Understanding LFP Cells: A Comprehensive Overview
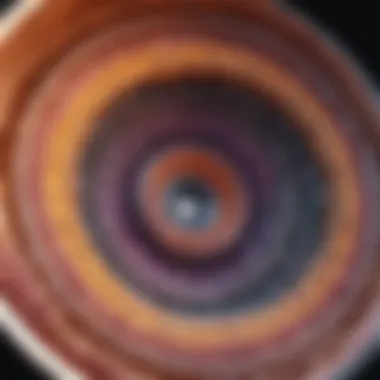
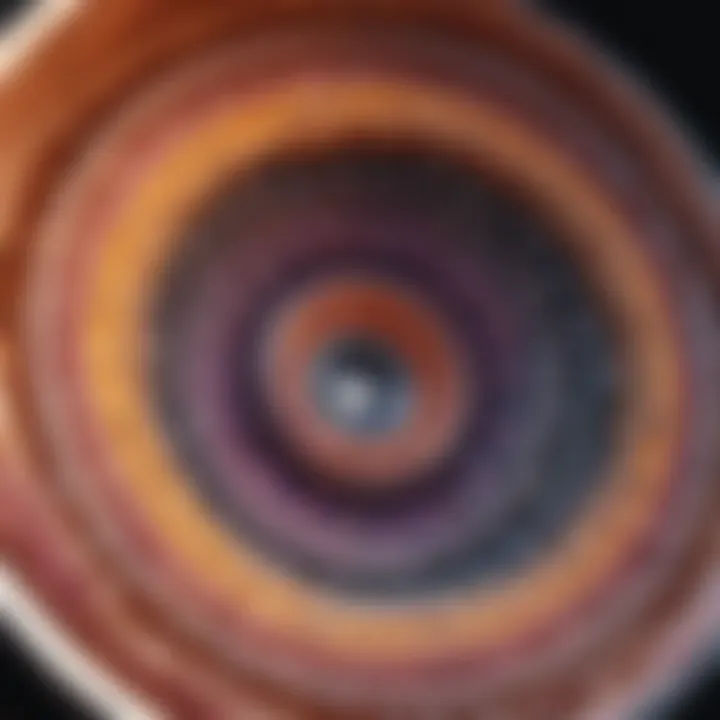
Intro
Lithium Iron Phosphate (LFP) cells have gained significant attention in recent years. Their unique composition and operational principles set them apart within the domain of energy storage. This section offers a foundational understanding of LFP cells, focusing on their structure, functionality, and rising prominence in various applications.
LFP cells consist of lithium iron phosphate as the cathode material, which is responsible for the storage of lithium ions during operations. Unlike other lithium-ion technologies, LFP cells use iron rather than cobalt for cathodes, enhancing safety and cost-effectiveness. The anode is typically made of graphite, allowing ion transport while ensuring high energy capacity.
The operational principle of these cells involves intercalation and deintercalation of lithium ions. When charged, lithium ions move from the cathode to the anode, and during discharge, they return to the cathode, creating an electric current. This process is more stable than in other lithium-ion battery technologies, which contributes to the overall safety of LFP cells.
Interest in LFP cells is driven by their advantages such as longer lifespan, thermal stability, and lower toxicity. These attributes make them suitable for a wide range of applications, including electric vehicles, energy storage systems, and even power tools. Nevertheless, LFP cells also face limitations such as lower energy density compared to other lithium-ion batteries.
In this article, we will explore the composition, advantages, challenges, and the latest advancements concerning LFP technology, as well as its potential to address today's energy problems. Through detailed analysis, we aim to provide a holistic view of LFP cells, catering to students, researchers, educators, and professionals who are interested in this field.
Prologue to LFP Cells
Lithium Iron Phosphate (LFP) cells represent a significant advancement in battery technology. Their relevance in today's energy landscape cannot be overstated. As we seek sustainable energy solutions, LFP cells offer unique advantages that align with the needs of various applications, making them a focal point of research and development within the battery sector.
Understanding LFP cells includes exploring their design, functionality, and performance metrics. This section highlights their importance, considering various factors that influence their performance and effectiveness. Through this analysis, readers will gain insights into why LFP technology is becoming increasingly popular in industries ranging from electric vehicles to renewable energy systems.
