Understanding Pore Dynamics in Materials Science
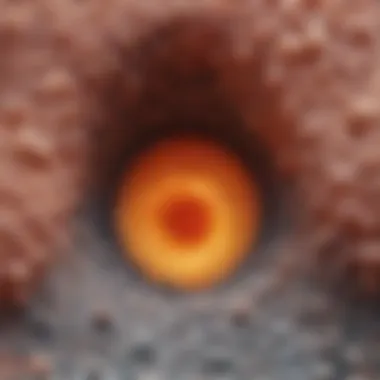
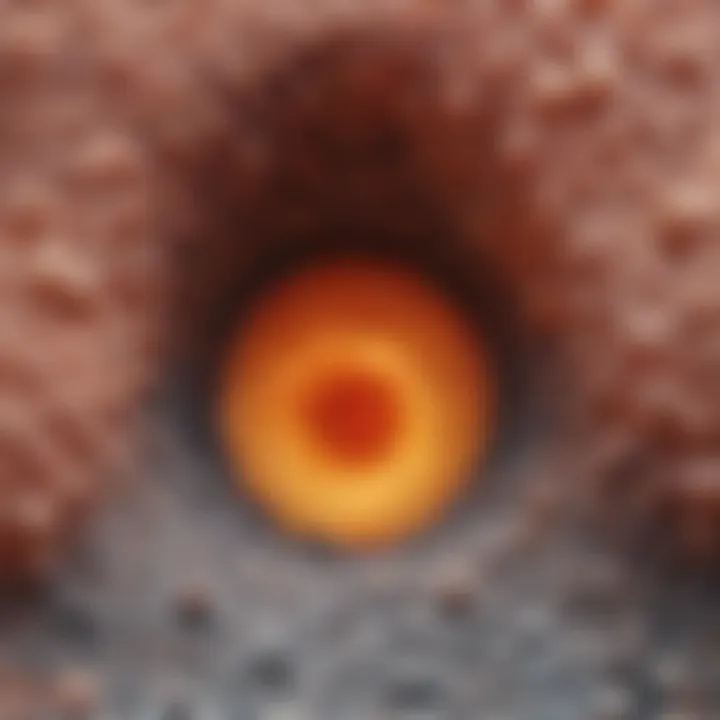
Intro
The study of pore formation in materials, be they biological membranes or synthetic substances, is critical to many scientific disciplines. Understanding how pores form, open, and interact with their environment can unlock new applications across medicine, materials science, and engineering. The processes surrounding pore dynamics are complex, involving numerous factors from molecular interactions to external influences.
Biological materials, such as cell membranes, serve essential roles in living organisms. They regulate the passage of ions and molecules, maintaining homeostasis within the cell. Synthetic materials, on the other hand, have wide-ranging applications, from filtration systems to drug delivery devices. The methods of controlling pore size and structure in both biological and synthetic contexts hold significant implications for advancements in technology and healthcare.
This article will unpack the critical elements of pore dynamics, methodologies to influence pore size, and the broad implications of these processes. With this exploration, we aim to bridge the gap between biology, chemistry, and materials science, providing readers with a comprehensive understanding of the mechanisms at play.
Research Highlights
Overview of Key Findings
Pore formation mechanisms vary widely between biological and synthetic materials. Key insights include:
- Biological Systems: Cell membranes employ proteins that can form transient pores, a process influenced by electrochemical gradients and signaling pathways.
- Synthetic Techniques: Materials like polycarbonate and hydrogels can have their pore sizes adjusted through techniques such as lithography and phase separation.
- Hybrid Approaches: Combining biological elements with synthetic frameworks is proving effective for developing advanced materials with tailored porosity.
Significance of the Research
The research into pore opening patterns is pivotal. The ability to control and manipulate pore sizes can lead to advances such as:
- Improved Drug Delivery Systems: Tailored pores can ensure that medications are released at desired rates.
- Enhanced Filtration Technologies: Pore size manipulation allows for selective filtering of substances in various industrial applications.
- Biocompatible Materials: Understanding how to create pores in synthetic materials that mimic biological ones can improve implants and grafts.
Understanding pore dynamics can lead to significant breakthroughs in both medicine and materials science, bridging crucial fields of study.
Original Research Articles
Summary of the Article
This article synthesizes numerous findings from recent studies on pore dynamics, highlighting both established and emerging methods. Each research piece contributes to a clearer understanding of the interactions involved in both biological and synthetic materials.
Author Contributions
The authors of the original studies discussed in this article have provided critical insights into the complexities of pore formation. Their collaborative efforts converge on a common goal: unlocking the potential of pore engineering in various applications, fostering innovation that can radically change current practices.
