Understanding Light Lattice: Theoretical Foundations and Applications

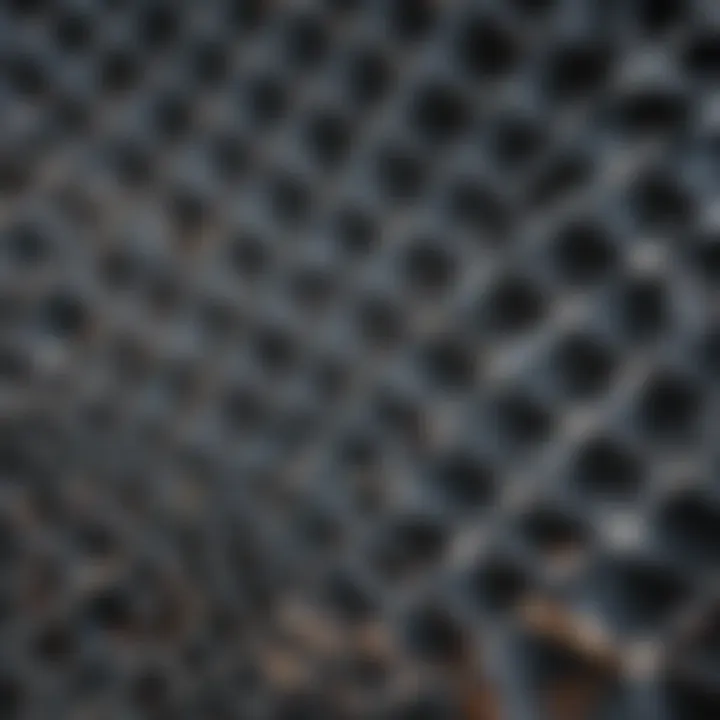
Intro
The phenomenon of light is something that has intrigued scientists and philosophers for centuries. It plays a crucial role in various fields, from optics to material science. One aspect of light that has gained traction in recent years is the concept of light lattice. This concept refers to a structured arrangement of light that can manipulate light waves in intricate ways, with far-reaching implications for technology and research.
Light lattice essentially refers to a spatial arrangement where light waves are organized in a regular pattern, much like a crystal lattice does for atoms. This structured control of light allows researchers to explore new domains of photonics and optics. The beauty of light lattice lies in its versatility, facilitating advancements in imaging techniques, communication systems, and even quantum computing.
As we delve into this topic, we will explore foundational theories, real-world applications, and potential future developments, painting a broad picture that appeals to students, educators, and researchers alike. Understanding light lattice sets the groundwork for various exciting explorations in the scientific community.
Research Highlights
Overview of Key Findings
The exploration of light lattice has brought several key findings to light:
- Control Over Light Behavior: Structuring light waves allows for precise manipulation, enhancing applications in optical communication and imaging.
- Interdisciplinary Impact: Light lattice bridges disciplines such as physics, engineering, and material science, fostering collaborative research initiatives.
- Quantum Applications: There are promising developments aimed at utilizing light lattices for advancements in quantum bits, potentially revolutionizing quantum computing.
These highlights showcase the multifaceted nature of the light lattice concept, solidifying its significance across various scientific fields.
Significance of the Research
The significance of researching light lattices cannot be overstated. As technology continues to evolve, the demand for innovative solutions is ever-growing. By harnessing the potential of light lattice:
- Researchers can innovate more efficient communication systems, paving the path for faster data transfer and improved networks.
- In medical imaging, advancements enabled by light lattice can lead to clearer images and enhanced diagnostic capabilities.
- Understanding light behavior at the lattice level can unlock further insights into fundamental physics, broadening our comprehension of the universe.
Such research not only aims to enhance technology but also addresses global challenges in communication and healthcare, making it an area ripe for exploration.
Original Research Articles
Summary of the Article
The research into light lattice often focuses on specific methodologies and applications. Recent studies have explored areas such as:
- Theoretical frameworks that define the structure of light lattices.
- Experimental setups that enable observation and manipulation of light at various wavelengths.
- Applications in emerging technologies that depend on high-precision light manipulation.
