Transfection of siRNA: Mechanisms and Applications
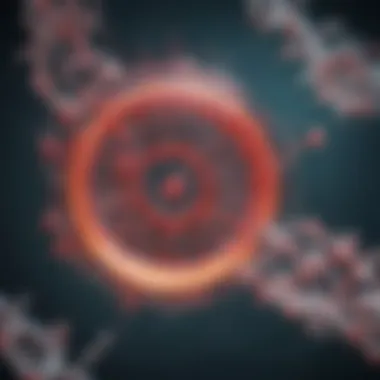
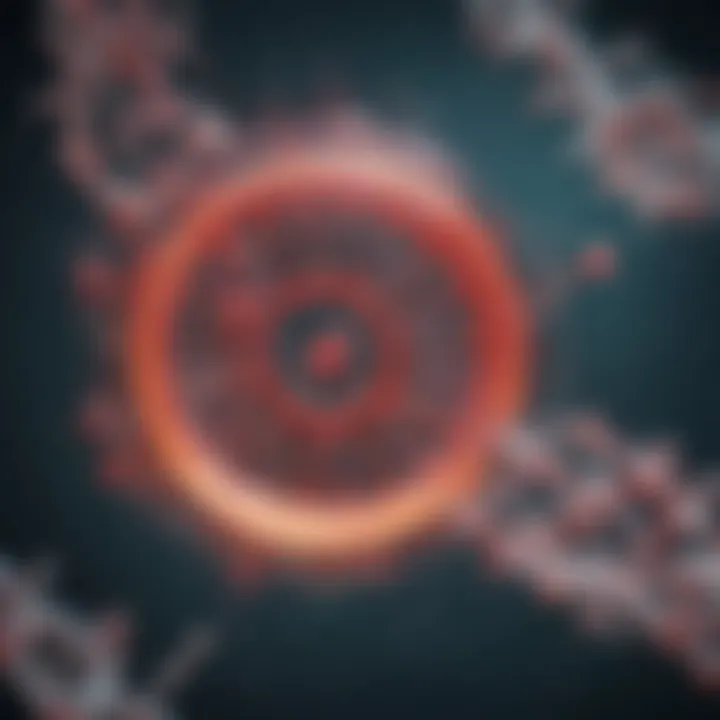
Intro
The transfection of small interfering RNA (siRNA) is pivotal in modern molecular biology. Gene silencing via siRNA has opened many avenues for research as well as treatment. This technique allows for targeted modulation of gene expression, making it an invaluable tool in both laboratory and clinical settings. Understanding the intricacies of siRNA transfection—how it functions, the methods used to achieve it, and its applications—enables researchers to harness its full potential for innovative therapies.
Research Highlights
Overview of Key Findings
The key findings in the study of siRNA transfection reveal its significance in gene regulation. siRNA operates by binding to complementary mRNA molecules, resulting in their degradation and subsequent silence of targeted genes. Significant aspects worth noting include:
- Various methods of siRNA delivery, each with their own strengths and challenges.
- The role of cellular uptake mechanisms in effective transfection.
- Optimizing conditions to enhance transfection efficiency.
- Applications beyond basic research, such as gene therapy, cancer treatments, and combating viral infections.
Significance of the Research
Research into siRNA transfection is critical for advancing genetic engineering techniques. The implications of successful gene silencing extend from elucidating gene function to developing therapeutics for complex diseases. By understanding the mechanisms involved in siRNA activity, scientists can develop better strategies for gene modulation, thereby contributing to significant improvements in targeted therapies.
"The ability to silence specific genes is not only fundamental for research but also transformative in clinical applications."
Techniques and Mechanisms Involved
The mechanisms behind siRNA transfection are complex but essential to grasp for effective application. Key processes include the following:
- siRNA Design: Understanding target sequences is crucial for designing effective siRNAs. Careful selection reduces off-target effects.
- Transfection Methods: Various techniques are used to introduce siRNA into cells. These include lipofection, electroporation, and viral vectors, among others. Each method has its particular use case, advantages, and limitations.
