eco-aware businesses. We often hear “think globally, act locally,” and with thermoelectric refrigeration, you can do just that. Businesses can adopt this technology to align with sustainability goals while still enjoying efficient cooling performance.
Low Maintenance Requirements
Another appealing aspect of thermoelectric refrigeration is the minimal maintenance it requires. Traditional refrigeration systems often necessitate routine checks, including refrigerant replenishment and inspection of mechanical components. Thermoelectric systems, on the other hand, have fewer mechanical parts, leading to less wear and tear over time.
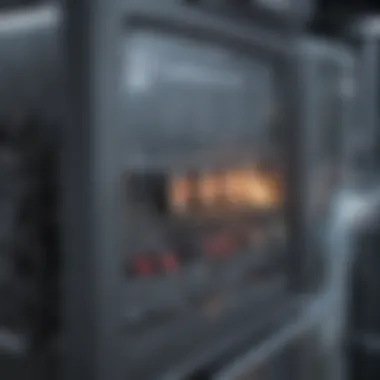
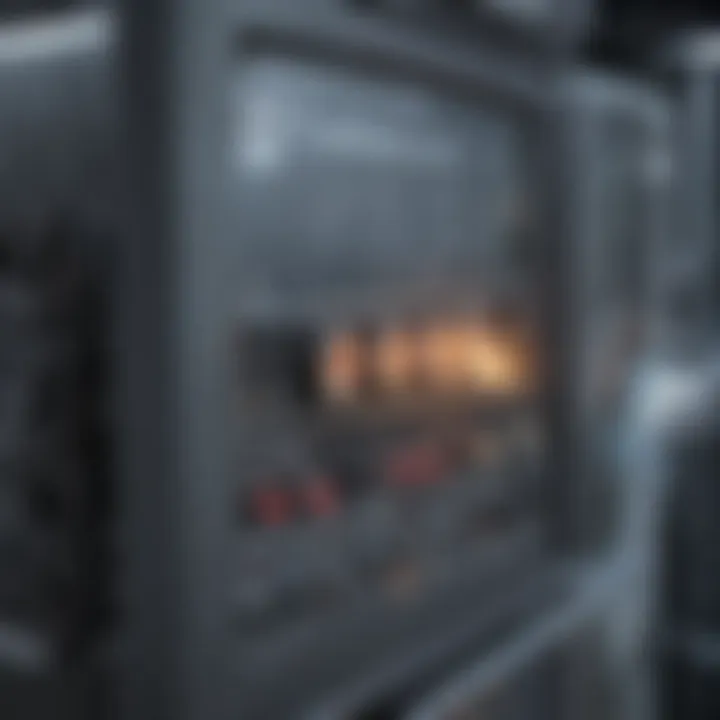
This translates to lower costs and less hassle for end users. For industries that depend on continuous operation, like pharmaceuticals and food preservation, the reliability and reduced downtime are vital benefits. The simplicity of thermoelectric units allows them to be run without extensive operator training, thereby ensuring that maintenance can be straightforward and easily handled.
"With fewer parts to break down and easier operational protocols, thermoelectric refrigeration stands out as a practical choice in various fields."
In summary, the advantages of thermoelectric refrigeration—compactness, eco-friendliness, and low maintenance—make it a promising choice for many applications today. As more industries seek to optimize their cooling solutions while being mindful of environmental impacts, this technology is set to gain even more traction.
Limitations of Thermoelectric Refrigeration
Thermoelectric refrigeration holds promise as an alternative to traditional cooling methods, but it’s crucial to address its limitations. Understanding these constraints isn’t merely academic; it could shape decisions in research, design, and application. Insights into these limitations prompt a more informed approach to adopting thermoelectric technology, keeping in mind both its potential and drawbacks.
Cost Considerations
Cost is often a dealbreaker when it comes to widespread adoption of any technology. Thermoelectric refrigeration, unfortunately, isn’t the most budget-friendly option on the market. The materials required to achieve efficient thermoelectric effects tend to be expensive. Bismuth telluride, a well-known thermoelectric material, is notably costly, and sourcing it can also be a bear due to limitations in availability.
The initial setup for thermoelectric cooling systems usually demands a higher investment than standard vapor-compression systems. This can make it a tough sell, particularly in settings where cost competitiveness is a priority.
Moreover, production processes are not yet optimized for mass manufacturing. This means that economies of scale haven’t yet kicked in for thermoelectric systems. Consequently, in applications where budget is a primary concern, decision-makers may be hesitant to adopt this technology—even if the long-term savings on maintenance and energy use could make it worth considering.
Performance Challenges
Performance hurdles are another thorn in the side of thermoelectric refrigeration. While these systems can be quite efficient at cooling, they often fall short when it comes to handling large heat loads. The efficiency, measured by the coefficient of performance (COP), can drop significantly with increasing temperature differences between the hot and cold sides of the system.