Author Contributions
Contributions to the field of light lattice research come from a diverse group of scientists and researchers:
- Physicists emphasize theoretical work and modeling to predict behaviors of light within lattices.
- Engineers develop practical applications and systems integrating light lattices into existing technologies.
- Material scientists study the properties of materials that can enhance or inhibit the effectiveness of light structures.
Together, these contributions create a comprehensive body of work that continually advances our understanding of light and its manifold applications.
Intro to Light Lattice
Light has been a pivotal subject of inquiry for centuries, touching on both the physical realm and philosophical debates. In exploring light lattice, we delve into a specialized framework that not only enhances our understanding of light behavior but also opens pathways to innovative applications in multiple scientific fields. This section will provide insights into why light lattice deserves attention and how it relates to our broader comprehension of light.
Definition and Overview
The concept of light lattice refers to a structured arrangement of light waves that create specific interference patterns. It can be thought of as a sort of architectural framework built from light itself. Lattices are typically formed through the interaction of multiple light sources or via modulations in light's path, leading to intricate designs that can be manipulated for various uses. This opens up discussions regarding the profound implications that light lattice can have, ranging from communication technologies to advanced imaging techniques.
Historical Context
Early Developments
The study of light began in earnest with scholars like Isaac Newton and Thomas Young. Newton's experiments laid the groundwork for understanding light as a particle, while Young's double-slit experiment illuminated the wave nature of light. These early ventures into the mechanics of light contributed significantly to the foundational knowledge that would eventually support the idea of light lattice.
The key characteristic of these early developments is their dual approach to understanding light, which presents a balanced view that is vital for the concept of light lattice. By blending particle and wave theories, researchers were able to expand on how light interacts with different mediums. Notably, the simplicity of Young's experimental setup—using slits to demonstrate interference patterns—became foundational and continues to be a beneficial choice for modern science. One unique feature of this early development is its applicability; these principles are not confined to theoretical discussions but have practical implications in technologies we use today.
Key Figures in Light Research
Alongside Newton and Young, other scientists like Albert Einstein and James Clerk Maxwell contributed immensely to light research, shaping its trajectory like pieces of a puzzle fitting together. Einstein's theory of photons added another dimension to the understanding of light, while Maxwell's equations provided a framework for electromagnetism, crucial for grasping how light propagates.
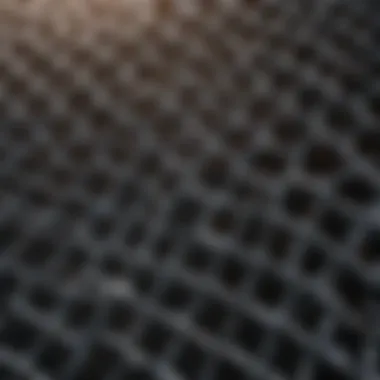
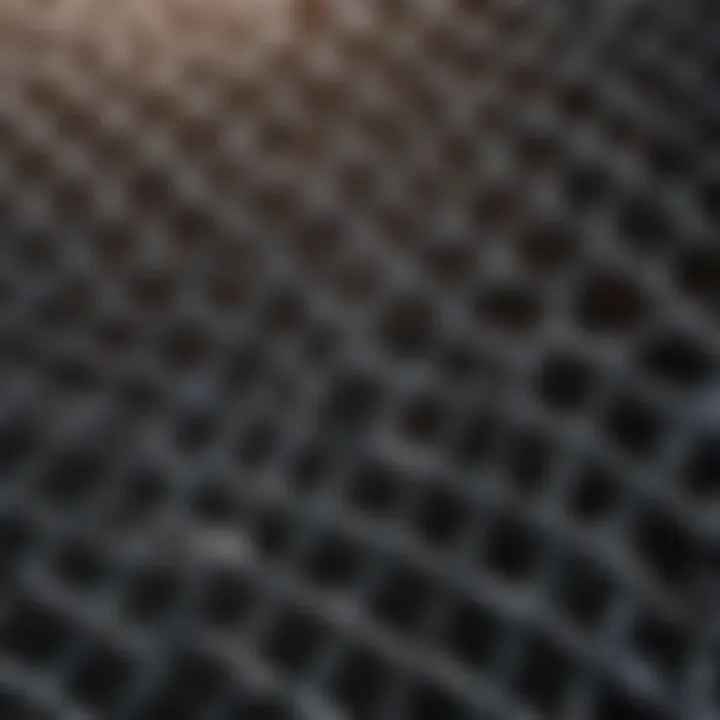
The defining aspect of these key figures is their ability to push boundaries, challenging previous understandings and introducing new ideas that intersect with the concept of light lattice. Their contributions represent a confluence of thought that serves as an inspirational benchmark for current researchers. A notable feature of their work is its interdisciplinary nature—each discovery had a ripple effect, influencing fields beyond just optics, from chemistry to engineering. However, the challenge lies in reconciling these diverse theories, each with its own merits and disadvantages, to build a cohesive understanding that can foster advancements in light lattice research.
