Key Components of Lithium-Ion Batteries Explained
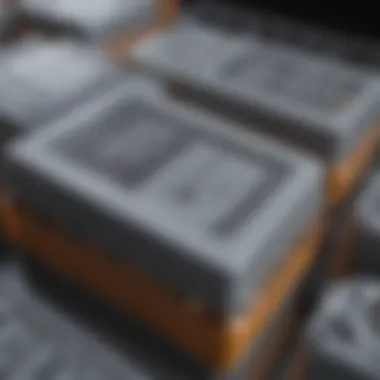
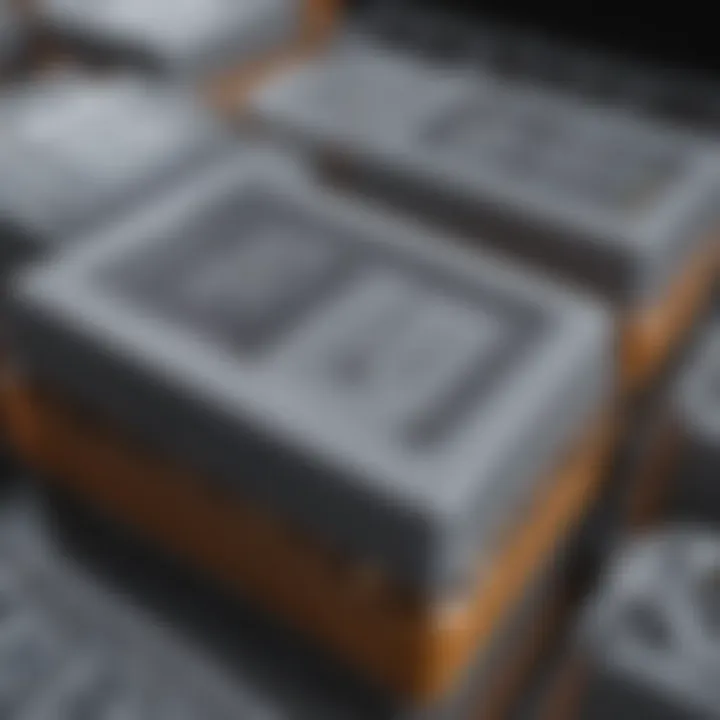
Intro
Lithium-ion batteries have become a cornerstone of modern energy solutions, powering everything from smartphones to electric vehicles. Their structure and materials play a pivotal role in determining efficiency, longevity, and overall performance. By dissecting each component, we can understand not only how these batteries function but also the advancements that continue to enhance their capabilities.
This exploration delves into the electrochemical processes that underpin the operation of lithium-ion batteries. We analyze the various materials used in their construction, detailing the importance of material choice in terms of performance and environmental impact. Ongoing research in this field presents opportunities for innovations that ensure the sustainability of lithium-ion technology.
Through this comprehensive guide, we aim to provide insights relevant to students, researchers, educators, and professionals in the field, enhancing their understanding of the critical elements that sustain modern energy applications.
Research Highlights
Overview of Key Findings
The investigation into lithium-ion batteries reveals several key findings:
- The anode and cathode materials greatly influence the energy density. Common materials include graphite for anodes and lithium metal oxides for cathodes.
- The choice of anode and cathode materials impacts not just performance but also charging times and cycle life.
- The electrolyte facilitates ion movement between the anode and cathode, and the type of electrolyte correlates with the overall battery stability and safety.
- Current research emphasizes the importance of solid-state electrolytes, which promise higher efficiency and safety compared to traditional liquid electrolytes.
Significance of the Research
The significance of this research lies in its ability to inform ongoing developments in energy storage technology. Understanding the components allows for more targeted innovations:
- Improved efficiency ensures longer-lasting batteries, which is crucial for consumer electronics and electric vehicles.
- Innovations in material science can lead to batteries that are not only more effective but also more environmentally friendly.
- As renewable energy sources expand, efficient storage systems will become indispensable, making lithium-ion technology even more pertinent.
"The evolution of lithium-ion batteries encapsulates the intersection of technology, environmental consciousness, and consumer demand."
With these insights, we will delve deeper into the specific components that constitute lithium-ion batteries, their functions, and the latest advancements in this rapidly evolving field.