Definition and Basic Principles
LFP cells are a type of lithium-ion battery that uses lithium iron phosphate as the cathode material. The chemical formula, LiFePO4, illustrates their basic structure. They operate on the principle of ion movement: lithium ions migrate between the anode and cathode during charge and discharge cycles.
The energy is stored when the lithium ions move to the cathode. Conversely, during discharging, lithium ions travel back to the anode, releasing energy. These reactions do not produce harmful byproducts and are more stable compared to other lithium-ion technologies. This stability leads to enhanced safety and lower risks associated with overheating or combustion.
Historical Development
The development of LFP cells began in the late 1990s. Researchers discovered that lithium iron phosphate provided not only safety benefits but also an extended cycle life compared to traditional lithium-ion batteries. The technology gained traction in the early 2000s, and it became a more viable option as concerns over battery safety increased.
In more recent years, manufacturers have focused on improving energy density and overall efficiency. Advances in electrode materials and design continue to enhance their market presence. The rise in electric vehicle adoption has also catapulted LFP cells into the spotlight, since their inherent safety and longevity make them ideal for this application.
Comparative Analysis with Other Battery Technologies
Understanding LFP cells requires contextualizing them alongside other battery technologies. Compared to traditional lithium-ion batteries that use cobalt oxide, LFP offers several benefits. First is their improved thermal stability. Second, LFP batteries are more environmentally friendly, as they do not contain cobalt, which is often associated with unethical mining practices.
In terms of energy density, LFP cells typically fall short when compared to Nickel Manganese Cobalt (NMC) cells. However, their advantages often outweigh this limitation in specific applications. For instance:
- Safety: LFP cells are less likely to catch fire, which is a critical factor for applications like electric vehicles.
- Life Cycle: They can achieve higher cycle counts, often exceeding 2000 cycles.
- Cost: LFP materials are generally less expensive, leading to a lower cost per kilowatt-hour.
Chemical Composition and Structure of LFP Cells
The chemical composition and structure of Lithium Iron Phosphate (LFP) cells play a crucial role in defining their performance and applications. Understanding the specific elements involved in LFP technology helps to highlight its advantages and limitations. Additionally, insights into the composition offer perspectives on how these batteries can be optimized for various uses.
Raw Materials Used in LFP Cells
LFP cells are primarily composed of lithium, iron, phosphorus, and oxygen. Each element serves a distinctive purpose contributing to the battery’s overall properties:
- Lithium: This alkali metal is vital for electrochemical reactions, serving as the primary charge carrier in the battery. Its lightweight characteristic enables high energy density.
- Iron: Iron phosphate provides structural integrity and stability to the cathode material. Unlike other lithium-based batteries which use cobalt, using iron makes LFP more environmentally friendly.
- Phosphorus and Oxygen: These elements are integral to forming the phosphate structure, which helps enhance thermal stability and safety. The stable structure reduces risks related to thermal runaway, a common concern in battery technologies.
The selection of raw materials not only influences performance but also emphasizes sustainability, a key consideration for modern energy solutions.
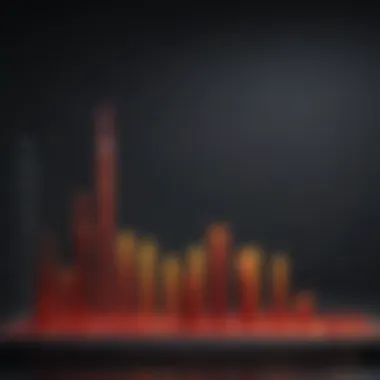
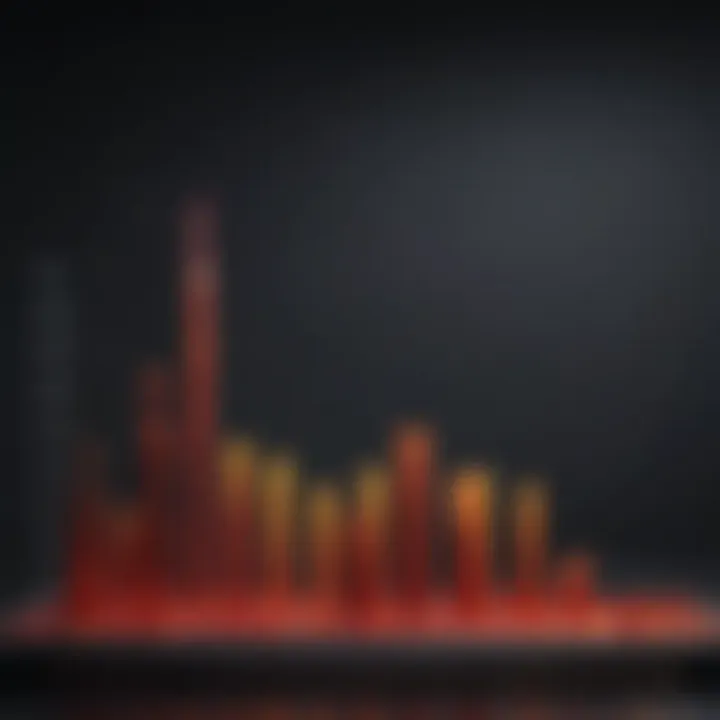
Electrode Composition and Structure
The electrodes in LFP cells consist mainly of lithium iron phosphate for the cathode and generally graphite for the anode. The distinct characteristics of these materials contribute to the overall efficiency and functioning of the battery.