One major issue is thermal management. The heat generated on the hot side needs to be dissipated effectively; otherwise, it can lead to overheating, impacting the overall performance of the unit. Unlike conventional systems that excel in larger environments, thermoelectric units are more sensitive to their operational environment. For example, if not properly ventilated, a thermoelectric cooler can struggle to maintain its cooling capacity.
Lastly, the temperature differential that can be achieved with current materials is often limited. This can lead to scenarios where thermoelectric refrigeration isn’t suitable for applications that require significant cooling below ambient temperatures.
"Understanding the limitations of thermoelectric refrigeration is as crucial as recognizing its advantages; only then can one make rational and informed decisions regarding its use in practical applications."
In summary, while thermoelectric refrigeration shows great potential, stakeholders must carefully weigh its costs and performance challenges against their unique needs. Recognizing these limitations allows for a more balanced view of what thermoelectric technology can achieve in the future.
Applications of Thermoelectric Refrigeration
Thermoelectric refrigeration, an innovative cooling method, is carving out a niche across various sectors. The importance of this topic lies in its potential for energy efficiency, compact design, and eco-friendliness compared to traditional cooling systems. As we delve into specific applications, it's crucial to recognize how the unique characteristics of thermoelectric modules respond to diverse cooling demands.
Consumer Electronics
In the realm of consumer electronics, thermoelectric refrigeration is gaining traction. Devices like portable coolers, mini refrigerators, and wine coolers benefit from this technology due to its compactness.
- Size: Unlike conventional systems, thermoelectric units can be designed small enough to fit into a variety of electronic devices. Think of how your favorite gadget might keep your drink cold without taking up too much space.
- Silent Operation: These cooling systems operate quietly, a significant benefit for devices requiring minimal noise, such as gaming consoles and audio equipment.
As an added bonus, the ability to operate in reverse (heating in winter) opens further applications. Consumers are increasingly attracted to products that combine functionality with sleek design, and thermoelectric modules fit that bill.
"The small footprint and efficiency of thermoelectric cooling make it invaluable for the modern tech landscape."
Automotive Applications
Automotive applications of thermoelectric refrigeration are also on the rise. From seat cooling systems to battery temperature regulation, the automotive industry is exploring the benefits of this technology.
- Seat Cooling: Imagine a car on a hot day where you can keep your seats at a comfortable temperature. Thermoelectric modules ensure a pleasant driving experience without needing extensive modifications.
- Battery Management: The performance of electric vehicles depends heavily on battery temperature. Thermoelectric units can provide targeted cooling to maintain optimal operating conditions, thus enhancing battery life and efficiency.
Automakers looking to modernize their fleets with eco-friendly features are finding thermoelectric modules an attractive choice. The minimal moving parts mean these systems require less maintenance compared to traditional cooling methods.
Medical Refrigeration
In the medical field, precision cooling is paramount. Thermoelectric refrigeration is particularly suited for applications like laboratory equipment, blood storage, and pharmaceutical refrigeration.
- Stable Temperatures: Maintaining a consistent, cold temperature is critical for the preservation of vaccines and sensitive samples. Thermoelectric systems can achieve this without the fluctuations common in conventional units.
- Portability: Devices designed for on-site testing or fieldwork benefit from the lightweight nature of thermoelectric modules. This mobility is vital for emergency medical services and remote locations.
Hence, the advantages of thermoelectric refrigeration are not merely theoretical; they manifest in practical applications that serve crucial societal needs. As the technology develops, expect further innovations that harness these principles, addressing ever-changing market demands.