Prologue to Lithium-Ion Batteries
Lithium-ion batteries are a cornerstone of modern energy solutions. They power devices from smartphones to electric vehicles. Understanding their structure and components is vital to appreciate their impact and applications. This section delves into the historical context and contemporary relevance of lithium-ion technology, which has reshaped our interaction with energy.
Historical Context and Development
The development of lithium-ion batteries began in the 1970s. Early research focused on lithium as a light and highly reactive element, suitable for energy storage. Initial prototypes were not practical. However, in the 1990s, significant advancements were made. John Goodenough, Rachid Yazami, and Akira Yoshino contributed essential discoveries. Goodenough's work led to the lithium cobalt oxide cathode, while Yazami developed a functional anode using graphite.
In 1991, Sony commercialized the first lithium-ion battery. It quickly became the preferred choice for portable electronics due to its light weight and high energy density. Over the years, ongoing research has improved performance and safety. These innovations include advanced cathode and anode materials, enhancing capacity and cycle life. As these batteries evolved, they found applications in various fields, including renewable energy storage and electric transportation.
Importance in Modern Applications
Today, lithium-ion batteries are integral to many industries. Their high energy density and efficiency make them ideal for powering electric vehicles, laptops, and personal gadgets. In renewable energy systems, they store electricity generated by solar and wind. This ability to store energy enhances grid stability and supports the transition toward sustainable energy solutions.
The versatility of lithium-ion technology also enables advancements in portable electronics. Smart devices, including smartphones and tablets, rely on these batteries for longer usage without frequent recharging.
Moreover, ongoing research focuses on improving the lifespan and sustainability of lithium-ion batteries. Innovations like solid-state batteries promise even safer and more efficient energy storage options in the future. Understanding the significance of lithium-ion batteries in today’s world is crucial for anyone interested in energy technologies and sustainability.
Fundamental Components
Understanding the fundamental components of lithium-ion batteries is crucial to grasp how these systems function. This section provides a breakdown of each main part, detailing their materials, structures, and roles. The effectiveness of a lithium-ion battery largely depends on its components, which directly influence energy storage capacity, longevity, and overall performance. By examining these elements closely, we can appreciate the advancements made in battery technology and their implications in real-world applications.
Anode Materials
The anode is one of the primary components in a lithium-ion battery, responsible for housing lithium ions during discharge and facilitating their return during charging. Various materials contribute to the efficiency and durability of the anode, each with its benefits and limitations.
Graphite
Graphite is the most widely used anode material in lithium-ion batteries. Its high electrical conductivity and stability make it popular among manufacturers. A key characteristic of graphite is its ability to intercalate lithium ions, allowing for effective charge storage. This unique feature contributes to its reliability in numerous applications, from mobile devices to electric vehicles. However, graphite's energy density is somewhat limited compared to other materials, which can be a disadvantage in pursuit of enhanced energy capacity.
Lithium Titanate
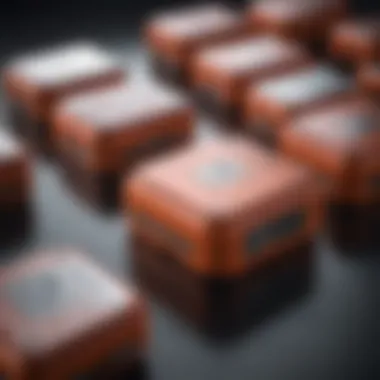
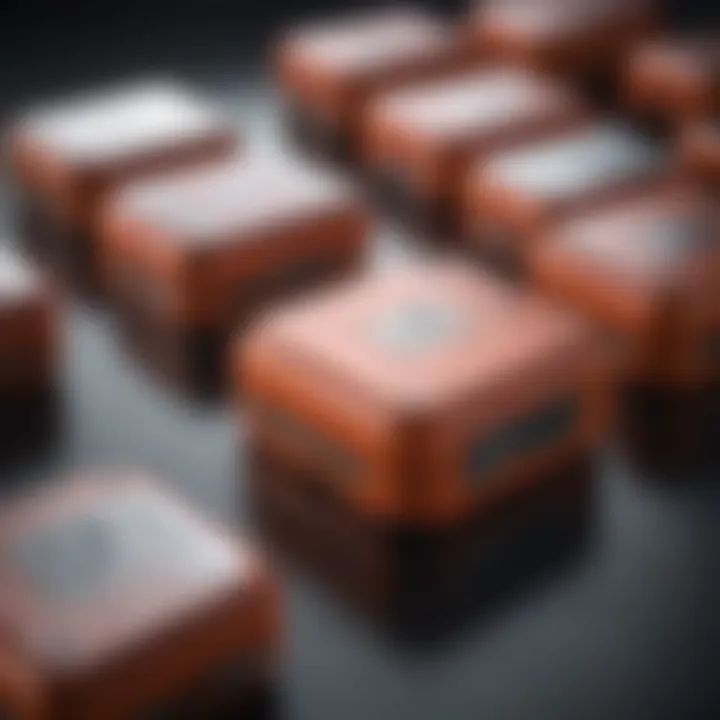
Lithium titanate presents an alternative as an anode material, praised for its quick charge capabilities and long cycle life. The key characteristic of lithium titanate is its stable structure, which allows it to endure a high number of charge cycles without significant degradation. This makes it an attractive option for applications requiring fast charging, such as some electric buses. Although it has great cycling stability, its lower energy density compared to graphite can limit its widespread adoption.