- Optimization of Delivery: Success in siRNA studies often relies on optimizing parameters like concentration, transfection reagent, and cell type. Simple adjustments can lead to significantly improved outcomes.
These elements should be closely considered when preparing experiments aiming to utilize siRNA transfection.
Ending
Prolusion to siRNA
Small interfering RNA (siRNA) has revolutionized the field of molecular biology, serving as a key tool for gene silencing. Its significance lies in the ability to manipulate gene expression with precision, providing insights into gene function and the underlying mechanisms of diseases. As this article endeavors to explore, understanding siRNA is essential for both research and therapeutic applications.
To fully appreciate the role of siRNA, one must begin with its fundamental definitions and structures that define it as a critical player in genetic regulation. Understanding siRNA's background enables researchers to harness its potential effectively. This section lays the groundwork for the subsequent exploration of its mechanisms, the various techniques of transfection, and its extensive applications across scientific and clinical contexts. A deep appreciation of siRNA’s properties is crucial not just for its application but also for informing the ongoing development of more effective delivery systems and transfection techniques.
Definition and Structure
siRNA refers to small, double-stranded RNA molecules, typically 20 to 25 base pairs in length. These molecules play an integral role in the RNA interference (RNAi) pathway, where they guide the cellular machinery to silence specific genes. The structure of siRNA consists of two strands: the guide strand and the passenger strand.
The guide strand is tasked with complementary pairing to its target messenger RNA (mRNA), leading to gene silencing. The passenger strand is often discarded during the RNAi process. The presence of 2-base overhangs at the 3' ends helps with the incorporation into the RNA-induced silencing complex (RISC), which is pivotal for the gene silencing process.
Historical Context
The history of siRNA is entwined with the discovery of RNA interference itself, first observed in the late 1990s. The term ‘‘RNA interference’’ was introduced after researchers found that injecting double-stranded RNA into C. elegans led to the specific silencing of target genes. This phenomenon attracted attention and propelled the investigation into its mechanisms and applications.
The development of synthetic siRNA in laboratories coincided with advances in molecular techniques, allowing scientists to explore siRNA as a versatile tool in functional genomics and therapeutic research. Over the years, the methodologies for generating and using siRNA have evolved, shaping the landscape of gene silencing and establishing siRNA as a prominent player in both academic research and clinical applications.
Mechanism of siRNA Action
The mechanism of siRNA action is fundamental to its effectiveness as a tool for gene silencing. Understanding this mechanism sheds light on the intricate processes involved in RNA interference, offering insights into how siRNA can selectively downregulate target genes. This section aims to engage with the core principles that govern the action of siRNA and elaborate on their significance in both research and therapeutic contexts.
RNA Interference Pathway
The RNA interference (RNAi) pathway is a natural cellular mechanism that regulates gene expression and protects against viral attacks. This process begins when long double-stranded RNA (dsRNA) is introduced into a cell. Dicer, an enzyme native to the cell, cleaves the dsRNA into small fragments of approximately 21-23 nucleotides. These fragments are known as small interfering RNAs (siRNAs).
Upon the creation of siRNAs, the next step involves their incorporation into the RNA-induced silencing complex (RISC). This complex is pivotal for the subsequent actions of siRNA. The strands within the siRNA duplex are unwound, allowing one strand, referred to as the guide strand, to associate with RISC. Meanwhile, the passenger strand is typically discarded. The guide strand is now poised to seek out complementary mRNA targets.
Once associated with RISC, the siRNA guide strand hybridizes with its complementary mRNA. This hybridization can trigger the cleavage of the mRNA at the site of binding, effectively silencing the gene it encodes. Alternatively, siRNA can also inhibit translation, thereby blocking the production of proteins that the mRNA would otherwise generate. It is this precision and efficiency in targeting specific RNA molecules that makes siRNA an indispensable tool in molecular biology.
The exquisite specificity of siRNA to its target mRNA showcases the potential of RNA interference for therapeutic applications, particularly in the context of cancer and infectious diseases.