Prelude to Pore Dynamics
Pore dynamics is a fundamental aspect in the study of materials, both biological and synthetic. Understanding how pores form, open, and interact within various substances provides significant insights into the functionality and applications of these materials. In this section, we will explore the essential elements that govern pore dynamics.
The opening of pores is crucial for several reasons. First, it allows for the exchange of molecules, ions, and other entities, which is vital in biological processes such as nutrient uptake and waste elimination. In synthetic materials, pores are often engineered for specific tasks, such as filtration or catalysis. The mechanics of pore formation can lead to advancements in technology, from efficient drug delivery systems to improved water filtration processes.
Definition of Pores
Pores are small openings or voids within a material that facilitate the passage of substances. They can vary widely in size, shape, and orientation, depending on the material in question. In biological systems, pores are typically found in cell membranes, where they regulate the movement of ions and molecules. In synthetic materials, such as membranes used for filtration, the pores are engineered to achieve desired permeability and selectivity.
Importance of Pore Structure
The structure of pores is essential for determining the performance of a material in its intended application. The size and distribution of the pores influence how efficiently substances can penetrate or traverse through them.
- Impact on Functionality: The arrangement of pores can affect material properties such as strength, flexibility, and permeability. A balanced pore structure can enhance the efficiency of the material.
- Relevance to Applications: Different applications require specific pore characteristics. For example, a filter designed for water purification must have a distinct pore size to remove contaminants effectively.
- Material Interactions: The interaction between the material and the substances passing through the pores can lead to phase changes or alterations in the material structure itself. This can affect durability and longevity.
Pore Formation Mechanisms
The topic of pore formation mechanisms serves as a core element in understanding how pores develop and how they can be manipulated in both biological and synthetic materials. Grasping these mechanisms not only elucidates the natural processes at play but also enhances the application of engineered solutions in material sciences and biomedicine. This knowledge is essential for advancements in fields such as drug delivery, filtration, and sensing technologies.
Natural Pore Development
Natural pore development refers to the spontaneous formation of pores in various biological structures. This process is influenced by molecular and cellular dynamics, where specific environmental factors and biological signals contribute to pore growth. In biological membranes, such as those found in cells, pores are critical for processes like nutrient uptake and waste elimination.
In many organisms, membranes adapt their porosity in response to external stimuli. For example, in the case of E. coli, the formation of pores in the outer membrane allows for selective permeability, enabling the organism to regulate its internal environment based on nutrient availability. The mechanisms behind natural pore formation are intrinsic and often involve the action of proteins, Lipid composition, and external pressures.
- Factors influencing natural pore development include:
- Lipid Bilayer Composition: Variations in lipids can create regions of different fluidity, impacting pore formation.
- Protein Involvement: Membrane proteins play crucial roles in facilitating pore opening through conformational changes in response to signals.
- Environmental Stress: External factors such as temperature changes or ion concentrations can induce pore formation as a response mechanism.
The study of natural pore development offers insights into evolutionary adaptations and informs biotechnological applications. Researchers can harness these insights to design biomimetic materials that perform specific functions, mimicking the selective permeabilities found in nature.
Artificial Pore Engineering
Artificial pore engineering involves the deliberate creation or modification of pores within synthetic materials. This practice is integral to various technology sectors, particularly in filtration, drug delivery, and tissue engineering. By controlling pore size and distribution, scientists can fine-tune material properties to achieve desired functionalities.
Techniques for artificial pore creation vary greatly and can include:
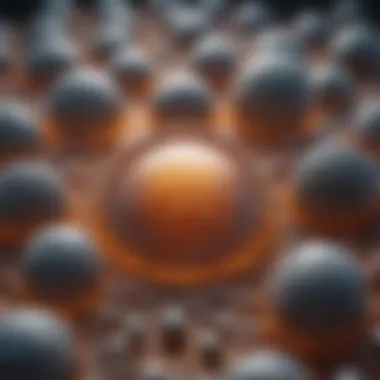
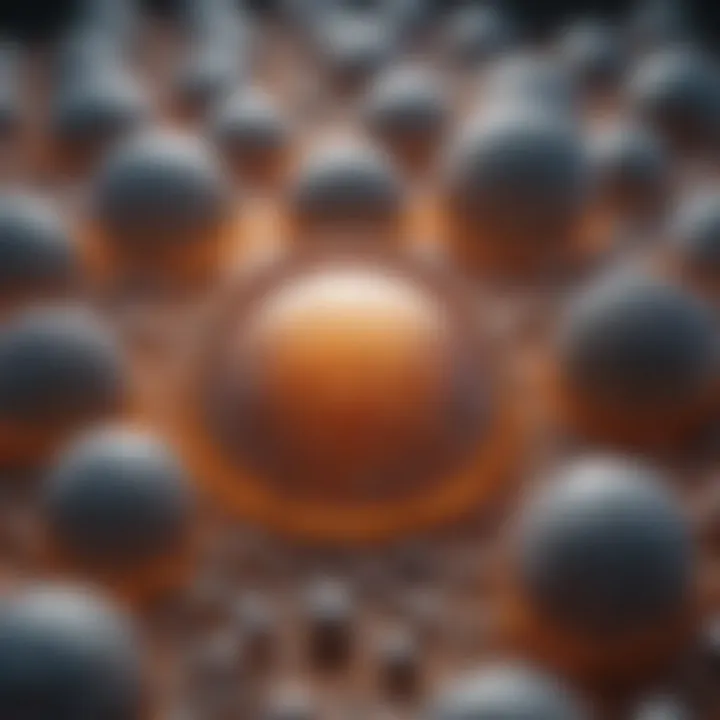
- Nanopatterning: Employing lithographic methods to create nanoscale patterns that dictate pore size.