Silicon-Based Anodes
Silicon-based anodes are gaining interest due to their substantial potential to increase energy capacity. The principal advantage of silicon is its ability to absorb significant amounts of lithium, providing a four to ten times higher theoretical capacity than graphite. However, the expansion and contraction of silicon during charge cycles can lead to mechanical degradation over time, causing challenges in maintaining battery longevity. Therefore, while silicon presents opportunities for improved energy density, it also poses technical hurdles that need to be addressed.
Cathode Materials
The cathode serves as the other half of the electrode pair and plays a critical role in the battery's overall functionality. Much like the anode, the choice of cathode material directly affects the battery's efficiency, energy density, and safety.
Lithium Cobalt Oxide
Lithium cobalt oxide is a common cathode material, particularly in consumer electronics. Its main advantage lies in its high energy density, which makes it ideal for compact devices. However, pricing and the cost of cobalt can be significant disadvantages. Additionally, cobalt poses ethical sourcing challenges, raising concerns about sustainability in battery production.
Lithium Iron Phosphate
Lithium iron phosphate offers a more stable and safer alternative to lithium cobalt oxide. The key advantage of lithium iron phosphate is its thermal stability, which enhances safety features and longevity in battery applications. While it has a lower energy density than cobalt-based materials, its lower cost and greater availability make it an appealing option for electric vehicles and renewable energy systems.
NMC and NCA Compositions
NMC (Nickel Manganese Cobalt) and NCA (Nickel Cobalt Aluminum) compositions represent sophisticated cathode materials designed to balance performance and cost. NMC provides excellent energy density, stability, and lower cobalt content. On the other hand, NCA focuses on high energy density, making it suitable for applications like electric vehicles. The trade-offs between these compositions often revolve around performance, safety, and material availability, which are critical considerations for future battery development.
Electrolytes
Electrolytes play an essential role in facilitating the movement of lithium ions between the anode and cathode. They can significantly affect the performance, life span, and safety of lithium-ion batteries, so the choice of electrolyte material is fundamental.
Liquid Electrolytes
Liquid electrolytes, typically composed of lithium salts dissolved in organic solvents, are the most common. They offer high ionic conductivity, which is necessary for efficient battery operation. However, their flammability poses safety concerns when a battery is damaged or improperly handled.
Solid-State Electrolytes
Solid-state electrolytes promise enhanced safety and stability in lithium-ion batteries. Unlike their liquid counterparts, solid-state electrolytes do not present the same flammability risks. Additionally, they can improve energy density by allowing a higher voltage. The main challenges currently lie in manufacturing processes and cost-effectiveness, yet research in this area continues to grow.
Gel Polymer Electrolytes
Gel polymer electrolytes combine the properties of liquid and solid electrolytes, offering a compromise between safety and performance. A key characteristic is their ability to remain stable and flexible while presenting lower risks of leakage. Gel polymer electrolytes are still in development stages but hold promise for various applications where flexibility and safety are priorities.
Separators
Separators are another crucial component in lithium-ion batteries, positioned between the anode and cathode. Their function is to prevent short circuits while allowing lithium ions to pass through. Evaluating the materials and designs used in separators can reflect on the performance and efficiency of batteries.