Role of Dicer and RISC Complex
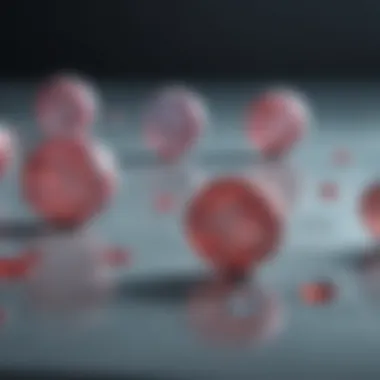
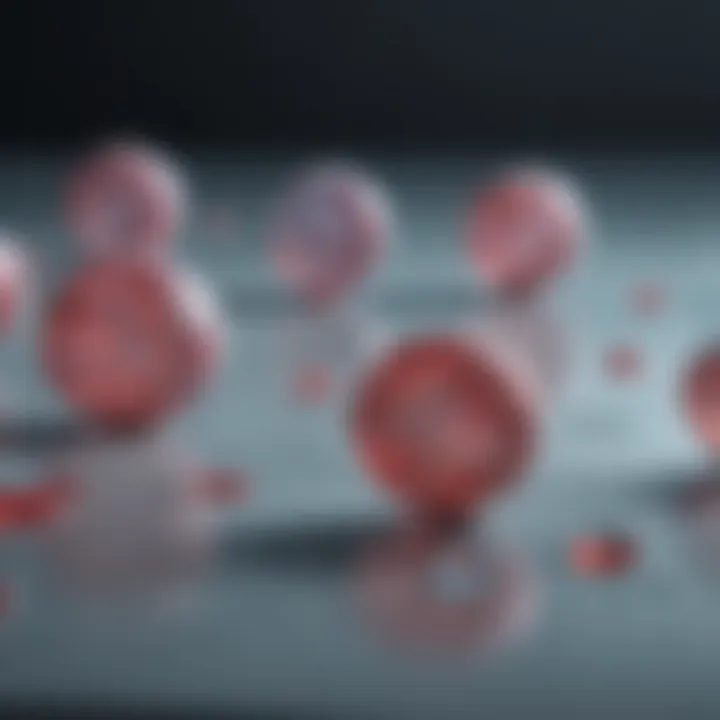
Dicer and the RISC complex play crucial roles in the siRNA pathway. Dicer is an RNAse III-like enzyme that recognizes and processes long dsRNA into short, functional siRNA. Its accuracy ensures that the generated siRNAs are optimal for incorporation into RISC. Dicer's activity is regulated, necessitating suitable subcellular localization and timely expression to maintain effective siRNA-mediated silencing.
The RISC complex, which includes proteins such as Argonaute, is where siRNA exerts its action. Argonaute is fundamental to RISC's ability to bind and cleave the target mRNA. The presence of the guide strand facilitates this interaction, allowing for a very specific targeting mechanism. The result is a potent and effective downregulation of genes that contribute to various pathological conditions.
In summary, understanding the mechanisms of siRNA action is essential for optimizing its use in gene therapy and other biomedical applications. Both Dicer and the RISC complex form a sophisticated system that allows siRNA to exert its effects with high specificity, underscoring the value of this approach in modern molecular biology. Integrating such knowledge informs both research methods and therapeutic strategies involving RNA interference.
Transfection Techniques
In the realm of molecular biology, transfection techniques are pivotal for introducing small interfering RNA (siRNA) into cells. These methods are essential for research and therapeutic applications where precise gene silencing is needed. Understanding and choosing the right transfection technique can significantly affect the outcome of experimental procedures.
Chemical Methods
Lipofection
Lipofection is a widely utilized method for transfecting siRNA into various cell types. This technique involves the use of lipid-based reagents that form liposomes, which encapsulate the siRNA. The liposomes facilitate the fusion with the cell membrane, allowing for an efficient delivery of siRNA into the cytoplasm.
A key characteristic of lipofection is its simplicity and versatility. It is compatible with many cell types, making it a popular choice for researchers. The unique feature of lipofection lies in the formulation of the lipids. Various types of lipids can be optimized to enhance transfection efficiency and minimize cytotoxicity. However, one disadvantage is that lipofection may lead to off-target effects if not carefully managed.