- Polymer Membranes: Using phase separation or solvent casting to engineer pore characteristics during material synthesis.
- Laser Ablation: Applying focused laser beams to carve out precise pore structures in materials like films or membranes.
One significant advantage of artificial pore engineering is the ability to customize pore features to meet specific application requirements. For instance, in drug delivery systems, engineered pores can enhance the uptake and release profiles of therapeutic agents, ensuring that drugs are delivered more effectively in controlled manners.
Developments in artificial pore engineering have also opened pathways for creating more responsive materials that can adapt their pore structures under specific conditions, such as changes in pH or temperature. This adaptive capability presents exciting opportunities for dynamic systems in biomedical applications that require real-time responsiveness.
Through the integration of chemical, biological, and physical techniques, researchers can manipulate pore structures with high precision. This balance between natural processes and engineered solutions will likely continue to evolve, driving innovation across scientific and industrial landscapes.
Biological Perspectives on Pore Dynamics
Understanding the biological dimensions of pore dynamics sheds light on how organisms interact with their environments at a cellular level. Pores in biological membranes are crucial for maintaining homeostasis and facilitating essential processes, such as nutrient uptake and waste elimination. In this section, we will explore two critical aspects of pore dynamics: membrane permeability and the role of transport proteins. Each of these elements underscores the significance of pore formation in biological systems.
Membrane Permeability
Membrane permeability refers to the ability of substances to cross biological membranes through pores. This characteristic is fundamental for cellular function. The permeability of membranes affects how cells communicate, metabolize nutrients, and respond to environmental stimuli.
Key factors influencing membrane permeability include:
- Pore size and distribution: Larger or more numerous pores typically increase permeability, allowing a greater flow of molecules, while smaller or fewer pores can restrict movement.
- Lipid composition: The composition of lipids can change the fluidity and, thus, the permeability of membranes. Unsaturated fatty acids generally increase membrane fluidity, enhancing permeability.
- Protein presence: Proteins embedded within membranes can form channels that facilitate the transport of specific molecules. This adds complexity to the permeability profile of membranes.
Thus, understanding membrane permeability provides insights into how cells function and adapt. It also helps in developing drug delivery systems, where tailored pore structures can enhance the effectiveness of pharmaceutical compounds.
Role of Transport Proteins
Transport proteins play a crucial role in enabling the movement of ions and molecules across cellular membranes. These proteins can be categorized according to their function:
- Channel Proteins: These allow passive transport of substances down their concentration gradient. The presence of specific channels increases membrane permeability for certain ions or molecules, enhancing cellular functionality.
- Carrier Proteins: Unlike channel proteins, carrier proteins actively transport substances against a concentration gradient, often requiring energy. This function is vital for maintaining essential ion concentrations within cells.
Moreover, transport proteins can also undergo conformational changes during transport, which is critical for their function. This mechanism is a prime focus in biochemistry, as it relates to how cells precisely control their internal environments.
Understanding these transport mechanisms is vital for developing targeted therapies and optimizing conditions for various biotechnological applications.
In summary, the biological perspectives on pore dynamics reveal how essential these processes are for life. Pore dynamics ensure substance movement, which is fundamental for biochemical reactions, signaling, and overall cellular health. By investigating membrane permeability and transport proteins, we gain valuable insights into physiological processes that can inform scientific advancements.