Material Types
Common materials for separators include polyethylene and polypropylene. These materials have distinct properties that allow for operational efficiency in batteries. The key characteristic of these materials is their effectiveness in preventing dendrite growth, which can lead to battery failure. However, challenges such as temperature stability need to be considered when selecting separator materials.
Functionality
The functionality of separators, beyond preventing electrical contact between electrodes, also involves ensuring ionic conductivity. An effective separator not only protects the mechanical integrity of the battery but enhances overall performance. Additives and coatings are sometimes employed to improve functionality, although they may increase cost or complexity.
Improvements in Efficiency
Ongoing research focuses on enhancing separator efficiency through innovative materials and designs. Improvements can contribute to higher energy density and longer cycle life, ultimately impacting battery performance. Although advancements are promising, integrating these improvements must balance performance with manufacturing feasibility and cost-effectiveness.
Electrochemical Principles
Understanding the electrochemical principles is essential in comprehending how lithium-ion batteries operate and deliver their energy efficiently. These principles govern the interactions between the various components of the battery, influencing its performance, stability, and longevity. By parsing these interactions, one gains insights into the operation of batteries in modern devices, ranging from smartphones to electric vehicles.
Charge and Discharge Mechanism
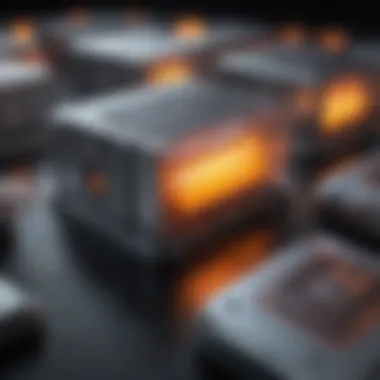
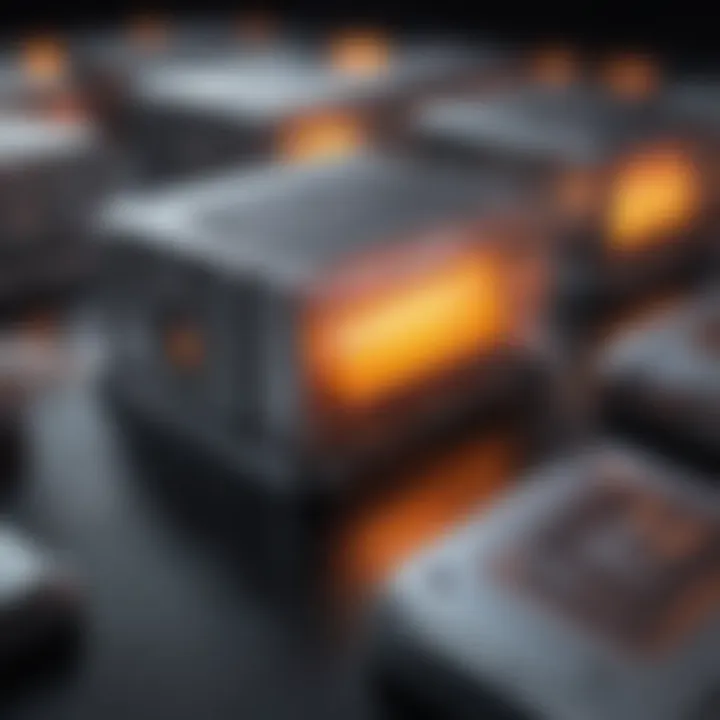
The charge and discharge mechanism in lithium-ion batteries fundamentally revolves around the movement of lithium ions between the anode and cathode. During charging, lithium ions migrate from the cathode, usually made of lithium cobalt oxide or lithium iron phosphate, through the electrolyte toward the anode, typically composed of graphite. This movement stores electrical energy in the form of chemical energy at the anode.
Conversely, during discharge, the process reverses. Lithium ions move back from the anode to the cathode, releasing the stored energy as electrical power. These movements involve oxidation and reduction reactions, where lithium ions undergo redox processes. The efficiency of this cycle is paramount, as it determines the overall performance of the battery. The optimization of these charge-discharge cycles is ongoing, to enhance efficiency and reduce degradation over time.
Energy Density vs. Power Density
Energy density and power density are critical metrics in evaluating lithium-ion batteries. Energy density refers to the amount of energy stored per unit mass or volume. High energy density is desirable for applications requiring long-lasting power without increasing size, such as electric vehicles or portable electronics. Lithium-ion batteries feature relatively high energy densities compared to other battery technologies, making them suitable for these applications.