Polymer-Based Methods
Polymer-based methods employ polymers to encapsulate siRNA for cellular delivery. Often, these polymers possess cationic properties enabling them to form complexes with negatively charged siRNA. This technique is important because it can provide a higher stability and protect siRNA from degradation in biological fluids.
The key characteristic of polymer-based methods includes their ability to be tailored for specific applications. Researchers can modify the polymers for differing cellular uptake and release profiles. A unique feature is their potential for sustained release of siRNA, which can lead to prolonged effects. However, toxicity remains a concern, as some polymers can induce significant cellular responses that may affect experimental results negatively.
Physical Methods
Electroporation
Electroporation is a physical method that uses electrical pulses to create temporary pores in the cell membrane, facilitating the entry of siRNA directly into the cytoplasm. This technique is highly effective, especially for hard-to-transfect cells like primary and stem cells.
A key characteristic of electroporation is its efficiency. It often achieves higher transfection rates compared to chemical methods. The unique feature of electroporation is that it can be optimized by adjusting voltage, pulse duration, and number of pulses. However, it does carry a risk of cellular apoptosis and can lead to cell lysis if the conditions are not carefully controlled.
Microinjection
Microinjection is a precise method that involves directly injecting siRNA into individual cells using a fine glass needle. This technique is optimal for applications where targeted delivery to specific cells is crucial, such as in embryos or during early developmental stages.
The key characteristic of microinjection is its specificity, allowing researchers to inject into only the cells of interest. A unique feature of this method is its high level of control, resulting in immediate and potent transfection. However, it is technically demanding and time-consuming, which limits scalability and makes it less practical for large-scale studies.
Viral Methods
Adenoviral Vectors
Adenoviral vectors are modified viruses that can efficiently deliver siRNA to host cells. These vectors can transduce both dividing and non-dividing cells, making them a versatile tool in gene therapy and research.
A key characteristic of adenoviral vectors is their high transfection efficiency. Their ability to accommodate large transgene inserts offers flexibility in experimental design. A unique feature is their relatively low immunogenicity compared to other viral vectors, although they can still provoke immune responses. A disadvantage is that adenoviral vectors typically do not integrate into the host genome, leading to transient expression of siRNA.
Lentiviral Vectors
Lentiviral vectors are another class of viral methods used for siRNA transfection. These vectors, derived from HIV, have the capability to stably integrate into the host cell genome, allowing for long-term expression of siRNA.
The key characteristic of lentiviral vectors is their ability to transduce non-dividing cells, expanding the range of potential target cells. The unique feature is their stability, enabling sustained silencing effects over time. Nonetheless, their usage raises safety concerns due to the potential for insertional mutagenesis, which must be carefully evaluated in experimental designs.
Nanoparticle Delivery Systems
Nanoparticle delivery systems represent an innovative approach to siRNA transfection. These systems utilize nanoparticles to transport siRNA across the cell membrane effectively. They can improve the stability and bioavailability of siRNA in biological environments.
A key characteristic of nanoparticle delivery is their customization potential. Researchers can modify particle size and surface charge to enhance cell interaction and uptake. A unique feature of these systems is their ability to provide targeted delivery through active or passive mechanisms, increasing the precision of gene silencing. However, challenges remain in ensuring consistent and reproducible results across different cell types.
Optimization of siRNA Transfection
Optimization of siRNA transfection is essential for achieving effective gene silencing. This process involves manipulating various factors to enhance the delivery of siRNA into target cells. Special attention is needed to maximize transfection efficiency while minimizing potential negative effects. Proper optimization can significantly influence experimental outcomes and subsequent analyses.