Chemical Methods for Opening Pores
The manipulation of pore size and accessibility in various materials is critical for enhancing their functionality. Chemical methods for opening pores provide a strategic approach in this regard. By leveraging different chemical agents and reactions, researchers can effectively modify the structural characteristics of both biological and synthetic materials. This section will discuss solvent-assisted techniques and acidic and basic treatments, highlighting their significance, benefits, and considerations.
Solvent-Assisted Techniques
Solvent-assisted techniques play a pivotal role in the realm of pore modification. These methods involve the use of specific solvents to swell or dissolve certain components of the material, leading to the expansion of existing pores or the creation of new ones. For instance, in polymer membranes, solvents can be utilized to enhance the pore sizes temporarily, promoting increased permeability.
Key advantages of using solvent-assisted techniques include:
- Precision: The use of selective solvents allows for targeted modifications, minimizing the impact on the overall material structure.
- Mild conditions: These techniques can often be conducted at ambient temperatures, avoiding potential thermal degradation of the materials.
- Versatility: Various solvents can be employed depending on the material and desired pore characteristics, making this a flexible option for researchers.
However, considerations related to solvent compatibility and potential toxicity must be addressed. It is essential to select solvents that do not compromise the integrity of the material or pose health risks to the users.
Acidic and Basic Treatments
Acidic and basic treatments provide another effective means for opening pores within materials. These methods rely on the reaction of acids or bases with the matrix of the material. For example, treating a polymer with an acid can break down certain linkages, thereby increasing pore volume and surface area.
Some notable points regarding acidic and basic treatments are:
- Enhanced Surface Properties: These treatments can alter the surface chemistry of the material, affecting adsorption and interaction with other substances.
- Controlled Reactions: Adjusting the concentration and exposure time can lead to fine-tuning of pore sizes and structures.
- Broad Applicability: Acidic and basic treatments can be applied to a variety of substrates, including ceramics and metals.
Nevertheless, such treatments require careful handling due to the corrosive nature of many acids and bases. Proper safety protocols and material selection must be prioritized to avoid unwanted damage or hazards.
In summary, chemical methods for managing pores establish a foundation for effective engineering in both biological and synthetic materials, offering substantial potential for advancements in various scientific disciplines.
Physical Techniques in Pore Manipulation
The study of physical techniques in pore manipulation is pivotal for both scholarly inquiry and practical applications. Undoubtedly, understanding these methods provides insights into how we can tailor materials for specific uses. Physical techniques leverage fundamental principles of physics to alter the structure and functionality of materials. This section will focus on the core aspects of thermal methods and mechanical interventions, both of which play significant roles in modifying pore characteristics. These techniques are essential in various fields, including biotechnology, materials science, and nanotechnology.
Thermal Methods
Thermal methods for pore manipulation involve the application of heat to materials to induce changes in their physical structure. The process may entail modifying existing pore sizes or creating new pores altogether. These techniques can be beneficial for several reasons. First, heat can facilitate the expansion of materials, leading to increased pore size. This characteristic is particularly valuable when designing membranes used in filtration applications or in systems requiring enhanced permeability.
Moreover, thermal methods can create structural changes without introducing chemical contaminants. This feature is particularly important in biological contexts where maintaining material integrity is crucial. When applying thermal techniques, however, several considerations should be kept in mind:
- Temperature Control: Maintaining a controlled temperature is vital to prevent overheating, which can lead to material degradation.
- Material Selection: Not all materials respond favorably to heat. Understanding the thermal properties is necessary for effective results.
- Uniformity: Ensuring uniform heat distribution is essential to achieve consistent pore characteristics.