- Cathode Composition: The cathode is composed of LFP particles designed to maximize surface area and facilitate lithium-ion movement. This design contributes to the high cycle stability and longevity of LFP cells.
- Anode Composition: Typically, the anode consists of graphite, which offers a good balance of energy density and electrical conductivity. Together with the cathode, these components create a robust electrochemical environment.
- Structural Arrangement: The arrangement of these materials impacts the electrochemical processes during charge and discharge cycles. A well-structured electrode results in improved efficiency and faster charge rates, which are significant factors for applications like electric vehicles and energy storage systems.
Electrolytes in LFP Cells
The electrolyte in LFP cells is crucial for conducting ions between the anode and cathode during the charge and discharge processes. Most LFP batteries utilize liquid electrolytes, commonly lithium salt dissolved in organic solvents.
- Conductivity: The electrolyte needs to have high ionic conductivity while being stable over a wide temperature range to ensure reliable performance.
- Safety Considerations: The choice of electrolyte affects safety. Organic solvents can be flammable. Thus, advancements have been made towards solid-state electrolytes, which could potentially eliminate many safety concerns associated with liquid electrolytes.
- Performance Effects: The type of electrolyte and its concentration directly influence the battery's overall efficiency, thermal stability, and cycle life. Research continues to explore these elements for improved performance in LFP cells.
Important Note: The efficacy of LFP cells is largely contingent on the meticulous selection and combination of these raw materials, electrode composition, and electrolyte formulations. Their unique structure helps navigate some of the challenges found in other lithium-ion batteries.
Operating Mechanisms of LFP Cells
The operating mechanisms of Lithium Iron Phosphate (LFP) cells are crucial for understanding how these batteries function effectively within various applications. By exploring the intricacies of charge and discharge cycles, energy density mechanisms, and safety considerations, one can better appreciate the strengths and limitations of LFP cells. This section delves into these various aspects, highlighting their significance and relevance in the context of current energy solutions.
Charge and Discharge Cycles
Charge and discharge cycles represent the fundamental operational behavior of LFP cells. During the charging phase, lithium ions move from the cathode to the anode, stored within the porous structure of the anode material. It is essential to maintain an efficient electrolyte interface to facilitate this transfer. The reverse occurs during the discharge process, where lithium ions travel back to the cathode, delivering stored energy to the output. The efficiency and longevity of these cycles will determine the performance of the battery.
Often, LFP cells exhibit a longer cycle life compared to other lithium-ion batteries. This characteristic is particularly beneficial for applications requiring repeated charging, such as in electric vehicles and energy storage systems. The stability of LFP during charge and discharge contributes to its popularity, with minimal degradation even after numerous cycles.
Energy Density Mechanisms
Energy density is an important factor in the overall performance of a battery. In LFP cells, the energy density is typically lower than that of other lithium-ion technologies, like lithium nickel cobalt aluminum oxide (NCA). The reasons for this include the specific arrangement of iron phosphate in the crystal lattice, which can provide lower energy capacity. However, the energy density mechanisms of LFP can still support numerous applications effectively, especially where safety and longevity are prioritized over sheer energy output.
Innovative methods are being explored to enhance the energy density of LFP cells. Researchers are investigating advanced material combinations and structural refinements. These improvements aim to offer better performance without compromising the benefits that LFP cells provide, like stability and environmental impact.