In contrast, power density indicates how quickly energy can be delivered. A battery with high power density can provide bursts of energy rapidly, which is especially important for applications needing quick acceleration or high instantaneous power, such as in power tools or hybrid vehicles. Balancing energy and power density is essential, as enhancing one can sometimes negatively impact the other. Research is ongoing to develop materials and designs that optimize both metrics simultaneously, ensuring better efficiency in a variety of applications.
Cycle Life and Performance Metrics
Cycle life is a significant aspect of the performance of lithium-ion batteries. It reflects how many complete charge-discharge cycles a battery can undergo before its performance deteriorates to a certain percentage—usually around 80%. Factors influencing cycle life include the quality of materials used, design of the battery, temperature conditions, and depth of discharge. Advanced materials like silicon-based anodes and lithium iron phosphate can contribute to a longer cycle life by preventing rapid degradation during cycles.
Performance metrics extend beyond cycle life. They encompass charge times, efficiency during charge and discharge, thermal performance, and safety measures. A better understanding of these metrics informs users about how well a battery will perform in various conditions. Moreover, researchers concentrate on developing technologies that improve these metrics, enhancing the overall viability of lithium-ion batteries in meeting future energy needs.
Investing in research for advanced materials could significantly enhance the lifespan and performance of lithium-ion batteries.
These electrochemical principles are not simply academic; they guide practical applications and innovations that shape the future of energy storage solutions in an increasingly energy-dependent world.
Technological Innovations
Technological innovations in lithium-ion batteries play a pivotal role in enhancing performance and addressing environmental concerns. This section breaks down specific advancements that are shaping the future of energy storage systems. Focusing on materials, management systems, and development strategies, we analyze their importance and applicability.
Advancement in Materials
Nanotechnology Applications
Nanotechnology applications are revolutionizing the landscape of battery materials, contributing immensely to lithium-ion battery efficiency. These technologies enable the manipulation of materials at the nanoscale, leading to enhanced conductivity and surface area. A key characteristic of nanomaterials is their ability to improve electrode performance, allowing for faster charge and discharge rates. This is crucial for applications that demand high power output, such as electric vehicles.
The unique feature of nanotechnology lies in its potential to reduce the overall weight and size of batteries while maintaining energy density. This offers significant advantages in portable devices, where space and mass are critical constraints. However, there are challenges associated with nanomaterials, particularly in terms of production costs and scalability, which must be addressed to fully realize their potential.
Next-Generation Cathode Materials
Next-generation cathode materials are essential in increasing the energy capacity of lithium-ion batteries. Unlike traditional materials, newer options—like lithium-rich layered oxides or lithium-sulfur—promise higher theoretical energy densities. Their key characteristic is the ability to store more energy per unit weight compared to previous generations. This aspect makes them a popular choice for developers looking to maximize performance.
A major advantage of next-generation materials is their potential to improve battery lifespan. They can resist degradation better than conventional cathodes, contributing to longer-lasting energy solutions. On the downside, these newer materials may face issues during manufacturing and integration into existing production lines, necessitating careful evaluation of their economic viability.
Battery Management Systems
Temperature Regulation
Temperature regulation systems in lithium-ion batteries ensure optimal performance and safety. They monitor battery temperatures during operation, mitigating risks associated with thermal runaway, which can lead to failures or accidents. The ability to maintain a stable temperature is a critical characteristic that improves reliability.
Such systems utilize various methods, including active cooling and thermal insulation, to manage heat effectively. The unique feature of these regulatory mechanisms lies in their capability to extend battery life by preventing excessive heat buildup. On the downside, additional components can increase complexity and cost in battery design.
State of Charge Estimation
The state of charge estimation is vital for effective energy management in lithium-ion batteries. This system calculates the remaining capacity of the battery, which is crucial for ensuring efficient usage and planning for recharge cycles. Its main characteristic is accurate and timely reporting, allowing users to avoid unexpected shutdowns.
One unique aspect of state of charge estimation is the use of advanced algorithms, which can incorporate factors like temperature and aging effects to improve accuracy. This adds value by enhancing the user experience and reliability of battery-operated devices. Nevertheless, complexity in the algorithms can lead to challenges in real-time implementation.