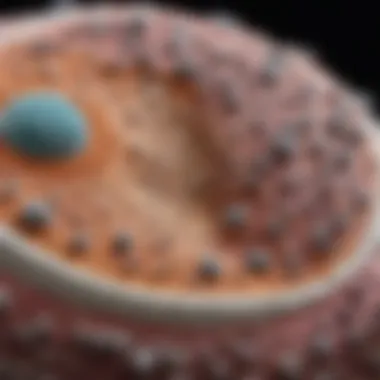
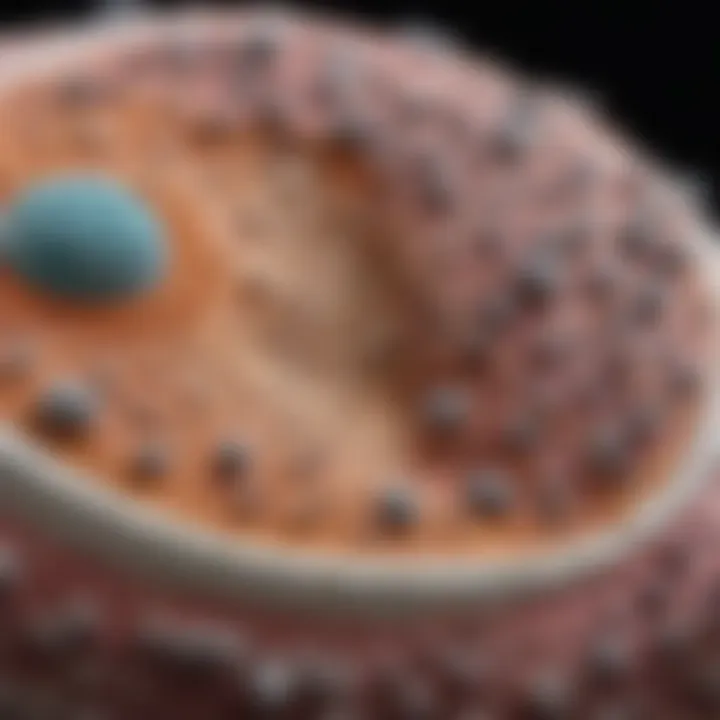
In recent studies, such as those pertaining to polymer membranes, researchers have shown significant promise in the utilization of thermal methods, yielding a greater understanding of their effectiveness.
Mechanical Interventions
Mechanical interventions refer to the techniques that involve physical forces to manipulate pore structures within materials. This category encompasses a variety of methods, including pressure application, stretching, and even ultrasonication. One crucial aspect of mechanical interventions is their potential for precision. These approaches allow researchers to tailor size and distribution of pores at a micro or nanoscale.
-Key Advantages of Mechanical Interventions-
- Customized Pore Sizes: The ability to adjust pore sizes based on specific needs can optimize performance in various applications like filtration and catalysis.
- Non-Chemical Process: Similar to thermal methods, these interventions often do not require chemicals, minimizing risks associated with contamination.
- Immediate Effects: Changes can be observed in real-time, facilitating rapid experimentation and adjustments based on experimental results.
However, implementing mechanical interventions also poses challenges. Quality control is critical, as uneven application of force can lead to unwanted variations in pore sizes. Furthermore, care must be taken to not compromise the integrity of the substrate material.
"Physical techniques provide powerful means to manipulate pore structures, with both thermal methods and mechanical interventions revealing unique advantages and challenges that merit careful consideration within their applications."
In summary, physical techniques in pore manipulation offer a vital understanding of how structural changes can enhance material functionality. Incorporating these methods allows for significant advancements in various applications, paving the way for innovative solutions in the fields of materials science and biotechnol.
Technological Applications
The study of pore dynamics has substantial implications for both biological and synthetic materials. Understanding the mechanisms of opening pores not only enriches our theoretical knowledge but also enhances practical applications across various fields. Technological applications serve as a bridge between fundamental research and everyday use, enabling advancements in diverse sectors such as medicine, environmental engineering, and materials science.
Opening pores can lead to significant improvements in efficiency and effectiveness, particularly in processes such as filtration, separation, and drug delivery. This section delves into the specific applications of pore dynamics in these areas and highlights the potential benefits and considerations necessitated by these technological advancements.
Filtration and Separation
Filtration and separation techniques leverage the concept of pore dynamics to enhance efficiency in many industrial processes. Pores in membranes facilitate selective permeability, allowing desired substances to pass through while retaining unwanted particles. This characteristic is crucial in applications such as water purification, air filtration, and even in the food industry.
Key Elements:
- Membrane Materials: Different materials are selected based on pore size and distribution. Polymeric membranes, ceramic membranes, and metallic membranes all offer unique advantages depending on the target application.
- Pore Size Control: Adjusting pore sizes is essential for optimizing performance. Nanofiltration and ultrafiltration are examples where specific pore sizes are crucial for achieving desired separation efficiency.
- Cost-Effectiveness: Advanced filtration systems using porous materials often reduce operational costs due to increased efficiency and reduced maintenance.
Considerations:
- Clogging and Fouling: One of the significant challenges is the fouling of membranes which can hinder performance. Techniques to minimize fouling are essential for long-term sustainability.
- Environmental Impact: Considering the environmental effects of materials used in membranes is vital. Sustainable choices can contribute positively toward ecological conservation.
"The importance of filtration and separation technologies cannot be overstated. They play a critical role in ensuring clean water, breathable air, and safe food products."
Drug Delivery Systems
Another profound technological application of opening pores is in drug delivery systems. Pore manipulation in various biomaterials creates pathways for precise drug dispensing, which enhances the bioavailability and effectiveness of pharmacological agents.
Key Elements:
- Controlled Release: Pore size and distribution in drug delivery matrices enable controlled release of therapeutics, allowing for steady plasma concentration over prolonged periods.