Safety and Thermal Stability
Safety is a critical consideration in battery technology. LFP cells hold a significant advantage in this area due to their inherent thermal stability. The risk of thermal runaway is considerably lower in LFP cells as compared to other lithium-ion chemistries. This safety profile is attributed to the stronger ionic bonds within the LFP structure, which prevents decomposition even at elevated temperatures.
"The robust thermal stability of LFP cells creates a safer operational environment, which is particularly important in applications like electric vehicles."
LFP cells can tolerate higher temperatures without releasing volatile gases. This quality enables safe use in various environments, minimizing risks associated with battery failure during intense operational conditions. Understanding these safety features is essential for industries looking to implement LFP technology, ensuring that they can meet both performance and regulatory standards.
In summary, the operating mechanisms of LFP cells define their functionality and reliability across applications. Analyzing their charge and discharge cycles reveals how energy is stored and utilized. Evaluating energy density mechanisms highlights potential areas of innovation, while considering safety and thermal stability enhances their appeal in the market. Each of these elements contributes to a broader understanding of LFP technology.
Advantages of LFP Cells
The advantages of Lithium Iron Phosphate (LFP) cells are significant in the realm of energy storage and usage. Understanding these benefits is crucial for students, researchers, and professionals alike, as it directly relates to the growing demand for efficient and sustainable energy sources. These advantages contribute effectively to their adoption in various applications, emphasizing their role in future energy solutions.
Longevity and Cycle Life
One of the standout features of LFP cells is their impressive longevity and cycle life. Unlike other lithium-ion battery technologies, LFP cells can endure a higher number of charge and discharge cycles. Typical lithium-ion cells may begin to lose capacity after 500-1000 cycles, while LFP cells can last up to 2000 cycles or more, depending on usage and maintenance. This extended lifespan means lower replacement costs over time. It is essential for applications such as electric vehicles and stationary storage systems, where battery longevity translates to reduced operational costs and higher reliability.
Environmental Impact
When comparing battery technologies, the environmental impact is one area of concern. LFP cells are often considered more environmentally friendly than their counterparts. They do not contain cobalt, which raises ethical and environmental issues due to mining practices. Additionally, iron and phosphate used in LFP batteries are more abundant and less harmful to the ecosystem. Their ability to withstand harsher conditions also reduces the frequency of replacements, contributing to lower waste. In essence, choosing LFP cells aligns with a sustainable approach to energy, offering a responsible alternative to conventional batteries.

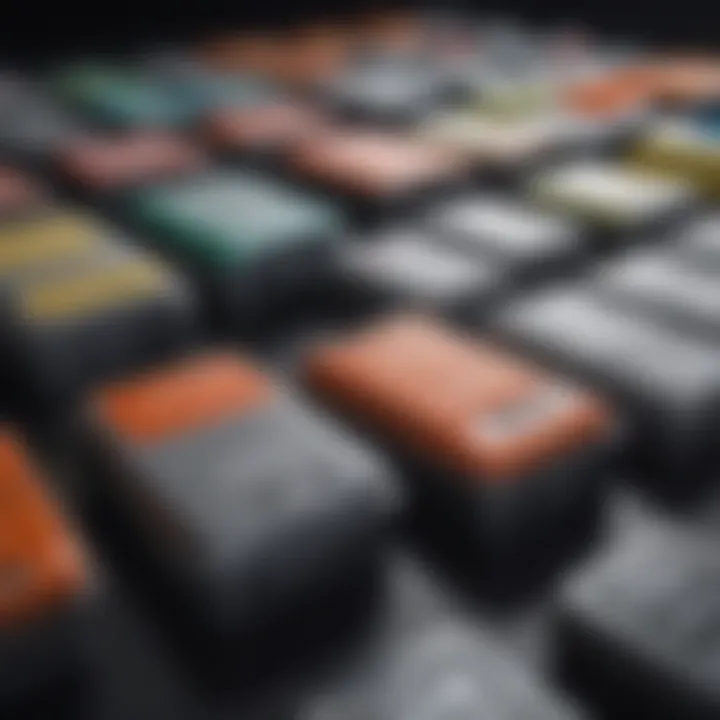
Cost-Effectiveness
Cost is a significant factor in the selection of battery technology. LFP cells are generally more cost-effective in terms of raw material availability and manufacturing processes. The prices of lithium, cobalt, and nickel can fluctuate based on market dynamics, but iron and phosphate are more stable and cheaper. This stability leads to a lower overall cost for LFP batteries. Moreover, their longer life cycle means fewer replacements, further conserving resources and reducing costs in the long-term.