"The interplay between theory and practical application, as seen in light research, exemplifies the essence of scientific progression."
In summary, investigating the light lattice begins with a sturdy foundation built from historical context and pivotal figures. Understanding these early developments and contributions shows how we arrived at our current comprehension of light, setting the stage for further exploration into its multifaceted applications.
Theoretical Foundations
Understanding the theoretical foundations of light lattice is crucial in grasping how light behaves in various environments. This section delves into the key elements that form the backbone of light lattice theory, addressing basic principles of light and the nuances of lattice formation within this context. By exploring these fundamentals, readers will appreciate the intricacies of how light lattices are not only formed but also manipulated in practical applications. This comprehension opens doors to innovations across multiple scientific disciplines - from optics to material science.
Basic Principles of Light
Wave-Particle Duality
Wave-particle duality stands as a cornerstone in the study of light, embodying the notion that light displays properties of both waves and particles. This duality is not just an abstract concept; it has real implications for understanding the formation of light lattices. A key characteristic of wave-particle duality is its ability to explain phenomena like interference patterns, which are pivotal for the construction of light lattices. This makes it a popular choice for guiding our discussions in this article.
The unique feature here is that light can behave as a continuous wave while simultaneously exhibiting particle-like characteristics in certain experiments, such as the photoelectric effect. This leads to insightful advantages, particularly in developing technologies that leverage both aspects of light. However, one must also consider the challenges posed by this duality; it can complicate theoretical models and lead to interpretational disagreements. Understanding this balance is vital for anyone looking to delve into the realm of light lattices.
Electromagnetic Theory
Electromagnetic theory offers another crucial perspective, bolstering our understanding of light as an electromagnetic wave. This theory demonstrates how electric and magnetic fields oscillate perpendicularly to each other and to the direction of wave propagation. One of its key characteristics is the formulation of Maxwell's equations, which govern the behavior of these fields. This theoretical foundation is a beneficial choice for our exploration because it provides the mathematical backbone needed to describe various light-related phenomena.
A unique feature of electromagnetic theory is its application in describing light’s propagation through different media, highlighting how variations in properties like refractive index lead to lattice formation. While the principles laid down by this theory have undeniable advantages in applications, such as fiber optics and laser technology, there are also disadvantages. The complexity involved in understanding and applying these concepts can be daunting, particularly in experimental setups involving light lattices.
Lattice Formation in Light
Photon Interference
Photon interference plays a pivotal role when discussing the formation of light lattices. This phenomenon occurs when two or more light waves overlap, leading to a resultant wave with varying amplitudes. A key characteristic here is constructive and destructive interference, which can enhance or diminish light intensity, respectively. This feature is instrumental, as it allows for the creation of intricate patterns—a hallmark of light lattices.
The unique facet of photon interference lies in its ability to be manipulated for various applications, such as holography or interferometry. These applications demonstrate its usefulness in both theoretical exploration and practical innovations. However, the challenges involved, like achieving precise control over interference conditions, can complicate experiments and applications, demanding rigorous calibration processes.
Wavefront Division
Wavefront division is another critical aspect in shaping how light lattices form. This principle focuses on splitting a wavefront, leading to the formation of separate paths for light. One of its key traits is the ability to create distinct interference patterns, which are foundational for applications like diffraction gratings. This characteristic makes it a beneficial choice for our exploration, especially in contexts that require precise control over light behavior.