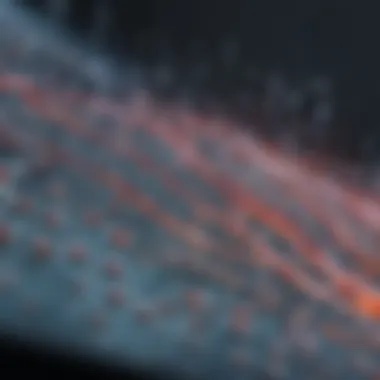
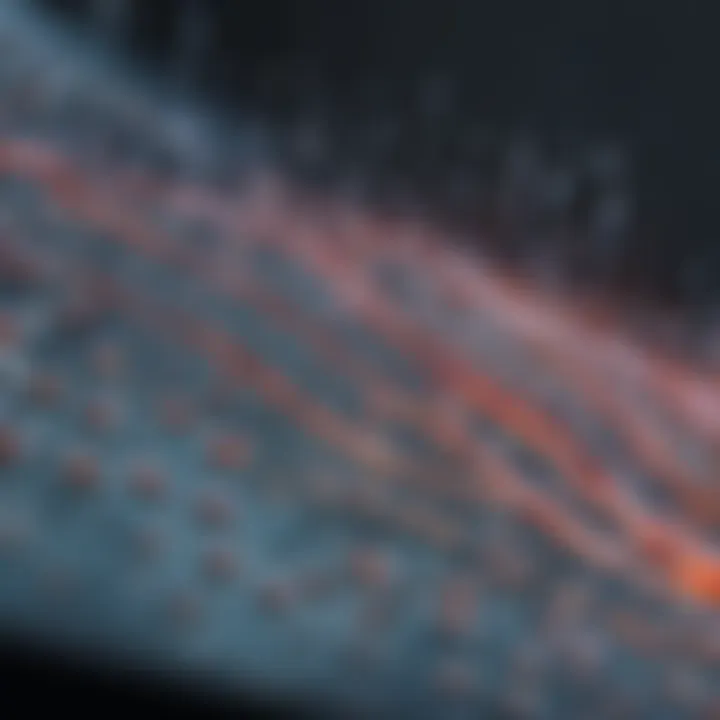
In practical terms, there are specific elements that researchers must consider. These include the choice of transfection method, the design of siRNA molecules, and the characteristics of the target cells. All these aspects contribute directly to the effectiveness of the transfection process. An optimized approach can lead to more reliable results, making it a critical focus in the field of molecular biology.
Factors Affecting Transfection Efficiency
Cell Type Compatibility
Cell type compatibility plays a crucial role in the success of siRNA transfection. Different cell lines exhibit varying levels of susceptibility to transfection agents. Understanding the cellular environment ensures that the chosen method aligns with the specific characteristics of the cell type in question.
A key characteristic of cell type compatibility is its influence on the uptake of siRNA. For instance, some cell lines, such as HeLa or HEK293, are more amenable to transfection than others, like primary cells or certain tumor cells. This compatibility is a beneficial choice for experiments needing consistent and reproducible results. Additionally, the unique feature of selecting a compatible cell type is to improve the transfection efficiency, enhancing the likelihood of achieving targeted silencing of genes.
However, some disadvantages exist. Not all cell types can be easily transfected, which may limit the scope of certain experiments and require alternative strategies.
siRNA Design and Concentration
Another vital aspect influencing siRNA transfection is the design and concentration of the siRNA used. Optimal design significantly affects the stability, potency, and specificity of siRNA molecules. Researchers have found that tailored siRNA sequences lead to enhanced silencing effects. Moreover, the concentration of siRNA must be carefully balanced. Too little may not produce the desired effects, while excessive amounts could induce off-target effects.
A primary consideration is that well-designed siRNA contributes to not only higher efficiency but also reduced side effects. Unique features in siRNA structure, including chemical modifications, can increase resistance to degradation and enhance target binding, benefiting overall experimental outcomes.
However, increasing concentration can complicate the protocol and introduce variables affecting results, potentially leading to varied levels of gene knockdown.
Monitoring and Evaluation Techniques
Ensuring the success of siRNA transfection requires effective monitoring and evaluation. Employing robust techniques allows researchers to quantitate the extent of gene silencing and validate their results, guiding them on the effectiveness of their transfection protocols.
Western Blot Analysis
Western blot analysis is a widely used method for monitoring protein expression levels post-transfection. This technique provides qualitative and quantitative data by detecting specific proteins, allowing researchers to evaluate the efficacy of siRNA in silencing targeted genes.