Safety Profile Compared to Other Lithium-Ion Batteries
Safety is paramount in battery technology, and LFP cells shine in this regard. They have a stable thermal profile, meaning they are less prone to overheating and thermal runaway compared to other lithium-ion options. This characteristic makes them suitable for applications in environments where safety is critical, such as residential energy storage systems or electric vehicles. Their enhanced safety features enable manufacturers to design systems with fewer safety measures, ultimately lowering overall development costs.
In summary, the advantages of LFP cells include remarkable longevity and cycle life, environmental benefits, cost-effectiveness, and superior safety. These advantages support the case for more widespread adoption in various applications, including electric vehicles and energy storage systems.
Limitations of LFP Cells
Understanding the limitations of Lithium Iron Phosphate (LFP) cells is crucial for several reasons. While these cells offer various advantages, recognizing their constraints helps researchers and industry professionals make informed decisions. Addressing these limitations can enhance the overall design and application of LFP technology, ensuring it meets specific energy demands.
Energy Density Constraints
LFP cells are often critiqued for their lower energy density compared to other lithium-ion battery chemistries, such as lithium nickel manganese cobalt oxide (NMC) or lithium cobalt oxide (LCO). Energy density refers to the amount of energy stored per unit of mass. Generally, higher energy densities are desirable for applications requiring longer range or higher power in a compact space, such as electric vehicles (EVs). LFP cells typically offer an energy density of about 90-160 Wh/kg, which is lower in contrast to NMC's 150-250 Wh/kg range.
This limitation means that devices, particularly those in the EV market, might require larger battery packs to achieve similar performance levels. Furthermore, the lower energy density can lead to increased weight and volume, affecting the overall efficiency and design of the vehicle.
Temperature Sensitivity
Temperature ranges significantly impact the performance and longevity of LFP cells. Although they are generally more stable than other lithium-ion chemistries in high-temperature conditions, the efficiency of LFP cells can degrade at extreme low temperatures. At temperatures below freezing, the internal resistance within the cell can increase, leading to reduced charge acceptance and available capacity. This characteristic may hamper the effectiveness in colder climates, posing a challenge for specific applications such as outdoor energy storage systems or electric vehicles operating in winter conditions.
It becomes essential to consider thermal management strategies to address this limitation. Battery heating systems might be implemented, though they add complexity and can influence overall efficiency and cost.
Electrical Conductivity Issues
Another noteworthy limitation of LFP cells is their relatively lower electrical conductivity. The conductivity of the cathode material, in this case, lithium iron phosphate, is not as high as that of alternatives like nickel-cobalt-manganese oxides. This property can lead to increased charge/discharge resistance, impacting the power output during high-demand situations.
In practical terms, this means that LFP cells may not perform as well in applications requiring rapid discharge rates or high power bursts, such as in high-performance electric vehicles or certain renewable energy storage solutions. To cope with this, manufacturers often add conductive agents, such as carbon black, to the electrode material, enhancing conductivity but also adding to production costs.
In summary, while LFP cells offer stability and safety, their limitations in energy density, temperature sensitivity, and electrical conductivity are critical aspects for further research and development. Addressing these issues can unlock more potentials within the growing landscape of renewable energy and electric mobility.
Applications of LFP Cells
The significance of Lithium Iron Phosphate (LFP) cells extends beyond their chemical composition and operating principles. Their versatility in various applications illustrates their growing importance in addressing contemporary energy needs. This section delves into the diverse usage of LFP cells in electric vehicles, energy storage systems, industrial applications, and their potential for integration with renewable energy sources. By understanding these applications, stakeholders can better assess the strengths and limitations of LFP technology in today's energy landscape.
Usage in Electric Vehicles
Electric vehicles (EVs) are a prominent field for the application of LFP cells. The stable chemistry and enhanced longevity of LFP make it particularly well-suited for automotive use. A key feature is the longer cycle life, often exceeding 2000 cycles, which is beneficial for minimizing battery replacements. LFP batteries also exhibit a high level of safety, meaning they are less prone to thermal runaway, a significant safety concern in battery technology.