Comparative Analysis with Conventional Cooling Systems
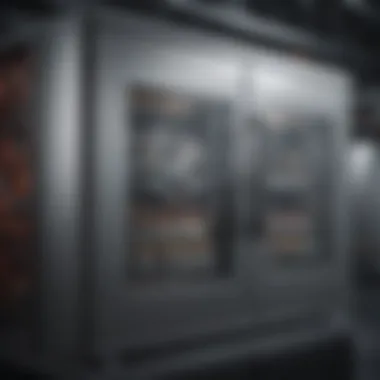
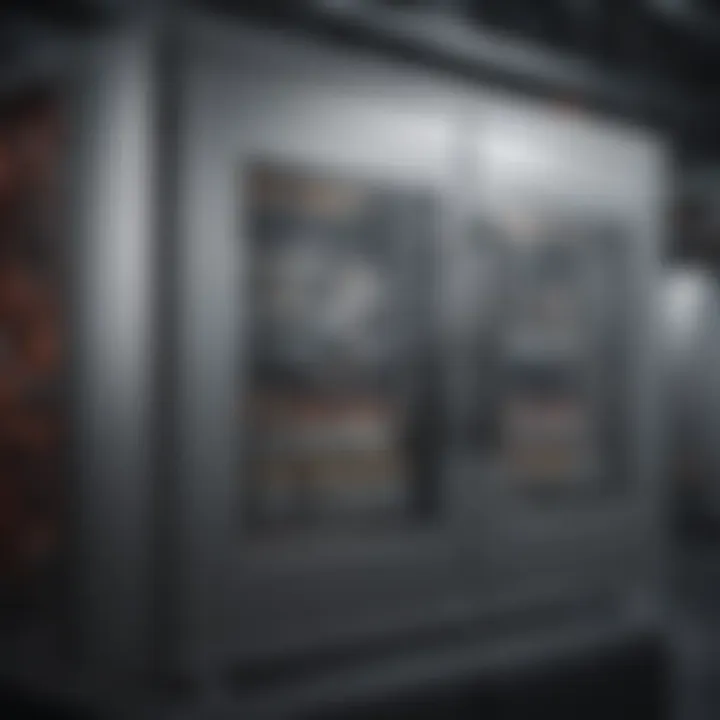
Thermoelectric refrigeration is a unique technology that poses a significant challenge to traditional cooling systems. Understanding this comparative analysis is crucial for anyone involved in the field of refrigeration and cooling, as it highlights key differences and potential advantages of employing thermoelectric mechanisms over conventional units, such as vapor-compression systems. This section will touch upon efficiency benchmarks and delve into environmental impacts that are paramount in today’s eco-conscious landscape.
Efficiency Comparison
When discussing efficiency, it's essential to establish a clear comparison between thermoelectric refrigeration and traditional methods. Conventional systems typically rely on a series of mechanical compressions and expansions to produce cooling effects. This process can be energy-intensive and less efficient, particularly in smaller applications.
In contrast, thermoelectric systems employ solid-state devices to transfer heat effectively. They harness the Peltier effect, which provides direct refrigeration without needing moving parts. This mechanism can notably lower energy consumption in many cases.
- Thermal Efficiency: Thermoelectric units often offer different cooling efficiencies, primarily because they can be more easily tailored for specific applications. For instance, small medical devices require precise temperature control, where thermoelectric systems may outperform traditional methods.
- Energy Use: In scenarios where low power consumption is desirable, thermoelectric units also stand out. For a battery-operated cooler, for instance, their ability to provide cooling at lower power consumption can make them favorable.
- Waste Heat: Another aspect where thermoelectric systems differentiate is through waste heat management. They generally produce less waste heat, making heat recovery systems more efficient and feasible.
On the flip side, it must be acknowledged that the maximum cooling power of thermoelectric devices currently does not match that of conventional cooling systems. The peak performance of traditional units in larger applications remains a tough contender.
Environmental Impact Assessment
Environmental considerations play a pivotal role in the ongoing transition to renewable technologies. Traditional refrigeration approaches often use hydrofluorocarbons (HFCs), which have a notorious reputation for contributing to global warming, in addition to requiring substantial energy inputs.
Thermoelectric refrigeration introduces a potentially better alternative. Here’s a detailed look into the various aspects:
- Refrigerants: Thermoelectric systems typically operate without harmful refrigerants, making them a more environmentally benign option. This is particularly advantageous in applying stringent upcoming regulations around refrigerants worldwide.
- Sustainability: The solid-state nature of thermoelectric modules results in lower material degradation. They are often more durable and can last longer than their vapor-compression counterparts, reducing waste during manufacturing.
- Integration with Green Energy: Furthermore, thermoelectric devices can easily be integrated with renewable energy sources, promoting a more sustainable future. For example, pairing them with solar panels for off-grid applications offers independent cooling solutions without fossil fuel reliance.
By comparing thermoelectric refrigeration with traditional cooling systems, it is clear that while each has its pros and cons, the niche applications and potential environmental benefits of thermoelectric systems give them an edge in certain contexts, especially as technology progresses.
In summary, while traditional systems hold ground in specific high-demand applications, thermoelectric refrigeration opens doors to opportunities aligned with contemporary energy efficiency and environmental sustainability goals. By continuing to innovate and expand thermoelectric technologies, significant strides can be made in reducing the impact of cooling on the planet.
Future Trends in Thermoelectric Refrigeration
In the ever-evolving landscape of technology, thermoelectric refrigeration stands on the brink of significant advancements. As sustainable practices gain traction and global consciousness shifts toward eco-friendliness, the potential future trends in this field warrant a close examination. Understanding these trends is crucial, as they may reshape how we perceive cooling systems, blending efficiency with environmental stewardship. Through enhancing technologies that harness both heat and electricity, thermoelectric systems hold the promise of more effective and versatile applications. As such, a focus on future trends opens the door to opportunities and innovations that could redefine market dynamics.