The unique feature of wavefront division is its straightforward implementation using beam splitters and similar devices, which allows for the diverse manipulation of light pathways to create structured light patterns. Despite its advantages, weak points exist, such as the reliance on high-quality optical components to achieve desired results. This requirement can pose frustrations in experimentation and wider implementation, again emphasizing the technical challenges faced by researchers.
"The nexus of wave-particle duality, electromagnetic theory, photon interference, and wavefront division creates a rich tapestry that defines the behavior and application of light lattices throughout the scientific landscape."
Each of these foundational elements we’ve discussed contributes significantly to understanding and utilizing light lattices, paving the way for advancements in optical technologies, material sciences, and beyond.
Applications of Light Lattice
The applications of light lattice are as diverse as the interplay between light and matter itself. This realm is significant in understanding how light can be manipulated to serve various purposes across multiple disciplines. From enhancing optical devices to innovating material sciences, the applications of light lattice represent not just technological advances but also profound impacts on our daily lives and future endeavors.
In Optical Devices
Lasers
Lasers are a quintessential example of light lattice application. The way lasers produce coherent light makes them an invaluable tool in numerous fields such as telecommunications, medicine, and manufacturing. The key characteristic of lasers is their ability to generate light waves that are in sync, creating an intense focus that can be controlled with precision.
What sets lasers apart is their unique feature of producing an extremely narrow beam of light, which can travel long distances without spreading much. This characteristic is not only beneficial in applications like laser cutting, where precision is crucial, but it also enables powerful technologies such as fiber optic communication systems. However, one downside is that lasers can sometimes be expensive to produce and maintain, limiting accessibility for smaller entities. In the bigger picture, the advancements in laser technology continue to revolutionize fields and foster new possibilities.
Fiber Optics
Fiber optics represent another ground-breaking application of light lattice principles. This technology utilizes glass or plastic fibers to transmit light signals over long distances. The key characteristic of fiber optics is their ability to carry vast amounts of data efficiently and at incredible speeds. Due to their flexibility and lightweight nature, these optical fibers are vital for modern telecommunications and internet connectivity.
One notable unique feature of fiber optics is their resistance to electromagnetic interference. This property enhances data integrity and allows for clearer signals compared to traditional metallic cables. While fiber optics offer many advantages, such as high bandwidth, the installation costs can be significant. However, as the demand for high-speed internet continues to grow, fiber optic technology is regarded as essential for future-proofing communication infrastructures.
In Material Sciences
Photonic Crystals
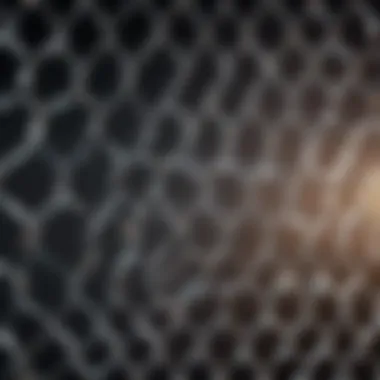
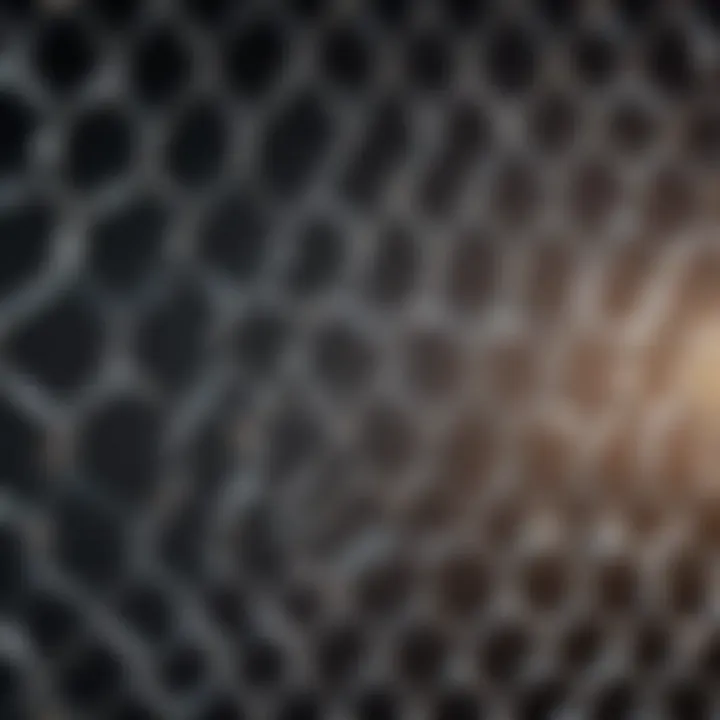
Photonic crystals offer intriguing possibilities in the realm of material sciences, acting like semiconductors for light. These structures are designed to control the flow of light in a similar manner to how electronic crystals control electron flow. A key characteristic of photonic crystals is their periodic structure, allowing them to create photonic band gaps, which prohibit certain wavelengths of light. Consequently, they can be tailored for specific applications in optical filtering and sensing technologies.