For manufacturers like Tesla, LFP cells provide a balanced performance, especially for lower-cost models where energy density is less critical. Additionally, the ability to operate well in higher temperatures makes LFP a favorable choice in various climatic conditions.
Energy Storage Systems
Energy storage systems benefit greatly from LFP cells due to their stability and long lifespan. They are used in applications from residential solar energy storage to large-scale grid support. Their capability to handle deep discharge cycles and maintain efficiency over time can lead to enhanced reliability in storing energy generated from renewable sources like solar and wind.
In many cases, LFP cells enable users to optimize their energy usage while contributing to grid stability. As energy demands fluctuate, having robust storage solutions can effectively bridge gaps and ensure continuous energy supply. This adaptability makes LFP a strong candidate in the expansion of smart grid technologies.
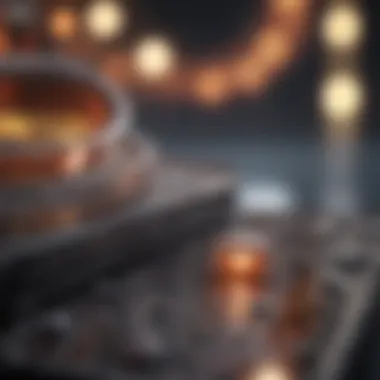
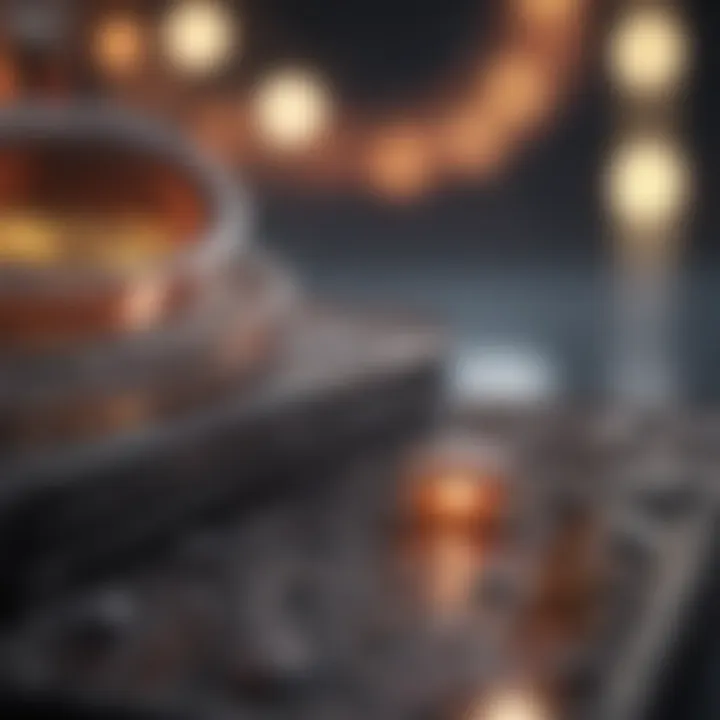
Industrial Applications
Various industries are beginning to embrace LFP cells for their efficient and safe energy supply. In sectors such as telecommunications and backup power systems, these cells help manage critical operations. Here, the reliability and longevity of LFP batteries ensure that essential services can continue uninterrupted.
Additionally, in sectors like mining and construction, LFP batteries power electric machinery, promoting a shift towards more eco-friendly equipment. This move not only enhances productivity but also aligns with global efforts to reduce carbon emissions.
Potential for Renewable Energy Integration
The role of LFP cells in renewable energy integration is noteworthy. Their stable performance makes them well-suited for storing surplus energy produced by wind or solar installations. LFP cells can discharge energy during peak demand times when energy prices are higher, thus providing economic benefits.
Furthermore, as more countries strive to meet renewable energy targets, these cells’ ability to efficiently store and release energy helps mitigate the intermittent nature of renewables. This capability is crucial for creating a dependable energy supply in the transition to a sustainable future.
LFP technology not only contributes to current energy needs but also plays a pivotal role in shaping the future of sustainable energy.
In summary, the applications of LFP cells span a broad range of industries and energy sectors. Their ability to provide stable, safe, and efficient energy solutions makes them an asset in today’s energy landscape. Understanding these applications informs further research and development efforts, ensuring that advancements in LFP technology align with global energy goals.