Solid-State Battery Development
Benefits Over Conventional Batteries
Solid-state batteries present significant advantages compared to conventional lithium-ion batteries. One notable benefit is improved safety, as these designs eliminate the flammable liquid electrolyte, reducing the risk of fires. Their key characteristic is the solid electrolyte, which facilitates better ion conduction and overall battery stability.
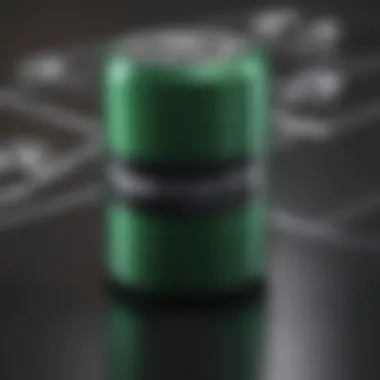
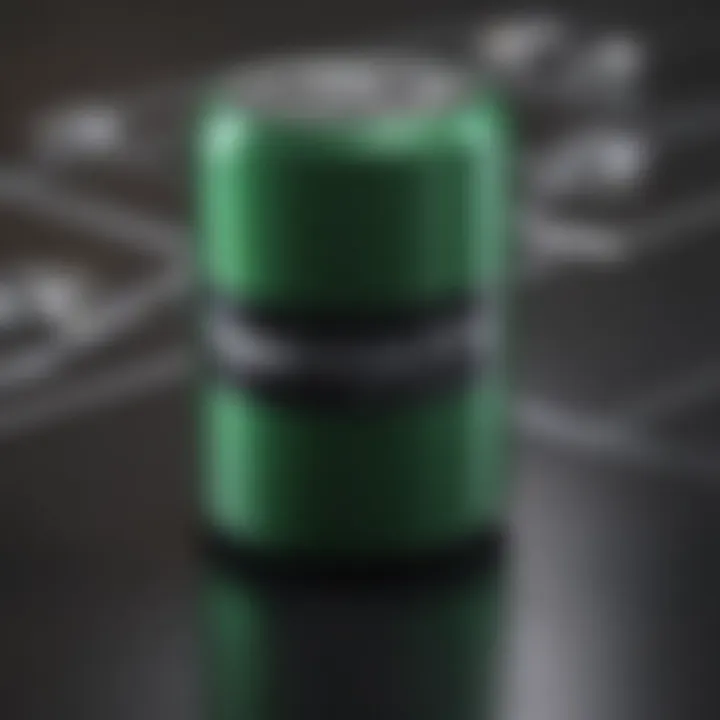
The unique feature of solid-state batteries lies in their potential for higher energy density, allowing for more compact designs. This is particularly advantageous in applications requiring lightweight materials. However, the high-cost implications of materials and production processes remain a key disadvantage, hindering widespread adoption.
Challenges in Implementation
Despite their benefits, solid-state batteries face several challenges in implementation. A major concern is the difficulty of manufacturing these batteries at scale without compromising performance. Their key characteristic includes intricate design requirements that can complicate production lines.
Unique challenges are also evident in interface stability between electrodes and solid electrolytes. This can lead to performance degradation over time. While research continues to address these obstacles, the complexity of these challenges highlights the need for ongoing innovation in the field.
Advances in technology are transforming the efficiency and safety of lithium-ion batteries, ensuring they remain a cornerstone of modern energy solutions.
Environmental Impact and Sustainability
The exploration of environmental impact and sustainability in lithium-ion batteries is crucial today. As demand for clean energy solutions increases, understanding the complete lifecycle of these batteries becomes increasingly essential. This section focuses on the environmental consequences of sourcing materials for lithium-ion batteries, as well as recycling practices that aim to lessen these impacts. Addressing sustainability entails evaluating how these batteries align with collective efforts to promote circular economies.
Material Sourcing and Recycling
Recycling Technologies
Recycling technologies represent a significant advancement in reducing the environmental footprint of lithium-ion batteries. These methods enable the recovery of valuable materials, such as cobalt, nickel, and lithium, which can be reintroduced into the production cycle. One key characteristic of these recycling technologies is their ability to minimize waste generated from discarded batteries. The closed-loop system created through effective recycling is a beneficial approach in enhancing sustainability.