The unique feature of photonic crystals is their potential to manipulate light in ways that conventional materials cannot. This makes them particularly effective in developing advanced optical devices, yet manufacturing such precise structures can be challenging and costly. Despite the obstacles, the ongoing research in this area hints at breakthroughs in communication, imaging, and even quantum computing.
Metamaterials
Metamaterials represent a fascinating intersection of light lattice application and material science. These engineered materials exhibit properties not found in nature, primarily influencing electromagnetic waves. A key characteristic of metamaterials is their negative refractive index, allowing for the bending of light in unconventional ways. This property opens doors to applications like superlenses, which could surpass the diffraction limit of traditional lenses.
The unique feature of metamaterials is their capability to cloak objects or create illusions of invisibility. Such concepts seem pulled straight from science fiction, but researchers are working diligently on applications that could reshape how we perceive our environment. Nevertheless, these materials often require complex fabrication techniques, which can limit their commercial use. Undeniably, metamaterials present possibilities that may redefine the landscape of optics and communication.
"Light lattice applications are not just theoretical; their practical implementations are reshaping technologies and enhancing our understanding of the universe."
In summary, the applications of light lattice serve as foundations for innovation across sectors. The optical devices and material sciences showcase how manipulating light can lead to advancements that permeate every aspect of our lives, paving the way for future developments.
Interdisciplinary Perspectives
The concept of light lattice intertwines several fields, showcasing its vast reach across various disciplines. The exploration of light lattice isn't just confined to theoretical physics; it has ripple effects through biology, earth sciences, and beyond. This intersectionality enhances understanding and fosters innovation, making a compelling case for the value of interdisciplinary approaches.
Light Lattice in Biology
Biophotonics, a burgeoning field, leverages the properties of light to create transformative applications in biology. Its focus is on using light to understand biological processes. This allows scientists and researchers to explore cellular functions at an unprecedented depth, illuminating vital areas of study including imaging, diagnosis, and therapeutic methods. One key characteristic of biophotonics is its ability to enable non-invasive procedures, making it a popular choice among researchers reluctant to alter biological materials.
A notable unique feature of biophotonic applications is their reliance on specific wavelengths of light that resonate with biological molecules. These interactions enable researchers to observe processes in real time, which can be advantageous in identifying disease markers or understanding cellular interactions. However, the complexity and potential costs of implementing such technologies can be seen as disadvantages, particularly in resource-limited settings.
Imaging Techniques
Imaging techniques have evolved significantly with the advent of light lattice concepts. Modalities such as optical coherence tomography and laser scanning microscopy allow for high-resolution imaging of biological tissues. A critical aspect of imaging techniques is their precision—enabling researchers to pinpoint and study the microstructure of cells and tissues in detail.
Their popularity stems from providing clear images without the need for harmful contrast agents, thereby reducing risks associated with traditional imaging methodologies. The unique feature of these techniques lies in their capability to generate 3D representations of structures, offering a more comprehensive understanding of biological architectures. On the downside, imaging can be resource-intensive and requires sophisticated equipment, which might not always be available.
Implications in Earth Sciences
Light lattice principles also extend their influence into the realm of earth sciences. Remote sensing has emerged as a vital tool in environmental monitoring and resource management. This discipline uses light reflected from the earth's surface to gather data on everything from vegetation health to mineral composition. One of the critical characteristics of remote sensing is its ability to cover vast geographical areas quickly, making it beneficial for large-scale assessments.