Market Trends and Future Directions
The landscape of Lithium Iron Phosphate (LFP) cells is rapidly changing, driven by technological advancements and increasing demand in various sectors. Understanding market trends and future directions is critical for stakeholders to navigate the complexities of this evolving industry. Observing these trends enables researchers, manufacturers, and policymakers to anticipate shifts in demand, adapt to regulatory changes, and innovate effectively.
Current Market Landscape for LFP Cells
LFP cells have established themselves as a significant player in the energy storage market. These batteries are favored for their balance between performance and safety. The current market landscape shows that electric vehicles (EVs) are the primary drivers of LFP cell demand. Several automakers, including Tesla and BYD, have adopted LFP batteries for their lower cost and enhanced thermal stability, making them suitable for mass production.
Several market reports indicate that the global LFP battery market is expected to grow at a compound annual growth rate (CAGR) of over 20% in the coming years. The current demand is also bolstered by the shift towards renewable energy systems, which require reliable energy storage solutions. Furthermore, countries are increasingly investing in charging infrastructure, further fueling the need for efficient energy storage that LFP batteries can provide.
Technological Innovations
Technological innovations are playing a vital role in improving the efficiency and performance of LFP cells. Researchers focus on enhancing the electrode materials and designs to increase energy density without compromising safety. Innovations such as the incorporation of carbon-based materials alongside the traditional iron phosphate have shown promise in improving conductivity and charge acceptance.
Battery management systems (BMS) have also evolved significantly. Advanced BMS technology optimizes the performance of LFP cells by monitoring temperature, voltage, and state of charge in real-time. This advancement helps to extend the lifespan of batteries and ensure reliability, which is crucial for applications in sectors like electric vehicles and grid storage.
"Continued investment in research and development is essential to address the limitations of LFP technology and expand its applicability across different sectors."
Regulatory and Policy Influences
Regulatory and policy influences are shaping the future of LFP cells. As environmental concerns grow, governments are implementing stricter regulations and incentive programs aimed at reducing carbon emissions. These regulations encourage the use of cleaner battery technologies, consequently favoring LFP over other chemistries that are perceived as more hazardous to the environment.
Policies promoting electric vehicle adoption and renewable energy installations are also driving demand for LFP cells. Governments across the globe are setting ambitious targets for electric vehicle sales. For instance, the European Union aims for at least 30 million electric vehicles on the road by 2030. Such clear targets create a stable demand environment for LFP batteries, encouraging manufacturers to invest in production capacity.
Finale
In synthesizing the insights gathered from this exploration of Lithium Iron Phosphate (LFP) cells, it is essential to acknowledge their intricate design and remarkable utility. The importance of understanding LFP technology lies not only in its current market standing but also in its potential growth as energy demands evolve. LFP cells present a well-balanced profile of advantages, which include safety, cost-effectiveness, and substantial cycle life.
Summary of Key Findings
Key findings from the examination of LFP cells highlight several crucial aspects:
- Chemical Structure: The unique chemical composition of LFP cells, primarily involving lithium iron phosphate as a cathode material, leads to their enhanced thermal stability and safety compared to conventional lithium-ion batteries.
- Market Relevance: Currently, LFP cells are increasingly utilized in electric vehicles and energy storage systems due to their reliability and lifespan. This trend indicates a shift towards safer and more sustainable energy solutions.
- Technological Innovations: Research advancements have concentrated on enhancing the energy density and conductivity of LFPs, addressing some of their limitations.
Implications for Future Research
The prospect for future research in LFP technology is substantial and multifaceted. Several areas merit further exploration:
- Improving Energy Density: Future studies could focus on innovative methods to enhance energy density without compromising safety. This is critical as market demands for higher-performance batteries continue to escalate.
- Regional Adoption: Comparative research into the geographical applicability of LFP technology can provide insights on how different regions can effectively adopt this technology, maximizing its benefits based on local energy needs.
- Recycling and Sustainability: As the interest in sustainable technologies grows, examining the recycling of LFP cells could yield insights into minimizing environmental impact and improving lifecycle assessments.
Overall, LFP cells represent a significant and versatile component of the future energy landscape. Monitoring advancements in this field will not only inform industry practices but also guide regulatory policies aimed at promoting cleaner energy solutions.