- Targeted Delivery: Engineering pores at a molecular level can allow for the targeting of specific cell types, minimizing side effects and increasing therapeutic efficacy.
- Biocompatibility: The materials used in drug delivery systems must be biocompatible to ensure safety and efficacy. Polymeric materials are often engineered for this purpose.
Considerations:
- Regulatory Hurdles: Drug delivery systems often face stringent regulatory scrutiny. A clear understanding of pore dynamics is crucial for meeting these requirements.
- Patient Compliance: Innovations in drug delivery that enhance patient compliance can significantly improve treatment outcomes. Systems that utilize pore dynamics must take usability into account.
The applications of pore dynamics in filtration and drug delivery represent just a glimpse of the broader impact that understanding pore mechanisms can have in technology. Through exploring these dimensions, researchers and industry professionals stand to gain insights that drive both innovation and improvement.
Implications of Opening Pores
Opening pores in biological and synthetic materials carries significant implications across various fields such as biology, materials science, and engineering. Understanding these implications is essential for optimizing the functionality and durability of materials while enhancing performance in applications such as drug delivery, filtration, and biological responses.
Impact on Biological Processes
The presence and size of pores in biological entities often determines how substances penetrate membranes and interact with cells. For instance, in the context of cellular membranes, pores facilitate essential processes such as nutrient uptake and waste elimination.
- Selective permeability: Pores allow certain molecules to traverse while restricting others, critical for maintaining homeostasis in cells. This selective permeability ensures that cells can control their internal environment, which is vital for metabolic processes.
- Cell signaling: Many signaling molecules enter cells through pores, influencing various physiological functions. The size and type of pores can affect the rate at which these signals are received and processed, impacting cellular responses significantly.
- Pathogens and immune response: Pores also play a role in allowing bacteria and viruses to enter cells, prompting immune system reactions. Research into pore dynamics can lead to better understanding and potential methods for preventing infections.
Material Longevity and Stability
From an engineering perspective, the way pores are structured in synthetic materials significantly impacts their longevity and structural stability. The durability of these materials depends on several factors that arise from the opening of pores.
- Degradation resistance: Materials with appropriately sized pores can resist degradation. When pores are too large or too small, they may lead to water infiltration or air entrapment, resultant in faster material decay.
- Mechanical properties: The arrangement of pores affects the mechanical integrity of a material. For example, in foams and membranes, the pore size and distribution can influence strength and elasticity. Appropriate design leads to materials that endure wear and tear over long periods.
- Chemical stability: Opening pores can also affect a material's resistance to various chemicals. For instance, if a synthetic polymer has numerous wide pores, it may absorb solvents that cause performance issues or degradation. Identifying ideal pore structures leads to enhanced chemical stability.
Understanding these implications is pivotal not just for improving existing products, but also for innovating new materials with tailored properties and performance metrics that meet the growing demands of various industries.
Challenges in Pore Management
The management of pores in both biological and synthetic materials presents distinct challenges that reflect the intricate balance between functionality and stability. Understanding these challenges is crucial as they bear implications for both immediate applications and long-term sustainability. Pores play a vital role in various processes, from cellular transport to material filtration. Without effective management strategies, achieving desired outcomes could be difficult. This section delves into the key challenges associated with pore management, focusing on the dimensions of controlling pore size and maintaining structural integrity.
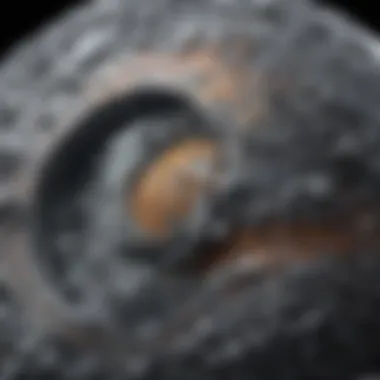
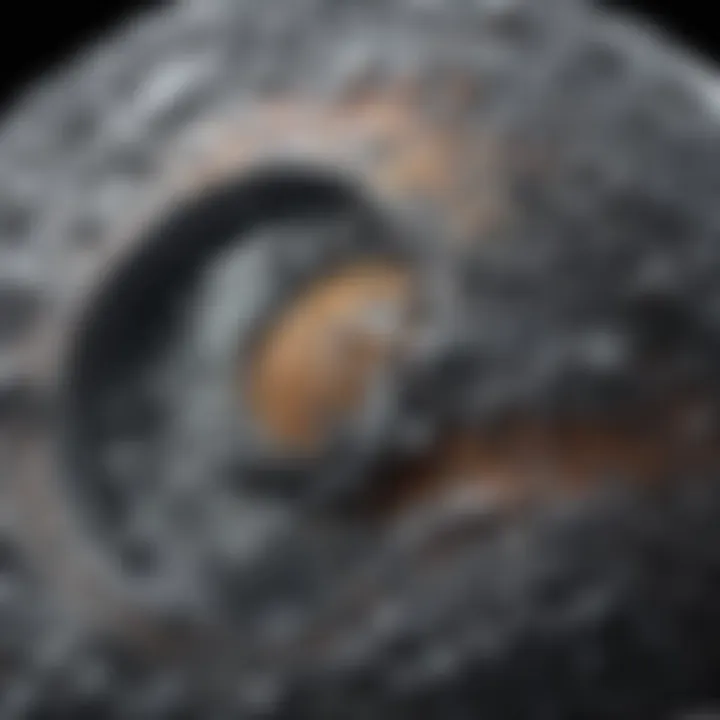
Controlling Pore Size
Controlling the size of pores is a critical factor in any application involving porous materials. The pore size directly influences permeability, adsorption capacity, and overall functionality. For example, in filtration applications, a precise pore size can determine whether harmful substances are retained or allowed to pass through.