Moreover, remote sensing utilizes multi-spectral and hyper-spectral imaging to capture fine details about various earth processes. The unique feature of this technology is its capacity to gather information on phenomena that would be nearly impossible to measure on-the-ground, providing a broad perspective on environmental issues. Nonetheless, the interpretation of remote sensing data can be complicated, requiring expertise to distinguish between noise and relevant signals.
Atmospheric Studies
In atmospheric sciences, employing light lattice theories can significantly enhance our understanding of climate and weather patterns. Various techniques such as lidar (light detection and ranging) are utilized to measure atmospheric components, offering insights into aerosols, clouds, and greenhouse gases. A core feature of atmospheric studies is the enhanced spatial resolution enabled by using laser light, which can penetrate through clouds and various atmospheric disturbances.
This makes it a highly effective method for monitoring air quality and weather changes. However, as with many sophisticated technologies, the technical skill required for proper data analysis presents a barrier, particularly in less developed regions.
The blend of light lattice principles across fields indicates not only the versatility of these concepts but also emphasizes the necessity of collaboration among different scientific communities.
Overall, understanding light lattice from interdisciplinary perspectives enriches both theoretical and practical realms, offering a promising outlook for future innovations and discoveries. This interplay is essential, considering the challenges faced in modern science, requiring comprehensive approaches to tackle complex problems.
Challenges in Light Lattice Research
Engaging with the concept of light lattice brings to light numerous challenges, which, while sometimes daunting, can also serve as fertile ground for innovation and advancement. Recognizing these hurdles provides a clearer understanding of the intricacies involved in light lattice research. This section breaks down the technical limitations and theoretical issues faced in the study of light lattices, elucidating their implications and the need for ongoing exploration in the field.
Technical Limitations
Measurement Challenges
At the core of any scientific inquiry lies the ability to measure phenomena accurately. When it comes to light lattices, the measurement challenges can pose significant obstacles. These challenges stem largely from the complexities of light behavior when subjected to various mediums. For instance, how light interacts with different materials can skew measurement results, creating a need for exceedingly precise tools and methodologies. Achieving clarity in these measurements is crucial for validating theoretical models and ensuring reproducibility of experiments, which is key in any scientific discipline.
A key characteristic of measurement challenges is the sensitivity of light to environmental factors. Changes in temperature, pressure, or even electromagnetic interference can adversely affect readings. This aspect makes rigorous training and calibration necessary for instruments measuring light lattice phenomena.
Though measurement challenges may initially appear as mere hurdles, they offer the unique opportunity for researchers to pioneer new techniques and technologies. Addressing these hurdles not only enhances measurement accuracy but also merges disciplines like engineering and physical sciences.
Material Constraints
The material constraints associated with light lattice research also play a pivotal role. The materials that are typically utilized in constructing light lattices—such as photonic crystals and metamaterials—have inherent limitations, particularly regarding their structural integrity and optical properties. A noteworthy feature of these materials is their dependence on precise fabrication techniques. Imperfections can result in unintended scattering and absorption, which complicate the overall design and functionality of light lattices.
The intricate relationship between light and material also brings forth peculiar challenges. For example, while certain materials may effectively manipulate light at specific wavelengths, they can fail to perform at others. Thus, researchers must remain flexible and innovative, often experimenting with combinations of materials to optimize performance.
Despite the potential complications posed by material constraints, the investigation into new materials continues to unveil avenues for enhancing light lattice applications. As researchers push the boundaries of materials science, new discoveries may lead to breakthroughs that elevate the capabilities of light lattices.