Advancements in Material Science
The backbone of thermoelectric refrigeration technology lies in the materials used. Current advancements in material science are a hot area of research and development. Scientists and engineers are diving into the properties of novel compounds, exploring their thermoelectric efficiency with an eager eye.
Emerging materials, like topological insulators or nanostructured semiconductors, are stepping into the spotlight. These materials show a higher thermoelectric figure of merit, which means they can convert temperature differences into usable electric power more efficiently. Key factors, such as cost, scalability, and sustainable sourcing, play pivotal roles in the material selection process. Researchers are also investigating organic thermoelectric materials due to their lower environmental impact and significant flexibility, opening avenues for integration into varied applications.
Here are some notable points about the ongoing advancements in material science:
- Improved Thermoelectric Performance: New compounds like skutterudites and half-Heusler alloys are showing impressive efficiency levels.
- Sustainable Materials Development: Focus on materials that are abundant and non-toxic can lead to more eco-friendly products.
- Nano-engineering: Techniques to manipulate materials at the nanoscale can significantly enhance thermoelectric properties.
Integration with Renewable Energy Sources
As the world pivots toward renewable energy, thermoelectric refrigeration must integrate seamlessly with alternative energy sources. Utilizing green energy not only enhances the sustainability of thermoelectric systems but also allows them to operate more efficiently in diverse environments. By leveraging solar, wind, or geothermal energy, thermoelectric devices could operate in remote areas or places where traditional systems fall short.
Consider this scenario: a thermoelectric cooling unit powered by solar panels situated in arid regions. The ability to generate electricity and cooling simultaneously opens the door to solutions far beyond conventional setups. Below are some key implications of integrating thermoelectric refrigeration with renewable energy sources:
- Enhanced Autonomy: Self-sufficient cooling units can develop in remote locations without the need for extensive infrastructure.
- Energy Storage Synergies: Such systems can be coupled with thermal energy storage, helping to balance loads and improve overall efficiency.
- Reducing Carbon Footprint: By sourcing energy from renewables, the environmental impact diminishes, promoting a greener planet.
End
In recapping the significance of thermoelectric refrigeration, the discussion shines a light on how this technology has evolved into a crucial player in modern cooling systems. This article explored the principles that underpin thermoelectric refrigeration, from fundamental concepts to in-depth analyses of materials and operational dynamics.
Thermoelectric refrigeration offers benefits not just in terms of environmental impact, but also in portability and minimal maintenance. This is particularly meaningful in a world increasingly prioritizing sustainability alongside technology's advances. Unlike conventional systems that rely heavily on refrigerants, thermoelectric devices provide an eco-friendly alternative. They utilize the thermoelectric effect to cool down spaces, which aligns well with current trends aiming to reduce harmful emissions.
Moreover, the article highlights challenges that come along with these innovations. For instance, the cost and performance efficiency compared to traditional cooling methods remain matters to tackle. The implications of these issues suggest that while immediate benefits are quite apparent, long-term solutions and advancements will be necessary for broader adoption.
In summary, the key points established throughout the article reveal a dual narrative: an optimistic view of thermoelectric refrigeration's potential and a practical approach towards recognizing its hurdles. The fusion of technology with eco-consciousness is not just a passing trend; it’s essential for the future of cooling technologies.
"Understanding thermoelectric refrigeration is not simply a matter of science; it's about anticipating the next step in energy efficiency and environmental responsibility."
Summary of Key Points
- Eco-Friendliness: Thermoelectric refrigeration reduces reliance on harmful refrigerants, potentially making it an environmentally safer option.
- Compactness: The ability to create smaller, more portable devices provides versatility across applications, from consumer electronics to automotive solutions.
- Low Maintenance: These systems generally require less upkeep than traditional refrigeration methods, offering convenience in various use cases.
- Cost and Performance Challenges: While benefits are clear, the financial and efficiency barriers remain considerations for widespread application.
Implications for Future Research
Looking ahead, the realm of thermoelectric refrigeration opens multiple doors for research. Investigating advanced materials capable of enhancing the efficiency of thermoelectric devices is paramount. This substitutes for traditional thermoelectric materials that can often be expensive and have limited performance metrics.
Exploring integration with renewable energy sources could also be a significant avenue. Harnessing solar or wind power could enable thermoelectric systems to operate independent of traditional energy grids, providing sustainable solutions for off-grid applications. Furthermore, developing better thermal management strategies might help tackle current performance limitations, leading to high-performance products suitable for various industries.
In summary, the journey of thermoelectric refrigeration is just beginning. New materials, deployment scenarios, and sustainability measures could very well create a new chapter in this technology's story, one that addresses the urgent demands of today's environment.