The key characteristic of Western blot analysis is its ability to confirm the absence or reduction of the protein of interest. It serves as a beneficial choice due to its straightforward procedure and clear results. One unique feature is its capability to detect modifications in protein expression, providing additional insights into cellular responses.
Nonetheless, Western blot analysis has its disadvantages. It is time-consuming and can be technically demanding. Variabilities in protein loading and transfer efficiency can also lead to misleading results if not correctly controlled.
qPCR Validation
Quantitative Polymerase Chain Reaction (qPCR) validation stands as another critical technique in monitoring siRNA activity. This method quantitatively assesses mRNA levels, thereby offering direct insights into gene expression changes following siRNA treatment.
The primary strength of qPCR lies in its sensitivity and specificity. It allows for the quantification of even small changes in mRNA levels, making it an effective tool for validating siRNA knockdown. A unique feature is its speed compared to Western blot analysis, which shortens the timeframe for obtaining results.
However, qPCR also presents challenges. Its performance heavily relies on the quality of the primers used and the efficiency of the reactions, which can sometimes introduce complexities. Hence, careful design and optimization are necessary to achieve reliable outcomes.
Optimization of transfection techniques and subsequent monitoring methods is vital for advancing siRNA research and ensuring impactful results.
Challenges in siRNA Transfection
The transfection of small interfering RNA (siRNA) presents unique challenges that can impact efficiency and efficacy. It is crucial to address these challenges to maximize the potential of siRNA as a tool in gene silencing. Several factors influence the success of siRNA delivery, which is essential in both research and clinical applications. Understanding these elements provides clarity on how to improve transfection methods and their outcomes.
Cellular Uptake and Stability Issues
One of the primary obstacles in siRNA transfection is the efficient uptake of siRNA by target cells. Cellular membranes are composed of lipid bilayers that act as barriers to large molecules such as siRNA. This necessitates the use of various delivery methods to facilitate uptake. Different cell types exhibit varying levels of permeability to siRNA, meaning that what works for one cell type may not apply to another.
Moreover, once siRNA enters the cell, it must remain stable before it reaches its target. siRNAs are inherently susceptible to degradation by nucleases present in the cellular environment. This degradation can lead to reduced efficacy and ultimately negate the intended silencing effect. For siRNA to exert its function effectively, it may require modifications such as chemical stabilization or encapsulation within protective carriers. Such strategies ensure that siRNAs can survive longer within the cellular milieu and reach their intended target efficiently.
Off-Target Effects
Another significant challenge associated with siRNA transfection is off-target effects. While siRNAs are designed to specifically target and silence complementary mRNA sequences, they may inadvertently interact with non-target mRNAs due to sequence similarities. This unintentional silencing can lead to unintended consequences on cellular functions, potentially skewing experimental results and raising concerns in therapeutic applications.
Consequently, it has become increasingly important to thoroughly validate the specificity of siRNA sequences prior to their use. Several approaches can be employed to account for off-target effects, including:
- Utilizing computational tools to predict potential off-target interactions
- Designing siRNAs with unique sequences that are less likely to match non-target mRNA
- Conducting control experiments to assess the specificity of observed gene silencing effects
By addressing the challenges of cellular uptake, stability, and off-target effects, researchers can enhance the reliability of siRNA transfection techniques. The continued exploration and refinement of methods related to these challenges signify a critical pathway towards maximizing the therapeutic and research applications of siRNA.