Theoretical Issues
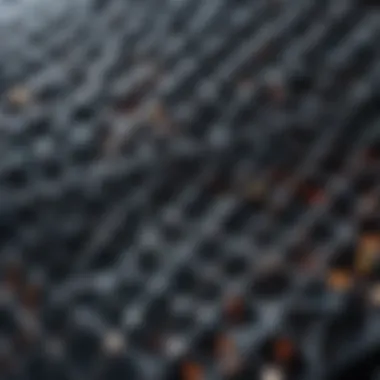
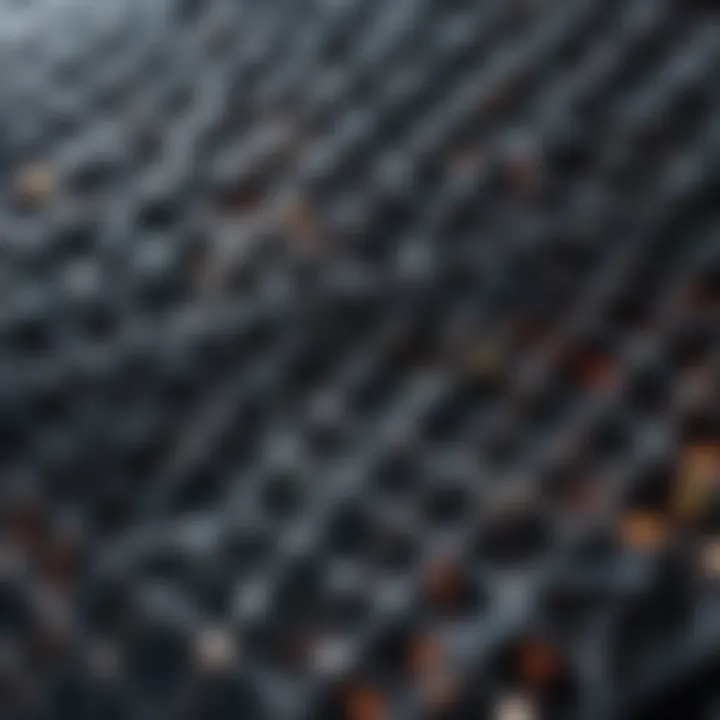
Interpretational Disparities
In any scientific field, interpretational disparities arise as a natural consequence of new findings and developing theories. In the context of light lattice research, these disparities can shape the progress of the discipline. Different interpretations of experimental data can lead to varied conclusions about the behavior and manipulation of light, creating divisions in the scientific community. For example, in some studies, findings may suggest a strong correlation between light lattice structure and performance, while others might downplay this connection.
An important characteristic of these disparities lies in the diversity of perspectives that researchers bring to the table. While variability can enrich discourse, it can also result in confusion and miscommunication. Recognizing and addressing these interpretational differences is beneficial for fostering collaboration, allowing researchers to build upon one another’s findings rather than contest them.
Modeling Complexities
Lastly, modeling complexities present another significant barrier in light lattice research. Theoretical models are essential for predicting behavior and validating experimental results. However, accurately simulating the behavior of light within a lattice is a daunting task, often involving intricate mathematical frameworks that can become unwieldy. The unique feature of these complexities is that they require a robust understanding of both light physics and computational methods, which can be a steep learning curve for newcomers.
Yet, these modeling complexities also serve as a driving force for advancements in computational optics. As technology progresses and new algorithms are developed, researchers can refine models, improving their accuracy and predictive capabilities. The interplay between theory and application will thus continue to shape the field of light lattices, leading to potential breakthroughs.
In essence, while challenges in light lattice research may seem intimidating, they provide essential context for continuous inquiry and the quest for understanding. Tackling these issues can potentially result in discoveries that shift existing paradigms in science.
Future Directions
Exploring the future directions of light lattice research is not just an academic endeavor but also a necessary step toward understanding how we interact with the world through light. As technology progresses, this field is poised to influence everything from information processing to environmental sustainability. Comprehending these future aspects sheds light on possibilities that can emerge from this fascinating area of study.
Emerging Technologies
Quantum Computing
Quantum computing represents a revolution in processing power. This technology leverages the principles of quantum mechanics to perform calculations at speeds unattainable by classical computers. In the context of light lattice, quantum computing can enhance our ability to model complex photonic systems. A key characteristic here is the concept of qubits, which can exist in multiple states simultaneously, allowing for parallel processing.
One unique feature of quantum computing lies in its potential to solve multifaceted problems through entanglement, which significantly boosts computational efficiency. However, the disadvantages include the current technological limitations and the complex nature of its implementation, making it less accessible for widespread research on light lattices. Despite this challenge, the benefits of integrating such quantum technologies hold promise for advancing understanding in light manipulation.