Different recycling technologies, including hydrometallurgical and pyrometallurgical processes, showcase unique features. For example, hydrometallurgical processes often yield a higher recovery rate of metals, making them efficient choices for the goal of resource conservation. On the other hand, the pyrometallurgical approach is often criticized for its higher energy consumption. Thus, while recycling technologies present advantages, they also carry disadvantages or challenges that must be addressed to optimize their efficacy for the environment.
Environmental Regulations
Environmental regulations play an important role in ensuring responsible practices in battery production and disposal. These regulations establish standards for emissions and waste management, influencing how manufacturers operate. A key characteristic of these regulations is their impact on driving innovation in cleaner production methods and safe disposal practices. This makes them a popular choice among policymakers and environmental advocates.
These regulations compel companies to adopt safer practices, thereby protecting ecosystems and communities from hazardous materials often found in batteries. One unique feature is the push towards implementing extended producer responsibility legislation, which mandates manufacturers to take responsibility for the entire lifecycle of their products. While regulations provide frameworks to guide industry practices, they may also create barriers to entry for smaller firms due to compliance costs, highlighting the complexities involved in balancing sustainable progress.
Life Cycle Assessment
Life cycle assessment is a critical tool in evaluating the environmental impacts throughout the lifespan of lithium-ion batteries. This process examines resource extraction, manufacturing, usage, and disposal phases. Each phase offers insights into areas where improvements can be made.
Conducting life cycle assessments can identify the most significant ecological footprints and inform better design choices for future battery technologies. In essence, it is an invaluable process for fostering sustainable innovation in battery production. Assessing these impacts ensures that advancements do not come at the expense of our environment.
Future Trends and Research Directions
The exploration of future trends and research directions in lithium-ion batteries is crucial for understanding how advancements will shape energy solutions. Continuous innovation is necessary to address challenges in performance, sustainability, and application. Researchers and engineers are focusing on improving energy density, enhancing safety, and reducing environmental impacts. The ongoing study of new materials and technologies is vital for the evolution of these batteries.
Emerging Technologies
Hybrid Energy Storage Systems
Hybrid energy storage systems combine different storage technologies to optimize energy output and efficiency. This integration allows for the mitigation of limitations associated with individual systems. The flexibility of hybrid setups enhances overall energy management for applications ranging from grid energy storage to electric vehicles. One key characteristic is their ability to deliver quick bursts of power, which is essential for applications like regenerative braking in cars.
The benefits of hybrid systems also include redundancy and improved cycle stability. They can efficiently balance load variations and serve as backup during peak demands. However, complications can arise in the management of different technologies, which may require sophisticated control strategies.
Wireless Charging Solutions
Wireless charging solutions represent a significant shift in how energy transfer occurs, eliminating the need for physical connectors. This technology utilizes electromagnetic fields to transfer energy, making charging more convenient and encouraging frequent top-offs, especially in mobile devices and electric vehicles. The inherent convenience of wireless charging positions it as a popular choice in various sectors today.
One unique feature is the potential for dynamic charging, where vehicles can charge while moving, reducing downtime significantly. However, there are challenges in efficiency, as energy losses can occur during transmission. Ensuring effective solutions for heat management and loss reduction is critical for its successful implementation in broader applications.
Impact of Artificial Intelligence
Algorithm Development for Battery Life Optimization
The development of algorithms for optimizing battery life is essential in maximizing efficiency and longevity. These algorithms can evaluate performance in real-time, adjusting operational parameters to extend the cycle life of batteries. This characteristic makes algorithm development a focal point for improving lithium-ion technology in research and industry.
A unique aspect of these algorithms is their ability to analyze vast datasets generated during battery operation. Machine learning techniques can predict future performance based on historical data, allowing for proactive adjustments. Although this approach promises substantial benefits, it requires careful design to accommodate the complexities inherent in battery systems and their varied usage scenarios.
Predictive Maintenance
Predictive maintenance involves using data analysis and monitoring systems to assess battery health, anticipating failures before they occur. By employing this strategy, organizations can minimize downtime and reduce overall maintenance costs, making it a beneficial approach for industries relying heavily on battery technologies.
The key characteristic of predictive maintenance is its reliance on real-time data to inform decision-making, ensuring optimal battery performance. Its unique feature lies in the potential to prevent catastrophic failures, as timely interventions can save resources and enhance safety. However, the challenge lies in establishing effective monitoring systems and interpreting the data accurately to ensure reliable outcomes.