- Defining Optimal Size: Different applications require specific pore sizes to function effectively. For instance, biomedical filters often need smaller pores compared to industrial membranes. This necessitates a comprehensive understanding of the operational requirements.
- Techniques for Size Adjustment: Several methodologies exist to manipulate pore size, including chemical etching, thermal treatments, and mechanical means. Each method has its own set of parameters and outcomes that must be carefully calibrated.
- Reproducibility Issues: Achieving consistent pore sizes across batches is often complicated. Variabilities in raw materials and processing conditions can contribute to this inconsistency.
- Impact on Properties: Altering pore size can sometimes lead to unintended changes in the physical and chemical properties of materials, which could affect performance. Evaluating these effects before finalizing pore modifications is essential.
Maintaining Structural Integrity
Maintaining the structural integrity of materials while manipulating pores is another significant challenge. Any alteration in pore structure can have profound implications on the stability and overall performance of the material. Fragility is a common concern, especially in biological membranes and synthetic materials.
- Interplay Between Structural Design and Pore Management: Designing materials with the ability to retain their integrity under varying conditions can be complex. Considerations include processing temperature, environmental conditions, and the physical stress materials may encounter.
- Degradation Risks: Pore creation processes may introduce flaws or weak points. For instance, excessive exposure to harsh chemicals can degrade membrane materials. Ensuring that the material remains robust involves careful selection of methods that balance pore creation and structural durability.
- Real-Time Monitoring: It's essential to develop means for monitoring the state of the material during processing. Techniques such as atomic force microscopy can be useful for observing changes in structure and ensuring that integrity is preserved.
"The balance between pore optimization and material integrity is not only a technical challenge but also a crucial determinant of material performance in practical applications."
In summary, managing pores in biological and synthetic materials entails navigating a landscape of challenges that, while complex, is surmountable with the right approach. The focus on controlling pore size and preserving structural integrity underscores the importance of strategic planning and execution in pore management.
Case Studies
Case studies play a pivotal role in understanding the mechanisms of opening pores in various materials. They offer practical examples where theoretical principles meet real-world applications. Furthermore, they provide insights into the effectiveness of different techniques, highlighting both successes and challenges faced during pore manipulation processes.
Pore Dynamics in Cellular Biology
Cellular biology is a prime field where pore dynamics significantly influence physiological processes. For instance, the opening and closing of pores in cellular membranes are critical for maintaining homeostasis. When studying these dynamics, researchers often examine ion channels and transport proteins. These proteins regulate the passage of ions and molecules in and out of cells, playing a vital role in cellular communication and metabolic processes.
One notable case study involves the use of electrophysiological techniques to measure the activity of ion channels in neurons. Researchers noted how pore opening allowed the influx of sodium ions, leading to action potentials necessary for nerve impulse transmission. Such studies reveal not just the mechanisms at play but also the broader implications for treatments in neurological disorders.
Additionally, the investigation of pores in pathogenic bacteria can provide insights into antibiotic resistance. By understanding how bacteria regulate pore size and accessibility, researchers can develop targeted strategies to combat infections. These case studies serve as vital benchmarks for ongoing research and innovation in both pharmacology and cellular bioengineering.
Applications in Membrane Technology
Membrane technology encompasses a wide array of applications, from water purification to gas separation and biomedical uses. Case studies in this area illustrate how engineered pores can optimize performance in various systems. For example, researchers have investigated polymer membranes with controlled pore sizes for desalination processes. These membranes utilize selective permeability to enable freshwater production from saline sources.