Integrated Photonics
Integrated photonics is another emerging technology packed with potential. This area focuses on the integration of various photonic functions onto a single chip, combining functionalities like generation, detection, and manipulation of light. It plays a crucial role in improving the efficiency of optical devices, which is pivotal for the applications of light lattice.
The key characteristic of integrated photonics is its compactness, which allows for scaling down devices while maintaining high performance. This unique feature offers advantages such as reduced energy consumption and increased speed of data transmission. However, challenges arise in terms of manufacturing techniques and the material limitations of photonic circuits, which can hamper scalability. The ongoing research in this field is critical for harnessing the full potential of light lattice technology.
Potential Societal Impact
Sustainable Practices
The societal implications of light lattice research extend into sustainable practices. Integrating sophisticated optical technologies can enhance energy efficiency in various sectors, including renewable energy. By improving energy harvesting methods—such as photovoltaic cells—the light lattice concept can play an instrumental role in driving sustainable technological advancements.
A key feature of sustainable practices in this context is their ability to minimize environmental impact while maximizing efficiency. The benefits of such practices include reduced waste and lower carbon footprints. However, the challenge lies in achieving large-scale adoption and overcoming the existing infrastructure barriers that might hinder the implementation of these advanced technologies.
Educational Perspectives
Finally, examining the educational perspectives tied to light lattice research reveals its role in shaping future scientists and engineers. By introducing concepts of light manipulation and photonics in educational curriculums, we foster a new generation capable of tackling forthcoming challenges related to energy and technology.
The hallmark of this educational initiative is its interdisciplinary approach. Integrating knowledge from physics, engineering, and environmental studies allows students to develop a comprehensive understanding of how light can be harnessed. The benefits extend beyond academia, as these insights prepare students for real-world applications. Yet the drawback may include the necessity of updating educational resources continuously to keep pace with rapid advancements.
"The future of light lattice research isn't just about technology; it's about improving our society's ability to interact with and sustain its environment."
In sum, the future directions of light lattice research highlight the intersection of technology, sustainability, and education. These elements are essential for navigating the complex landscape unfolding before us as we harness the power of light.
Epilogue
In this article, we have traversed the multifaceted terrains of light lattice, presenting a thorough understanding of its implications across various scientific disciplines. The importance of concluding our journey with an emphatic focus cannot be overstated. The conclusion synthesizes the primary findings and lays bare the significance of ongoing research in this vibrant field.
Summary of Findings
Our exploration showcased several key points. First and foremost, we defined light lattice and its underpinnings, guided by both historical context and theoretical foundations. We emphasized the dual nature of light, discussing its wave and particle aspects. From there, we ventured into the intricate mechanisms of lattice formation, elaborating on phenomena such as photon interference and wavefront division.
Second, the applications of light lattice were examined, particularly in optical devices like lasers, and in material sciences where photonic crystals and metamaterials take center stage. These advancements illustrate how light lattice acts as a cornerstone for developing cutting-edge technologies and enhancing existing solutions.
Lastly, we scrutinized the interdisciplinary perspectives, revealing how light lattice is vital in biology and earth sciences. Challenges and possible future directions were outlined, providing insight into where this field might lead us. The breadth of knowledge accumulated supports an understanding that transcends conventional boundaries and fosters innovative thinking.
Final Thoughts on Light Lattice Research
The light lattice concept stands out as a pivotal axis around which various scientific explorations revolve. As we’ve seen, its potential stretches far beyond mere theory, influencing practical applications and suggesting novel approaches to longstanding problems. The societal impact of advancing light lattice research cannot be ignored; it poses numerous benefits for sustainable practices, technological progression, and rich educational opportunities.
"The journey of light lattice research merges theoretical ingenuity with practical application, illuminating pathways to future discoveries."
In closing, as students, researchers, and educators engage with this dynamic field, a keen understanding of its principles will not only enrich their knowledge but illuminate the way forward in an increasingly complex world. The future of light lattice research is bright, holding promise for new frontiers that integrate science and technology in ways previously unimaginable.