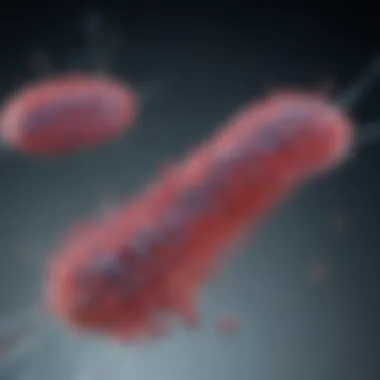
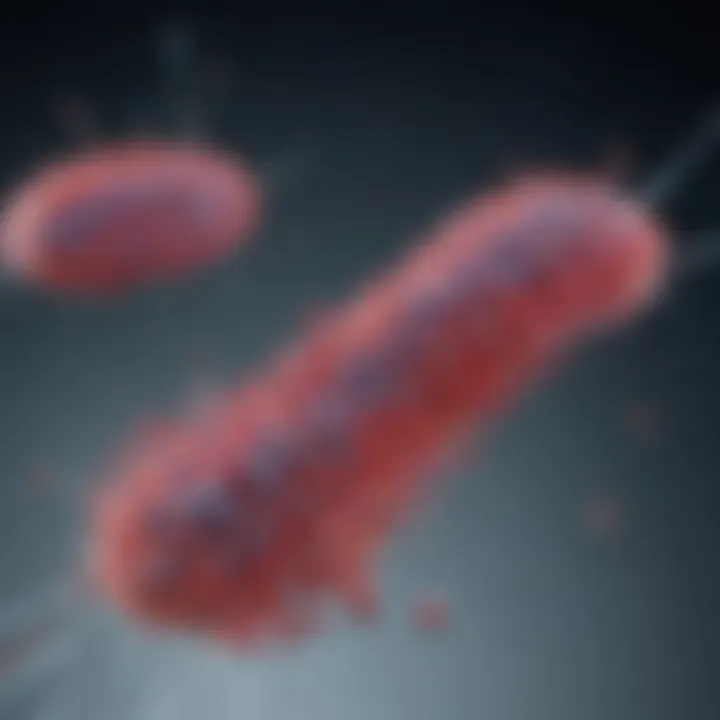
Applications of siRNA Transfection
The applications of siRNA transfection are pivotal in both research and therapeutic contexts. siRNA technology has revolutionized the way scientists investigate gene function and develop treatment strategies for various diseases. By utilizing siRNA, researchers can effectively silence specific genes, offering insights into their roles in cellular pathways and involved health conditions. Moreover, the therapeutic potential of siRNA is remarkable, particularly for conditions that have few effective treatments, such as certain cancers and viral infections.
In Research
Gene Function Studies
Gene function studies using siRNA transfection allow scientists to explore the roles of individual genes within biological systems. This methodology provides a means to temporarily knock down gene expression, facilitating the examination of the resulting phenotypic changes. One unique characteristic of gene function studies is their ability to link genetic data to biological outcomes, making it a powerful tool for functional genomics.
The benefits of employing siRNA in these studies lie in the precision with which gene expression can be regulated. Researchers can target genes specifically, minimizing off-target effects and leading to clearer interpretations of results. However, one consideration is the transient nature of siRNA-induced knockdown, which may limit long-term observations.
Pathway Analysis
Pathway analysis benefits significantly from siRNA transfection as it allows researchers to dissect complex signaling networks. By silencing key genes in particular pathways, scientists can assess the functional impact on downstream effects. This method is particularly beneficial in identifying novel interactions and components of signaling cascades that contribute to physiological processes and disease states.
A key feature of pathway analysis is the ability to observe changes in cellular behavior that exhibit critical linkage to specific pathways. The insights derived from these studies can pave the way for drug discovery and targeted therapies. However, the complexity of pathways may sometimes complicate the interpretation of the results, especially if certain genes have redundant functions.
In Therapeutics
Cancer Treatment
In the realm of cancer treatment, siRNA transfection holds substantial promise as a therapeutic strategy. Targeting oncogenes or genes involved in tumor progression can inhibit cancer cell growth and metastasis. The precision of siRNA allows for specific targeting of genes that may contribute to resistance against conventional therapies, enhancing treatment efficacy.
One significant characteristic of cancer treatment via siRNA is its ability to potentially overcome adverse side effects associated with traditional therapies. Unlike broad-spectrum drugs, siRNA can be tailored to target specific tumor genotypes. Nonetheless, challenges include efficient delivery to tumor sites and ensuring sufficient stability of the siRNA molecules once administered.
Viral Infections
For viral infections, siRNA transfection emerges as an innovative approach to combat diseases like HIV and Hepatitis C. By targeting viral genomes or essential host factors aiding viral replication, siRNA can significantly reduce viral load, offering a method to manage infections more effectively.