Furthermore, another case study highlights advancements in membrane bioreactors, which blend biological processes with membrane filtration. This approach enhances treatment efficiency for wastewater, demonstrating how tailored pore structures can significantly improve material function.
- Benefits of case studies in membrane technology might include:
- Practical insights into pore behavior under varying conditions.
- Validation of theoretical models through empirical evidence.
- Identification of potential limitations in current technologies, guiding future research.
Future Directions in Pore Research
The exploration of pore dynamics is a rapidly evolving field. It offers critical insights not only into biological functions but also for industrial applications. As our understanding of materials and their interactions grows, so does the importance of focusing on future directions in pore research. This area of study holds enormous potential for innovation and improved applications in diverse sectors, from medicine to environmental science.
Future research can lead to the development of new materials and methods for controlling pore structures, enabling us to refine processes in various scientific domains. Potential benefits include enhanced efficiency in filtration systems, increased effectiveness in drug delivery technologies, and improved energy storage capabilities. Moreover, addressing the challenges of pore management could pave the way for sustainable practices across multiple industries.
Innovative Approaches to Pore Engineering
Contemporary research is unveiling innovative strategies for pore engineering that go beyond traditional methods. For instance, the use of 3D printing allows for precise control over pore architecture. This technique can tailor materials for specific functions, such as adaptable filtration mechanisms or responsive drug release systems.
Additionally, advances in nanotechnology provide opportunities to manipulate pores at the molecular level. By engineering materials with specific pore sizes and distributions, researchers can enhance selectivity and transport efficiency. Such innovations are essential to keeping pace with the growing demands for specificity in applications like selective adsorption and targeted therapies.
Innovative approaches also embrace soft materials, which can change size or shape in response to environmental changes. These adaptable materials have vast potential in biomedical applications, especially for dynamic drug delivery.
Sustainability in Pore-Related Applications
Sustainability is a critical consideration in pore research and applications. As industries increasingly focus on minimizing their environmental impact, developing sustainable materials and processes becomes paramount. Pore-related research can contribute significantly to these efforts by optimizing the use of resources and reducing waste.
For instance, the design of biodegradable membranes with controlled porosity offers a way to improve the sustainability of filtering and separation processes. By utilizing renewable materials and minimizing the ecological footprint, these innovations align well with the current sustainability trends.
Moreover, the consideration of lifecycle impacts of materials will guide future developments. Assessing the environmental implications of pore dynamics—from production to disposal—ensures the longevity and safety of materials used in various applications.
Culmination
Understanding the mechanisms of opening pores in both biological and synthetic materials is essential for various scientific and industrial applications. This article provides a comprehensive overview of the key aspects that contribute to pore dynamics. By elucidating the principles of pore formation and manipulation, we gain insights that can lead to advancements in multiple fields.
Summary of Key Insights
In summary, the exploration of pore dynamics covers several vital themes:
- Definitions and Importance: Pores are not mere structures; they determine the functionality and effectiveness of materials in biological systems and technological applications.
- Formation Mechanisms: Natural and artificial processes govern the development of pores. Understanding these can enhance material design strategies.
- Methods of Manipulation: Techniques that include chemical and physical approaches allow for tailored pore characteristics, which is critical in drug delivery and filtration applications.
- Challenges: Controlling pore size and maintaining structural integrity present significant challenges that researchers continue to address.
- Future Directions: Innovations in pore engineering and sustainable applications are paving the way for future research, underlining the importance of interdisciplinary approaches in this domain.
"The exploration and manipulation of pores span a diverse range of disciplines, reflecting their fundamental role in both nature and technology."
The Way Forward in Pore Studies
Looking ahead, the way forward in pore studies entails several considerations:
- Innovative Research Approaches: Continued research into novel methods for pore creation and modification will drive progress in material sciences. This includes exploring nanotechnology and biotechnology as cutting-edge approaches.
- Collaboration Across Disciplines: A multidisciplinary approach connecting biology, chemistry, and materials science will deliver holistic solutions to complex problems. Leveraging expertise from these fields can foster innovative applications.
- Sustainability: As industries focus on environmental impact, developing eco-friendly methods to engineer pores will gain importance. Research in sustainable materials that incorporate desired pore properties while minimizing ecological footprints will be a priority.
- Application-Specific Studies: Tailored studies that focus on specific applications, such as biomedical engineering or environmental remediation, will provide more practical insights into pore manipulation.
- Technological Integration: The integration of advanced technologies, like artificial intelligence and machine learning, might revolutionize how effective pore management and analysis are conducted.