The key advantage of targeting viral infections with siRNA lies in its specificity and potential for development into therapies that do not rely on classical antiviral drugs. Furthermore, siRNA can be designed to adapt to rapidly mutating viruses, which is a notable benefit. However, the delivery mechanisms remain a critical challenge, as effective uptake into infected cells is essential for achieving therapeutic success.
"The applications of siRNA transfection in research and therapeutics offer a new horizon for genetic studies and disease treatment, emphasizing the importance of targeted approaches in modern biomedicine."
Future Directions in siRNA Research
The exploration of siRNA transfection is continually evolving with advances in technology and increasing understanding of genetic mechanisms. Future directions in siRNA research present significant possibilities that could enhance gene therapy and other applications in the biomedical field. This section discusses emerging technologies, as well as the potential for personalized medicine, emphasizing their relevance and implications for research and practice.
Emerging Technologies
The emergence of innovative technologies is pivotal in refining siRNA transfection methods. Here are some key areas of development:
- Nanotechnology: Utilization of nanoparticles for siRNA delivery is gaining traction. Nanoparticles can protect siRNA from degradation and facilitate its uptake by target cells. This approach offers improved stability and specificity in targeting disease-associated genes.
- CRISPR-Cas Systems: Combining siRNA with CRISPR technology offers enhanced capability to edit genomes. This combination may allow researchers to achieve more precise gene silencing and editing, expanding the potential uses for siRNA in therapeutic contexts.
- Microfluidics: This technology enables the manipulation of small fluid volumes, which can streamline the siRNA transfection process. Through precise control over the environment, microfluidics can improve transfection efficiency and reduce waste.
Emerging technologies such as these hold the promise to optimize the transfection efficiency of siRNA and broaden its applications in both research and clinical settings.
Potential for Personalized Medicine
Personalized medicine represents a shift towards tailored healthcare based on individual genetic profiles. In the context of siRNA transfection, this approach holds great promise.
- Targeted Gene Therapy: With a deeper understanding of genetic variation among patients, siRNA can be modified to target specific mutations in individuals. This ability could lead to more effective treatments for genetic disorders, reducing side effects associated with conventional therapies.
- Biomarker Development: Identifying biomarkers associated with specific diseases can lead to the development of siRNA therapies that address these conditions directly. Personalized siRNA constructs can significantly enhance the therapeutic outcomes.
- Adaptation to Resistance Mechanisms: Understanding how certain cancers develop resistance to treatment can guide the design of siRNA therapies tailored to bypass these mechanisms, providing better responses and outcomes.
As research progresses, integrating siRNA with personalized medicine approaches may revolutionize how diseases are treated, enhancing efficacy while minimizing adverse effects.
The integration of emerging technologies with personalized medicine in the field of siRNA transfection could reshape therapeutic strategies, making treatments more effective and patient-specific.
End
The conclusion serves as a critical evaluation of the topics discussed throughout the article, particularly emphasizing the significance of siRNA transfection in modern molecular biology and genetic research. It synthesizes the key points, reflecting on the mechanisms by which siRNA functions and the various techniques available for its transfection.
Understanding the conclusion is essential for several reasons:
- Integration of Knowledge: The conclusion encapsulates the essence of siRNA transfection. By blending insights regarding its mechanisms, techniques, and applications, it provides a cohesive picture of how siRNA can be utilized for gene silencing.
- Practical Implications: The article highlights the relevance of optimizing transfection methods and understanding challenges such as off-target effects. These are important considerations for researchers and practitioners who wish to apply siRNA in experimental or clinical settings.
- Future Perspectives: In recognizing the potential of siRNA in personalized medicine, the conclusion opens up avenues for future research. It encourages readers to consider how continued advancements in this area may improve therapeutic interventions and contribute to better health outcomes.
"An awareness of the complexities involved in siRNA transfection paves the way for innovation in gene therapy and molecular biology."
The conclusion ultimately reinforces the role of siRNA transfection as a foundational technique in both research and therapeutic applications. It invites further exploration into the future of gene silencing approaches that could lead to breakthroughs in understanding genetic diseases, offering hope for new therapeutic strategies.